1 Introduction
Pharmaceutical products used in the prevention, treatment and diagnosis of disease contain a wide variety of ingredients, often in quite complex physicochemical states. Such products must not only meet current good pharmaceutical manufacturing practice (GPMP) requirements for quality, safety and efficacy, but also must be stable and sufficiently attractive to be acceptable to patients. Products made in the pharmaceutical industry today must meet high microbiological specifications; i.e. if not sterile, they are expected to have no more than a minimal microbial population at the time of product release.
Nevertheless, from time to time a few rogue products with an unacceptable level and type of contamination will occasionally escape the quality assurance net. The consequences of such contamination may be serious and far-reaching on several accounts, particularly if contaminants have had the opportunity to multiply to high levels. First, the product may be spoiled, rendering it unfit for use through chemical and physicochemical deterioration of the formulation. Spoilage and subsequent wastage of individual batches usually result in major financial problems for the manufacturer through direct loss of faulty product. Secondly, the threat of litigation and the unwanted, damaging publicity of recalls may have serious economic implications for the manufacturer. Thirdly, inadvertent use of contaminated products may present a potential health hazard to patients, perhaps resulting in outbreaks of medicament-related infections, and ironically therefore contributing to the spread of disease. Most commonly, heavy contamination of product with opportunist pathogens, such as Pseudomonas spp., has resulted in the spread of nosocomial (hospital-acquired) infections in compromised patients; less frequently, low levels of contamination with pathogenic organisms, such as Salmonella, have attracted considerable attention, as have products contaminated with toxic microbial metabolites, such as mycotoxins in herbal medicines. The consequences of microbial contamination in pharmaceutical products are discussed in more detail below.
2 Spoilage—chemical and physicochemical deterioration of pharmaceuticals
Microorganisms form a major part of the natural recycling processes for biological matter in the environment. As such, they possess a wide variety of degradative capabilities, which they are able to exert under relatively mild physicochemical conditions. Mixed natural communities are often far more effective cooperative biodeteriogens than the individual species alone, and sequences of attack of complex substrates occur where initial attack by one group of microorganisms renders them susceptible to further deterioration by secondary, and subsequent, microorganisms. Under suitable environmental selection pressures, novel degradative pathways may emerge with the capability to attack newly introduced synthetic chemicals (xenobiotics). However, the rates of degradation of materials released into the environment can vary greatly, from half-lives of hours (phenol) to months ( ‘hard’ detergents) to years (halogenated pesticides).
The overall rate of deterioration of a chemical depends on its molecular structure; the physicochemical properties of a particular environment; the type and quantity of microbes present; and whether the metabolites produced can serve as sources of usable energy and precursors for the biosynthesis of cellular components, and hence the creation of more microorganisms.
Pharmaceutical formulations may be considered as specialized microenvironments and their susceptibility to microbial attack can be assessed using conventional ecological criteria. Some naturally occurring ingredients are particularly sensitive to attack, and a number of synthetic components, such as modern surfactants, have been deliberately constructed to be readily degraded after disposal into the environment. Crude vegetable and animal drug extracts often contain a wide assortment of microbial nutrients besides the therapeutic agents. This, combined with frequently conducive and unstable physicochemical characteristics, leaves many formulations with a high potential for microbial attack unless steps are taken to minimize it.
2.1 Pharmaceutical ingredients susceptible to microbial attack
• Therapeutic agents. Through spoilage, active drug constituents may be metabolized to less potent or chemically inactive forms. Under laboratory conditions, it has been shown that a variety of microorganisms can metabolize a wide assortment of drugs, resulting in loss of activity. Materials as diverse as alkaloids (morphine, strychnine, atropine), analgesics (aspirin, paracetamol), thalidomide (still used in the treatment of some forms of cancer), barbiturates, steroid esters and mandelic acid can be metabolized and serve as substrates for growth. Indeed, the use of microorganisms to carry out subtle transformations on steroid molecules forms the basis of the commercial production of potent therapeutic steroidal agents (see Chapter 26). In practice, reports of drug destruction in medicines are less frequent. There have, however, been some notable exceptions: the metabolism of atropine in eye drops by contaminating fungi; inactivation of penicillin injections by β-lactamaseproducing bacteria (see Chapters 11 and 13); steroid metabolism in damp tablets and creams by fungi; microbial hydrolysis of aspirin in suspension by esterase-producing bacteria; and chloramphenicol acetylase-producing contaminant.
• Surface-active agents. Anionic surfactants, such as the alkali metal and amine soaps of fatty acids, are generally stable because of the slightly alkaline pH of the formulations, although readily degraded once diluted into sewage. Alkyl and alkylbenzene sulphonates and sulphate esters are metabolized by ω-oxidation of their terminal methyl groups followed by sequential β-oxidation of the alkyl chains and fission of the aromatic rings. The presence of chain branching involves additional α-oxidative processes. Generally, ease of degradation decreases with increasing chain length and complexity of branching of the alkyl chain.
• Non-ionic surfactants, such as alkylpolyoxyethylene alcohol emulsifiers, are readily metabolized by a wide variety of microorganisms. Increasing chain lengths and branching again decrease ease of attack. Alkylphenol polyoxyethylene alcohols are similarly attacked, but are significantly more resistant. Lipolytic cleavage of the fatty acids from sorbitan esters, polysorbates and sucrose esters is often followed by degradation of the cyclic nuclei, producing numerous small molecules readily utilizable for microbial growth. Ampholytic surfactants, based on phosphatides, betaines and alkylaminosubstituted amino acids, are an increasingly important group of surfactants and are generally reported to be reasonably biodegradable. The cationic surfactants used as antiseptics and preservatives in pharmaceutical applications are usually only slowly degraded at high dilution in sewage. Pseudomonads have been found growing readily in quaternary ammonium antiseptic solutions, largely at the expense of other ingredients such as buffering materials, although some metabolism of the surfactant has also been observed.
• Organic polymers. Many of the thickening and suspending agents used in pharmaceutical formulations are subject to microbial depolymerization by specific classes of extracellular enzymes, yielding nutritive fragments and monomers. Examples of such enzymes, with their substrates in parentheses, are: amylases (starches), pectinases (pectins), cellulases (carboxymethylcelluloses, but not alkylcelluloses), uronidases (polyuronides such as in tragacanth and acacia), dextranases (dextrans) and proteases (proteins). Agar (a complex polysaccharide) is an example of a relatively inert polymer and, as such, is used as a support for solidifying microbiological culture media. The lower molecular weight polyethylene glycols are readily degraded by sequential oxidation of the hydrocarbon chain, but the larger congeners are rather more recalcitrant. Synthetic packaging polymers such as nylon, polystyrene and polyester are extremely resistant to attack, although cellophane (modified cellulose) is susceptible under some humid conditions.
• Humectants. Low molecular weight materials such as glycerol and sorbitol are included in some products to reduce water loss and may be readily metabolized unless present in high concentrations (see section 2.3.3).
• Fats and oils. These hydrophobic materials are usually attacked extensively when dispersed in aqueous formulations such as oil-in-water emulsions, aided by the high solubility of oxygen in many oils. Fungal attack has been reported in condensed moisture films on the surface of oils in bulk, or where water droplets have contaminated the bulk oil phase. Lipolytic rupture of triglycerides liberates glycerol and fatty acids, the latter often then undergoing β-oxidation of the alkyl chains and the production of odiferous ketones. Although the microbial metabolism of pharmaceutical hydrocarbon oils is rarely reported, this is a problem in engineering and fuel technology when water droplets have accumulated in oil storage tanks and subsequent fungal colonization has catalysed serious corrosion.
• Sweetening, flavouring and colouring agents. Many of the sugars and other sweetening agents used in pharmacy are ready substrates for microbial growth. However, some are used in very high concentrations to reduce water activity in aqueous products and inhibit microbial attack (see section 2.3.3). At one time, a variety of colouring agents (such as tartrazine and amaranth) and flavouring agents (such as peppermint water) were kept as stock solutions for extemporaneous dispensing purposes, but they frequently supported the growth of Pseudomonas spp., including Ps. aeruginosa. Such stock solutions should now be preserved, or freshly made as required by dilution of alcoholic solutions which are much less susceptible to microbial attack.
• Preservatives and disinfectants. Many preservatives and disinfectants can be metabolized by a wide variety of Gram-negative bacteria, although most commonly at concentrations below their effective ‘use’ levels. Growth of pseudomonads in stock solutions of quaternary ammonium antiseptics and chlorhexidine has resulted in infection of patients. Pseudomonas spp. have metabolized 4-hydroxybenzoate (parabens) ester preservatives contained in eyedrops and caused serious eye infections, and have also metabolized the preservatives in oral suspensions and solutions. In selecting suitable preservatives for formulation, a detailed knowledge of the properties of such agents, their susceptibility to contamination and limitations clearly provides invaluable information.
2.2 Observable effects of microbial attack on pharmaceutical products
Microbial contaminants usually need to attack formulation ingredients and create substrates necessary for biosynthesis and energy production before they can replicate to levels where obvious spoilage becomes apparent. Thus, for example, 106 microbes will have an overall degradative effect around 106 times faster than one cell. However, growth and attack may well be localized in surface moisture films or very unevenly distributed within the bulk of viscous formulations such as creams. Early indications of spoilage are often organoleptic, with the release of unpleasant smelling and tasting metabolites such as ‘sour’ fatty acids, ‘fishy’ amines, ‘bad eggs ’, bitter, ‘earthy ’ or sickly tastes and smells. Products may become unappealingly discoloured by microbial pigments of various shades. Thickening and suspending agents such as tragacanth, acacia or carboxymethylcellulose can be depolymerized, resulting in loss of viscosity and sedimentation of suspended ingredients. Alternatively, microbial polymerization of sugars and surfactant molecules can produce slimy, viscous masses in syrups, shampoos and creams, and fungal growth in creams has produced ‘gritty’ textures. Changes in product pH can occur depending on whether acidic or basic metabolites are released, and become so modified as to permit secondary attack by microbes previously inhibited by the initial product pH. Gaseous metabolites may be seen as trapped bubbles within viscous formulations.
When a complex formulation such as an oil-in-water emulsion is attacked, a gross and progressive spoilage sequence may be observed. Metabolism of surfactants will reduce stability and accelerate ‘creaming’ of the oil globules. Lipolytic release of fatty acids from oils will lower pH and encourage coalescence of oil globules and ‘cracking’ of the emulsion. Fatty acids and their ketonic oxidation products will provide a sour taste and unpleasant smell, while bubbles of gaseous metabolites may be visible, trapped in the product, and pigments may discol-our it (see Figure 17.1).
Figure 17.1 Section (× 1.5) through an inadequately preserved olive oil, oil-in-water, emulsion in an advanced state of microbial spoilage showing: A, discoloured, oil-depleted, aqueous phase; B, oil globule-rich creamed layer; C, coalesced oil layer from ‘cracked’ emulsion; D, fungal mycelial growth on surface. Also present are a foul taste and evil smell.
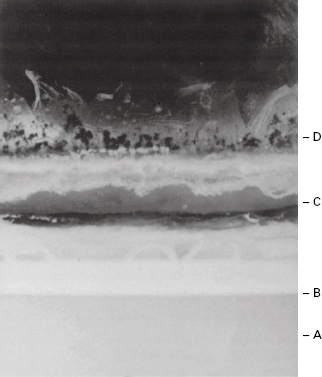
2.3 Factors affecting microbial spoilage of pharmaceutical products
By understanding the influence of environmental parameters on microorganisms, it may be possible to manipulate formulations to create conditions which are as unfavourable as possible for growth and spoilage, within the limitations of patient acceptability and therapeutic efficacy. Furthermore, the overall characteristics of a particular formulation will indicate its susceptibility to attack by various classes of microorganisms.
2.3.1 Types and size of contaminant inoculum
Successful formulation of products against microbial attack involves an element of prediction. An understanding of where and how the product is to be used and the challenges it must face during its life will enable the formulator to build in as much protection as possible against microbial attack. When failures inevitably occur from time to time, knowledge of the microbial ecology and careful identification of contaminants can be most useful in tracking down the defective steps in the design or production process.
Low levels of contaminants may not cause appreciable spoilage, particularly if they are unable to replicate in a product; however, an unexpected surge in the contaminant bioburden may present an unacceptable challenge to the designed formulation. This could arise if, for example, raw materials were unusually contaminated; there was a lapse in the plant-cleaning protocol; a biofilm detached itself from within supplying pipework; or the product had been grossly misused during administration. Inoculum size alone is not always a reliable indicator of likely spoilage potential. Low levels of aggressive pseudomonads in a weakly preserved solution may pose a greater risk than tablets containing fairly high numbers of fungal and bacterial spores.
When an aggressive microorganism contaminates a medicine, there may be an appreciable lag period before significant spoilage begins, the duration of which decreases disproportionately with increasing contaminant loading. As there is usually a considerable delay between manufacture and administration of factorymade medicines, growth and attack could ensue during this period unless additional steps are taken to prevent it. On the other hand, for extemporaneously dispensed formulations some control can be provided by specifying short shelf-lives, for example 2 weeks.
The isolation of a particular microorganism from a markedly spoiled product does not necessarily mean that it was the initiator of the attack. It could be a secondary opportunist contaminant which had overgrown the primary spoilage organism once the physicochemical properties had been favourably modified by the primary spoiler.
2.3.2 Nutritional factors
The simple nutritional requirements and metabolic adaptability of many common spoilage microorganisms enable them to utilize many formulation components as substrates for biosynthesis and growth. The use of crude vegetable or animal products in a formulation provides an additionally nutritious environment. Even demineralized water prepared by good ion-exchange methods will normally contain sufficient nutrients to allow significant growth of many waterborne Gram-negative bacteria such as Pseudomonas spp. When such contaminants fail to survive, it is unlikely to be the result of nutrient limitation in the product but due to other, non-supportive, physicochemical or toxic properties.
Acute pathogens require specific growth factors normally associated with the tissues they infect but which are often absent in pharmaceutical formulations. They are thus unlikely to multiply in them, although they may remain viable and infective for an appreciable time in some dry products where the conditions are suitably protective.
2.3.3 Moisture content: water activity (Aw)
Microorganisms require readily accessible water in appreciable quantities for growth to occur. By measuring a product’s water activity, Aw, it is possible to obtain an estimate of the proportion of uncomplexed water that is available in the formulation to support microbial growth, using the formula Aw = vapour pressure of formulation/vapour pressure of water under similar conditions.
The greater the solute concentration, the lower is the water activity. With the exception of halophilic bacteria, most microorganisms grow best in dilute solutions (high Aw) and, as solute concentration rises (lowering Aw), growth rates decline until a minimal growth-inhibitory Aw, is reached. Limiting Aw values are of the order of 0.95 for Gram-negative rods; 0.9 for staphylococci, micrococci and lactobacilli; and 0.88 for most yeasts. Syrupfermenting osmotolerant yeasts have spoiled products with Aw levels as low as 0.73, while some filamentous fungi such as Aspergillus glaucus can grow at 0.61.
The Aw of aqueous formulations can be lowered to increase resistance to microbial attack by the addition of high concentrations of sugars or polyethylene glycols. However, even Syrup BP (67% sucrose; Aw = 0.86) has occasionally failed to inhibit osmotolerant yeasts and additional preservation may be necessary. With a continuing trend towards the elimination of sucrose from medicines, alternative solutes which are not thought to encourage dental caries such as sorbitol and fructose have been investigated. Aw can also be reduced by drying, although the dry, often hygroscopic medicines (tablets, capsules, powders, vitreous ‘glasses’) will require suitable packaging to prevent resorption of water and consequent microbial growth (Figure 17.2).
Figure 17.2 Fungal growth on a tablet which has become damp (raised Aw) during storage under humid conditions. Note the sparseness of mycelium, and conidiophores. The contaminant is thought to be a Penicillium sp.
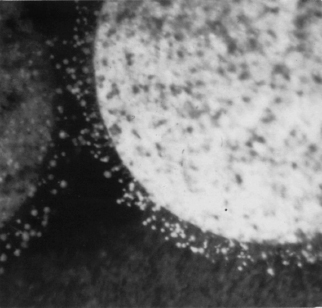
Tablet film coatings are now available which greatly reduce water vapour uptake during storage while allowing ready dissolution in bulk water. These might contribute to increased microbial stability during storage in particularly humid climates, although suitable foil strip packing may be more effective, albeit more expensive.
Condensed water films can accumulate on the surface of otherwise ‘dry’ products such as tablets or bulk oils following storage in damp atmospheres with fluctuating temperatures, resulting in sufficiently high localized Aw to initiate fungal growth. Condensation similarly formed on the surface of viscous products such as syrups and creams, or exuded by syneresis from hydrogels, may well permit surface yeast and fungal spoilage.
2.3.4 Redox potential
The ability of microbes to grow in an environment is influenced by their oxidation–reduction balance (redox potential), as they will require compatible terminal electron acceptors to permit their respiratory pathways to function. The redox potential even in fairly viscous emulsions may be quite high because of the appreciable solubility of oxygen in most fats and oils.
2.3.5 Storage temperature
Spoilage of pharmaceuticals could occur potentially over the range of about −20°C to 60°C, although it is much less likely at the extremes. The particular storage temperature may selectively determine the types of microorganisms involved in spoilage. A deep freeze at − 20 °C or lower is used for long-term storage of some pharmaceutical raw materials and short-term storage of dispensed total parenteral nutrition (TPN) feeds prepared in hospitals. Reconstituted syrups and multidose eye drop packs are sometimes dispensed with the instruction to ‘store in a cool place’ such as a domestic fridge (2–8 °C), partly to reduce the risk of growth of contaminants inadvertently introduced during use. Conversely, Water for Injections (EP) should be held at 80 °C or above after distillation and before packing and sterilization to prevent possible regrowth of Gram-negative bacteria and the release of endotoxins.
2.3.6 pH
Extremes of pH prevent microbial attack. Around neutrality bacterial spoilage is more likely, with reports of pseudomonads and related Gram-negative bacteria growing in antacid mixtures, flavoured mouthwashes and distilled or demineralized water. Above pH 8 (e.g. with soap-based emulsions) spoilage is rare. In products with low pH levels (e.g. fruit-juice-flavoured syrups with a pH 3–4), mould or yeast attack is more likely. Yeasts can metabolize organic acids and raise the pH to levels where secondary bacterial growth can occur. Although the use of low pH adjustment to preserve foodstuffs is well established (e.g. pickling, coleslaw, yoghurt), it is not practicable to make deliberate use of this for medicines.
2.3.7 Packaging design
Packaging can have a major influence on microbial stability of some formulations in controlling the entry of contaminants during both storage and use. Considerable thought has gone into the design of containers to prevent the ingress of contaminants into medicines for parenteral administration, because of the high risks of infection by this route. Self-sealing rubber wads must be used to prevent microbial entry into multidose injection containers (Chapter 22) following withdrawals with a hypodermic needle. Wide-mouthed cream jars have now been replaced by narrow nozzles and flexible screw-capped tubes, thereby removing the likelihood of operatorintroduced contamination during use of the product. Similarly, hand creams, previously supplied in glass jars, are now packed in closed, disposable dispensers. Where medicines rely on their low Aw to prevent spoilage, packaging such as strip foils must be of water-vapour-proof materials with fully efficient seals. Cardboard outer packaging and labels themselves can become substrates for microbial attack under humid conditions, and preservatives are often included to reduce the risk of damage.
2.3.8 Protection of microorganisms within pharmaceutical products
The survival of microorganisms in particular environments is sometimes influenced by the presence of relatively inert materials. Thus, microbes can be more resistant to heat or desiccation in the presence of polymers such as starch, acacia or gelatin. Adsorption on to naturally occurring particulate material may aid establishment and survival in some environments. There is a belief, but limited hard evidence, that the presence of suspended particles such as kaolin, magnesium trisilicate or aluminium hydroxide gel may influence contaminant longevity in those products containing them, and that the presence of some surfactants, suspending agents and proteins can increase the resistance of microorganisms to preservatives, over and above their direct inactivating effect on the preservative itself.
Nowadays, it is well recognized that the inadvertent use of a contaminated pharmaceutical product may also present a potential health hazard to the patient. Although isolated outbreaks of medicament-related infections had been reported since the early part of the 20th century, it was only in the 1960s and 1970s that the significance of this contamination to the patient was more fully understood.
Inevitably, the infrequent isolation of true pathogens, such as Salmonella spp. and the reporting of associated infections following the use of products contaminated with these organisms (tablets with pancreatin and thyroid extract), attracted considerable attention. More often, the isolation of common saprophytic and non-fastidious opportunist contaminants with limited pathogenicity to healthy individuals has presented a significant challenge to compromised patients.
Gram-negative contaminants, particularly Pseudomonas spp., which have simple nutritional requirements and can multiply to significant levels in aqueous products, have been held responsible for numerous outbreaks of infection. For example, while the intact cornea is quite resistant to infection, it offers little resistance to pseudomonads and related bacteria when scratched, or damaged by irritant chemicals; loss of sight has frequently occurred following the use of poorly designed ophthalmic solutions which had become contaminated by Ps. aeruginosa and even supported its active growth. Pseudomonads contaminating ‘antiseptic ’ solutions have infected the skin of badly burnt patients, resulting in the failure of skin grafts and subsequent death from Gram-negative septicaemia. Infections of eczematous skin and respiratory infections in neonates have been traced to ointments and creams contaminated with Gram-negative bacteria. Oral mixtures and antacid suspensions can support the growth of Gram-negative bacteria and serious consequences have resulted following their inadvertent administration to patients who were immuno-compromised as a result of antineoplastic chemotherapy. Growth of Gram-negative bacteria in bladder washout solutions has been held responsible for painful infections. In more recent times, Pseudomonas contamination of TPN fluids during their aseptic compounding in the hospital pharmacy caused the death of several children in the same hospital.
Fatal viral infections resulting from the use of contaminated human tissue or fluids as components of medicines are well recorded. Examples of this include HIV infection of haemophiliacs by contaminated and inadequately treated factor VIII products made from pooled human blood, and Creutzfeldt–Jakob disease (CJD) from injections of human growth hormone derived from human pituitary glands, some of which were infected.
Pharmaceutical products of widely differing forms are known to be susceptible to contamination with a variety of microorganisms, ranging from true pathogens to a motley collection of opportunist pathogens (see Table 17.1). Disinfectants, antiseptics, powders, tablets and other products providing an inhospitable environment to invading contaminants are known to be at risk, as well as products with more nutritious components, such as creams and lotions with carbohydrates, amino acids, vitamins and often appreciable quantities of water.
The outcome of using a contaminated product may vary from patient to patient, depending on the type and degree of contamination and how the product is to be used. Undoubtedly, the most serious effects have been seen with contaminated injected products where generalized bacteraemic shock and in some cases death of patients have been reported. More likely, a wound or sore in broken skin may become locally infected or colonized by the contaminant; this may in turn result in extended hospital bed occupancy, with ensuing economic consequences. It must be stressed, however, that the majority of cases of medicament-related infections are probably not recognized or reported as such. Recognition of these infections presents its own problems. It is a fortunate hospital physician who can, at an early stage, recognize contamination shown as a cluster of infections of rapid onset, such as that following the use of a contaminated intravenous fluid in a hospital ward. The chances of a general practitioner recognizing a medicament-related infection of insidious onset, perhaps spread over several months, in a diverse group of patients in the community, are much more remote. Once recognized, of course, there is a moral obligation to withdraw the offending product; subsequent investigations of the incident therefore become retrospective.
3.1 Microbial toxins
Gram-negative bacteria contain lipopolysaccharides (endotoxins) in their outer cell membranes (Chapter 22); these can remain in an active condition in products even after cell death and some can survive moist heat sterilization. Although inactive by the oral route, endotoxins can induce a number of physiological effects if they enter the bloodstream via contaminated infusion fluids, even in nanogram quantities, or via diffusion across membranes from contaminated haemodialysis solutions. Such effects may include fever, activation of the cytokine system, endothelial cell damage, all leading to septic and often fatal febrile shock.
The acute bacterial toxins associated with food poisoning episodes are not commonly reported in pharmaceutical products, although aflatoxin-producing aspergilli have been detected in some vegetable and herbal ingredients. However, many of the metabolites of microbial deterioration have quite unpleasant tastes and smell even at low levels, and would deter most patients from using such a medicine.
4 Sources and control of contamination
4.1 In manufacture
Regardless of whether manufacture takes place in industry or on a smaller scale in the hospital pharmacy, the microbiological quality of the finished product will be determined by the formulation components used, the environment in which they are manufactured and the manufacturing process itself. As discussed in Chapter 23, quality must be built into the product at all stages of the process and not simply inspected at the end of manufacture:
• Raw materials, particularly water and those of natural origin, must be of a high microbiological standard.
• All processing equipment should be subject to planned preventive maintenance and should be properly cleaned after use to prevent cross-contamination between batches.
• Cleaning equipment should be appropriate for the task in hand and should be thoroughly cleaned and properly maintained.
• Manufacture should take place in suitable premises, supplied with filtered air, for which the environmental requirements vary according to the type of product being made.
• Staff involved in manufacture should not only have good health but also a sound knowledge of the importance of personal and production hygiene.
• The end-product requires suitable packaging which will protect it from contamination during its shelf-life and is itself free from contamination.
4.1.1 Hospital manufacture
Manufacture in hospital premises raises certain additional problems with regard to contamination control.
4.1.1.1 Water
Mains water in hospitals is frequently stored in large roof tanks, some of which may be relatively inaccessible and poorly maintained. Water for pharmaceutical manufacture requires some further treatment, usually by distillation, reverse osmosis or deionization or a combination of these, depending on the intended use of water. Such processes need careful monitoring, as does the microbiological quality of the water after treatment. Storage of water requires particular care, as some Gram-negative opportunist pathogens can survive on traces of organic matter present in treated water and will readily multiply to high numbers at room temperature. Water should therefore be stored at a temperature in excess of 80 °C and circulated in the distribution system at a flow rate of 1–2 m/s to prevent the build-up of bacterial biofilms in the piping.
4.1.1.2 Environment
The microbial flora of the hospital pharmacy environment is a reflection of the general hospital environment and the activities undertaken there. Free-living opportunist pathogens, such as Ps. aeruginosa, can normally be found in wet sites, such as drains, sinks and taps. Cleaning equipment, such as mops, buckets, cloths and scrubbing machines, may be responsible for distributing these organisms around the pharmacy; if stored wet they provide a convenient niche for microbial growth, resulting in heavy contamination of equipment. Contamination levels in the production environment may, however, be minimized by observing good manufacturing practices (GMP), by installing heating traps in sink U-bends, thus destroying one of the main reservoirs of contaminants, and by proper maintenance and storage of equipment, including cleaning equipment. Additionally, cleaning of production units by contractors should be carried out to a pharmaceutical specification.
4.1.1.3 Packaging
Sacking, cardboard, card liners, corks and paper are unsuitable for packaging pharmaceuticals, as they are heavily contaminated, for example with bacterial or fungal spores. These have now been replaced by nonbiodegradable plastic materials. In the past, packaging in hospitals was frequently reused for economic reasons. Large numbers of containers may be returned to the pharmacy, bringing with them microbial contaminants introduced during use in the wards. Particular problems have been encountered with disinfectant solutions where residues of old stock have been ‘topped up’ with fresh supplies, resulting in the issue of contaminated solutions to wards. Reusable containers must therefore be thoroughly washed and dried, and never refilled directly.
Another common practice in hospitals is the repackaging of products purchased in bulk into smaller containers. Increased handling of the product inevitably increases the risk of contamination, as shown by one survey when hospital-repacked items were found to be contaminated twice as often as those in the original pack (Public Health Laboratory Service Report, 1971).
4.2 In use
Pharmaceutical manufacturers may justly argue that their responsibility ends with the supply of a well-preserved product of high microbiological standard in a suitable pack and that the subsequent use, or indeed abuse, of the product is of little concern to them. Although much less is known about how products become contaminated during use, their continued use in a contaminated state is clearly undesirable, particularly in hospitals where it could result in the spread of cross-infection. All multidose products are vulnerable to contamination during use. Regardless of whether products are used in hospital or in the community environment, the sources of contamination are the same, but opportunities for observing it are greater in the former. Although the risk of contamination during product use has been much reduced in recent years, primarily through improvements in packaging and changes in nursing practices, it is nevertheless salutary to reflect upon past reported case histories.
4.2.1 Human sources
During normal usage, patients may contaminate their medicine with their own microbial flora; subsequent use of such products may or may not result in self-infection (Figure 17.3).
< div class='tao-gold-member'>
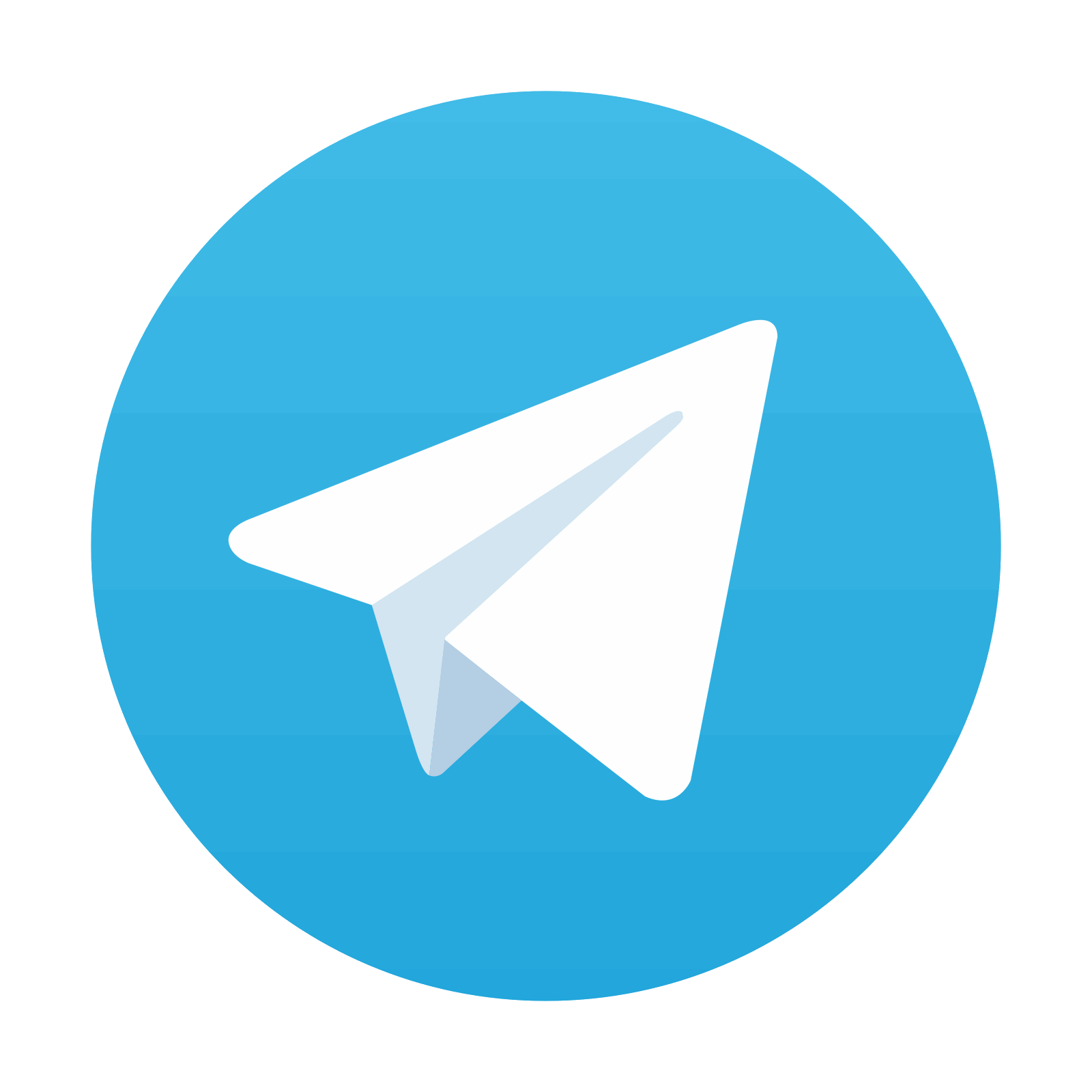