8 Maya Davidovich-Pinhas and Havazelet Bianco-Peled Department of Chemical Engineering Technion – Israel Institute of Technology Israel Mucoadhesion is a specific example of the more general phenomenon of adhesion. The origin of the term ‘adhesion’ is the Latin word adhaerere (ad = to, haerere = stick) [1]. The classical definition of adhesion is an assembly made by the use of an adhesive material between two other material surfaces (substrate) that creates a joint resisting separation [2]. Two forces act together to prevent breakage of an adhesion joint: adhesion and cohesion. The adhesive force is responsible for forming the intimate contact between the molecules of the adhesive and the atoms or molecules on the substrate surface. This process can be referred to as wetting where the adhesive material is spread on the substrate [2]. The cohesive force results from interactions between the adhesive’s molecules and can be expressed as the work which is required to break the adhesive material and form two new surfaces. Adhesives are typically applied on the surface in a liquid form to allow wetting, and then hardened by a chemical reaction, loss of solvent or water and so on to achieve good cohesion [2]. Six theories describing the adhesion phenomenon and its relationship to different exterior forces have been published [2–4]. Each of these theories is valid to some extent, depending on the nature of the material in contact and the condition of the process, and various types of forces could be activated simultaneously. The physical adsorption theory of adhesion is the most widely used approach. This theory attributes the adhesion to van der Waals forces between permanent dipoles and induced dipoles across the interface. The chemical bonding theory of adhesion invokes the formation of covalent, ionic or hydrogen bonds across the interphase. The diffusion theory of adhesion is based on the assumption that the adhesive strength of polymers to themselves (autoadhesion) or to each other is induced due to mutual diffusion (interdiffusion) of macromolecules across the interphase. The electronic theory was originally developed for metals where electrons transferred from one metal to the other forming an electrical double layer that results in a force of attraction. According to the mechanical interlocking theory, adhesion occurs due to penetration of adhesive into the cavities, pores and asperities of the solid surface. The weak boundary layer theory proposes that clean surfaces can produce strong bonds to adhesives, but contaminants such as rust, oils or greases form a layer which is cohesively weak. The adherence of mucoadhesive polymers is an outcome of their physical and/or chemical interactions with the mucin glycoproteins. These glycoproteins are the basic component in the mucus and are responsible for its gel structure [5]. Noncovalent bonds such as hydrogen bonds, van der Waals forces, ionic interactions and/or chain entanglements are the most common interactions between polymers and mucin [6]. In addition, due to the negative surface charge of the mucus, electrostatic interactions play an important role in the adhesion process [5]. Therefore, it is believed that the mechanisms involved in the mucoadhesion process are mostly surface energy thermodynamics, interpenetration/diffusion and formation of chemical bonds. Thus, the mucoadhesion process is established first by wetting and adsorption of the polymer on the mucus to create intimate contact, followed by interdiffusion or interpenetration of the mucoadhesive material. In some cases, the final bond formation is established by secondary chemical interactions further strengthening the adhesive and the interphase bond [7]. This chapter describes a variety of experimental methods that have been proposed over the years for the evaluation of adhesion ability. The ability to evaluate mucoadhesion properties depends to a great extent on the model surface used in a specific research. The state of the mucus model surface and its content are crucial parameters affecting the results of the bioadhesion assay. The use of fresh mucus surface is obvious due to its ability to imitate the physiological environment [8–12]. Therefore, most studies use fresh mucosa surfaces obtained from a local slaughterhouse. However, the availability of a fresh tissue might be limited and it has a very short shelf life. In addition, the tissue content can vary due to different animal condition and nutrition. Therefore, several new strategies have been developed to overcome those limitations. The use of frozen tissue sample avoids issues related to a viability and storage. However, the effect of freezing on the tissue characteristics is not fully understood [13–17]. A preliminary histological study by Mortazavi et al. [18] on rat small intestine mucosal surface indicated that the damage from the freezing and thawing processes is minimal. The same research group developed a membrane surface that imitates the fresh mucus surface. The fabrication technique involves scraping of fresh hog stomachs, which were stored at −20 °C. Before use the material was thawed to room temperature, gently blended in order to ensure homogeneity and then spread over a Whatman membrane filter to obtain a model gel surface. Another ex vivo mucosa surface model described in the literature uses an epithelial cell line grown in a controlled environment. This strategy employs different type of mammalian epithelial cell lines, such as GSM06 [19], GSM10 [20], Caco-2, HT29, E12 [21], and Guinea pig gastric epithelial cell [22]. Cells are grown on a collagen covered membrane surface, which can be inserted into a standard tissue culture plates or Transwells. The use of ‘as received’ commercial mucin powder reduces cost and increases availability. Some use it as a concentrated aqueous solution (gel) [23–25], others as a partially hydrated compressed disc [26] or partially hydrated film [27]. Commercial mucin was also used in hydrated form by absorbing mucin solution on a filter paper disc [9,28,29]. Another attempt to develop a simplified in vitro gastric mucus model used a combination of crude pig gastric mucin with polycarbophil spread on a parafilm surface. This surface was dipped into a L-α-phosphatidylcholine (LPC) liposomal dispersion in order to obtained a gel coated with LPC molecules [30]. Attempts have been made to use synthetic surface models due to their low cost and reproducibility. Hydrated cellulose dialysis membrane was used as a model substrate for mucosa in a mucoadhesion tensile analysis [31]. Mucus simulant gels having similar viscoelastic properties to real human airway mucus were also studied. A synthetic gel was prepared by mixing various proportions of locust bean gun solution with tetraborate sodium [32]. Blanco-Fuente et al. [33] have developed a semi-synthetic surface model based on tanned leather. They demonstrated a good correlation between the results obtained using tanned leather and sublingual mucosa, using carbopol as a mucoadhesive polymer. Recently, Hall and co-workers [34] reported on a successful attempt to develop a synthetic model surface capable of fully mimicking the adhesive properties of biological mucosa surface. This model surface was synthesized as a three-dimensional hydrogel matrix by copolymerization of 2-hydroxyethylmethacrylate (HEMA) with various co-monomers, such as N-vinylpyrrolidone (NVP), 2-hydroxyethylacrylate (HEA), sorbitol methacrylate (SMA) and N-acryloyl glucosamine (AGA) and N-N′-methylenebisacrylamide as a cross-linking agent. The underlying hypothesis of this research was that the presence of sugar-like functional groups in both SMA and AGA monomers could mimic the oligosaccharide side chains present in native mucin glycoproteins. The chemical and physical properties of the model surfaces could be tuned using different co-monomers, HEMA/co-monomer ratio and cross-linking agent concentration. This study has demonstrated excellent mucoadhesion properties with HEMA–AGA hydrogels. Testing is an essential step for the development, characterization and proper use of any mucoadhesive system. However, it is not easy to extrapolate the behaviour of a mucoadhesive system from an in vitro test to its performance in vivo. This is because in vitro testing is generally performed under controlled conditions and environment, in contrast to the constantly varying conditions occurring in vivo [18]. Therefore, many approaches have been used over the years in order to develop the most accurate, simple and easy test set-up to measure mucoadhesion ability. There are three types of adhesion assays that are commonly used in order to evaluate adhesion properties: tensile, shear and peeling (Figure 8.1). In a tensile (Figure 8.1a) test a machine is used to measure the applied force needed to detach the substrates in the axial dimension. In a shear test (Figure 8.1b) the machine will measure the applied force needed to detach the two substrates in a tangential axis. In a peeling test (Figure 8.1c) at least one of the substrates is made from a flexible material that could plastically deform during measurements. Typically, a flexible tape is bonded using the adhesive material to a rigid substrate and then peeled off. The peeling force, P, is assumed to produce a steady rate of peeling [4]. Figure 8.1 Types of forces commonly applied in adhesion assays. Most of the adhesion tests developed for mucoadhesive systems can be classified into one of the first two assays discussed above. However, there are variety of other new methods developed in order evaluate mucoadhesion ability on the macroscopic and microscopic level, such as spectroscopic methods, contact angle, dielectric measurements and so on. The basic principle of tensile assays is measuring the force needed to detach mucoadhesive material from mucus surface. The results are usually presented as the maximum detachment force (MDF) and the area under the curve, which is the total work of adhesion (TWA) of the measured load-extension curve [17,18,27]. A variety of operation modes are described in the literature; they can be classified according to the hydration of the mucoadhesive sample (dry, hydrated or semi-hydrated), sample form (tablet, film, powder and particles), test environment (wet or dry) and mucus surface type (fresh, frozen, commercial mucin powder, commercial mucin solution or semi-hydrated discs). Several instruments including texture analyser and tensiometer have been used. The operating conditions such as detachment speed, pre-load strength and contact time vary from study to study, as does the way to measure force: load cells or regular weights. The sample’s state of hydration is a key element affecting properties such as drug release profile and bioadhesiveness [35,36]. The use of a dry polymer sample for adhesion measurements is the most common [8,25,27,37–40]. Bernkop-Schnurch and co-workers [8,38] used dry compressed polymer tablets glued to a stainless steel grid attached to a nylon thread fixed to a laboratory stand. The mucus surface was glued to a lower platform which came into contact with the polymer tablet. The test was performed by pulling the lower platform at a rate of 6 mm/min while recording the force. This experimental set-up was further modified by placing the polymer tablet between two fresh mucus surfaces attached to both of the tensiometer arms for a fixed time, followed by pulling either the upper arm [41] or the lower instrument platform [42] at a constant rate. Another method employs polymer powder attached directly to the instrument probe [9,14]. The same methodology was used for measuring the mucoadhesion ability of microparticles, where dry microparticles prepared by a ‘water-in-oil emulsification’ method were mounted on the test probe using double-sided adhesive [15,43]. Other tests use dry polymer films prepared by pouring polymer solution onto an inert surface [10,23,44], immersing thin glass in polymer solution [45] or using a casting method that creates a polymer film inside a mould [46] and drying it evenly. The measurements are performed by the same manner as for dry tablets. Semi-dry materials are usually dry samples that were lightly hydrated using a known amount of solvent prior to their contact with mucus surface [17,24,25,27,35]. Several studies promote surface hydration by the addition of small amount of solvent, such as buffer [47] or mucin solution [48], to the mucosa surface model. The use of dry or semi-dry polymer sample can probably enhance the adhesion due to diffusion and interpenetration of polymer chains. However, such a format could not be used for encapsulation of living cells for therapeutic treatment. Fully hydrated samples were used by Davidovich-Pinhas and Bianco-Peled [49]. Cross-linked hydrated hydrogels were created and their adhesion to fresh mucus surface was monitored. The hydrated hydrogel tablets were attached to the upper arm of Lloyed tensile machine and the mucus to the lower. Measurements were performed by raising the upper arm. Another method of assessing adhesion of hydrated polymer gel involves packing the gels in a vessel and attaching the mucus sample to the upper arm of tensiometer machine, which was then lowered until it came into contact with the gel vessel connected to the lower arm [11,13,16,24,26,50]. Ferrari et al. [29], Caramella et al. [28] and more recently Edsman et al. [9] used a filter paper disc mounted with polymer solution attached to the sample holder, which was brought into contact with mucus surface for a fixed time and then pulled at a constant rate. Several studies used a wet environment during the mucoadhesion test in an attempt to mimic the physiological condition in the human body, where mucus-covered surfaces are constantly hydrated. Tensile tests were performed while both surfaces, the tested polymer and the mucus, were immersed in test liquid [8,10,12,51,52] throughout the measurements. The assays are similar to the ones used for dry samples, that is the surfaces are put in contact for a fixed period of time and then pulled in opposite directions. The force needed to separate the surfaces is considered as a measure of adhesion. Another tensile technique is designed to characterize bioadhesion of microspheres to intestinal tissue. This method uses a Cahn dynamic contact angle analyser, which is usually used for measuring contact angle or surface tension, as a microtensiometer instrument [53–57]. The instrument is equipped with an accurate and sensitive microbalance enabling detection of slight changes in the measured force. The testing procedure involved placing a tissue specimen in a temperature control and buffer surrounding environment on the mobile stage, attaching a single polymer microsphere to the balance and raising the tissue chamber until the microsphere was completely submerged in the buffer solution. Then the chamber stage was raised at a constant rate until contact was achieved between the microsphere and tissue. Following contact for a fixed period of time under the applied force, the sample was pulled vertically away while recording the required detachment force. Tensile force assay can be also measured by recording the normal force applied during a compression experiment using texture analyser instrument. This assay involves penetration of a probe into the sample at a pre-defined depth, force and velocity [58]. The adhesiveness can be referred to as the force required to overcome the interactions between the sample surface and the probe, or is calculated from the area under the force versus distance plot. Silva et al. [58] measured the force required for probe penetration into a polymer gel sample, while Nep and Conway [59] compared penetration and withdrawal profile obtained for a polymer, mucin gel and their mixture. Shear assays aim to quantify mucoadhesion by characterizing the ability of two surfaces to interact under applied shear forces. A number of assays that differ in their specific design are described in the literature. The ability of mucoadhesive formulations to maintain contact with a mucus surface under shear forces in a wet environment was evaluated using the rotating cylinder method. Bernkop-Scnurch and Steininger [60] were the first to propose this type of measurement. Their aim was to develop an adhesion assay which can evaluate the adhesion ability as well as the sample cohesiveness. Dry compressed tablets were attached to fresh mucus surface glued to a stainless steel cylinder. The cylinder was fully immersed in buffer solution and spun at 250 rpm. Detachment, disintegration and/or erosion of the tablets was monitored over a period of 10 hours. This type of experimental set-up was further used in several studies for the characterization of various polymer systems [52,61]. In most cases, the rotating cylinder method was combined with another type of mucoadhesion technique, such as tensile test measurements [62–68]. The set-up introduced by Bernkop-Scnurch and Steininger was further modified in order to allow characterization of polymers that are not in the form of tablets. Hagesaether et al. [69] investigated the mucoadhesive properties of zinc-pectinate hydrogel beads by means of the rotating cylinder method. The experimental procedure included adherence of gel beads to fresh mucus surface attached to cylinder for two minutes. The cylinder was then immersed in buffer solution and agitated at 300 rpm for 10 min. The results were expressed as the percentage of beads remaining attached to the fresh intestine at the end of each experiment. Flow assays measure the ability of a polymer to maintain binding with the mucus surface under shear forces subjected as a continuous flow. This type of method was first introduced by Rao and Buri in 1989 [70]. In this study glass spheres were coated with the polymer to be tested and a known amount of these particles were placed on a fresh mucus tissue for a fixed time in a humid environment in order to allow the polymer to hydrate and interact with mucin and to prevent the drying of the mucus tissue. The experiments were performed by washing the mucus surface at a constant rate for fixed time with phosphate buffer or dilute HCl solution. The percentage of beads washed away was determined by weighting the outlet product after drying. The results were considered to be a measure of bioadhesion calculated from the percentage of particles retained on the tissue. Similar procedures were used recently by Blegamwar et al. [68] and Alli et al. [71] to evaluate the mucoadhesiveness of polymeric microparticles. Nielsen et al. [44] developed this procedure further by placing the flow cell in a temperature-controlled container where the effluent solution was analysed by reversed phase high-performance liquid chromatography (HPLC) in order to determine the polymer content in it. The experiment was carried out by placing the polymer sample on a defrosted mucus tissue for fixed time and then washing it at a constant rate for a fixed time. The effluent was collected in a beaker and further analysed by HPLC to evaluate the amount of compound remained on the tissue. Recovery of at least 70% w/w of the applied sample on the mucus surface was taken as an indication of good mucoadhesion ability. This flow cell experiment can be referred to as a kind of ‘all or none’ test. In the cases mentioned above the tip of the tube carrying the washing solution was placed 2–3 mm above the tissue in order to ensure an even flow of liquid over the subjected mucus surface. To ensure an evenly distributed flow over the tested tissue, Batchelor et al. [72] separated the washing solution into four channels. The polymer was labelled and its concentration in the eluted solution was determined by fluorometric analysis. The results were expressed as the percentage dose retention with respect to the initial dose mounted on the mucus surface. The use of spectrophotometer analysis in order to monitor effluent content was also adopted by Le Ray et al. [73] who used a different flow adhesion cell consisting of a glass tube open at the top and bottom allowing free circulation of liquid. The mucus surface was introduced into the glass tube and fixed at its upper and lower ends. A coloured gel sample was mounted on the fresh intestine or intestine substitute (polyethylene type) using a syringe. After 10 minutes, the apparatus was returned to a vertical position and continuous flow at fixed rate was applied through the tube. The effluent solution was collected and further analysed by spectrophotometer. The results were expressed as the percentage of retained colour normalized with respect to the total amount recovered with time. Mikos and Peppas [74] used a channel flow device having a rectangular cross-sectional area. The tested mucus surface was placed on a mold cavity in the middle of the device where a polymer particle was placed. The lid was closed and the particles remained in contact with the surface for five minutes. Afterwards the flow rate was gradually increased until detachment of the particles occurred. A similar procedure, where the flow rate was fixed and the time needed for detachment was measured, was employed as well. The wash-off experiment measures the amount of microparticles that remain on a mucosa tissue surface after applying an agitating force. Fresh mucosa tissue was mounted on a glass slides using a commercial adhesive material. A known amount of microparticles was spread on the tissue and immediately thereafter the slides connected to a support were hung onto a USP arm disintegrating test apparatus. Then the machine started to perform a slow up and down movement inside to simulate gastric and intestinal fluid. The process was stopped at fixed intervals and the amount of particles still connected to the tissue was measured. The results were expressed as the ratio of particles remaining on the surface with respect to the initial amount [75,76]. A similar procedure was used to evaluate adhesion of films. A mucoadhesive film was attached to a mucosa surface mounted on a USP disintegrating test apparatus moving in a fluid simulant. The time necessary for complete erosion or detachment of the patch film was recorded [77]. Nanoscale shear methods usually require labelled particles due to the scale limitation. Therefore, fluorescein isothiocyanate (FITC)-labelled mucoadhesive nanoparticles were used in order to measure bioadhesion of nanoparticles to the mucosa surface in fluid simulant under agitation. The tissue was exposed to the labelled nanoparticle solution for a fixed time in order to obtain bioadhesion. Afterwards the tissue was immersed in fluid simulant for a fixed time. The analysis was performed by measuring the fluorescence of the fluid simulant before and after tissue insertion using a microplate reader [78]. The sliding parallel plate method measures the force required to separate or slide a coated mucoadhesive surface from the gel mucosa layer in a direction parallel to their place of contact of adhesion. The procedure includes coating one side of two glass plates with mucoadhesive agent followed by placing the mucosal layer between them (Figure 8.2) [42]. Another experimental set-up uses two smooth, polished plexiglass blocks with the mucoadhesive agent placed directly between them [79]. In both cases, the results are described in terms of the force required to separate the two surfaces [42,79]. Figure 8.2 Schematic illustration of the sliding plate experimental set-up. The Wilhelmy plate method includes suspension of a plate covered with mucoadhesive agent in tested solution for fixed time to achieve equilibrium followed by the continuously measurement of the force required to vertically pull this plate from the solution (Figure 8.3) [80]. The experimental set-up uses viscous mucus solution extracted from goat intestine [42] or gastric model fluid (buffer solution pH 1.2) [79]. Figure 8.3 Schematic illustration of the Wilhelmy plate method experimental set-up. The use of the peel test to evaluate mucoadhesion ability is rare. de Vries and co-workers [81] used a peel test in order to evaluate the adhesion of hydrogel strip to porcine tissue. The sample was allowed to set for 15 minutes before peel force was applied. The angle of the peel test remained 90° by moving the sample forward. The mucoadhesion process involves the association of the adhesion components through polymer entanglements, penetration, chain diffusion and chemical interactions. Therefore, rheology, the study of flow and deformation of materials [82], can offer a convenient means to monitor those interactions. In a rheology experiment, the influence of mucin addition on either the viscosity of a polymer solution or the frequency dependence of the storage and loss modulus is measured. The magnitude of change on these parameters is considered to be a measure of mucoadhesion. The viscosity of mucin dispersion is the total resistance to flow exerted by chain entanglements, noncovalent bonds such as hydrogen, electrostatic and hydrophobic bonding and covalent bonds such as disulfide bridges. These interactions are also the ones participating in mucin/polymer mucoadhesion [83]. Therefore, those interactions could be monitored by viscosity changes occurring due to an increase in the total sum interactions, which can be referred to as mucoadhesion. The use of a simple rheology method in order to assess mucin–polymer mucoadhesion was first introduced by Hassan and Gallo in 1990 [83]. In their work viscosity changes appearing upon mucin addition to polymer solution were monitored. The following empirical equation was proposed to describe the results
Methods to Study Mucoadhesive Dosage Forms
8.1 Introduction
8.1.1 Theories of Adhesion
8.1.2 Mucoadhesion
8.2 Model Surfaces for Mucoadhesion Evaluation
8.3 Methods to Evaluate Mucoadhesion Dosage Form
8.3.1 Tensile Assays
8.3.2 Shear Assays
8.3.2.1 Rotating Cylinder Method
8.3.2.2 Flow Assays
8.3.2.3 Wash-Off Experiment
8.3.2.4 Nanoscale Wash-Off Experiment
8.3.2.5 Sliding Parallel Plate
8.3.2.6 Wilhelmy Plate Method
8.3.3 Peel Test
8.3.4 Other Methods
8.3.4.1 Rheology
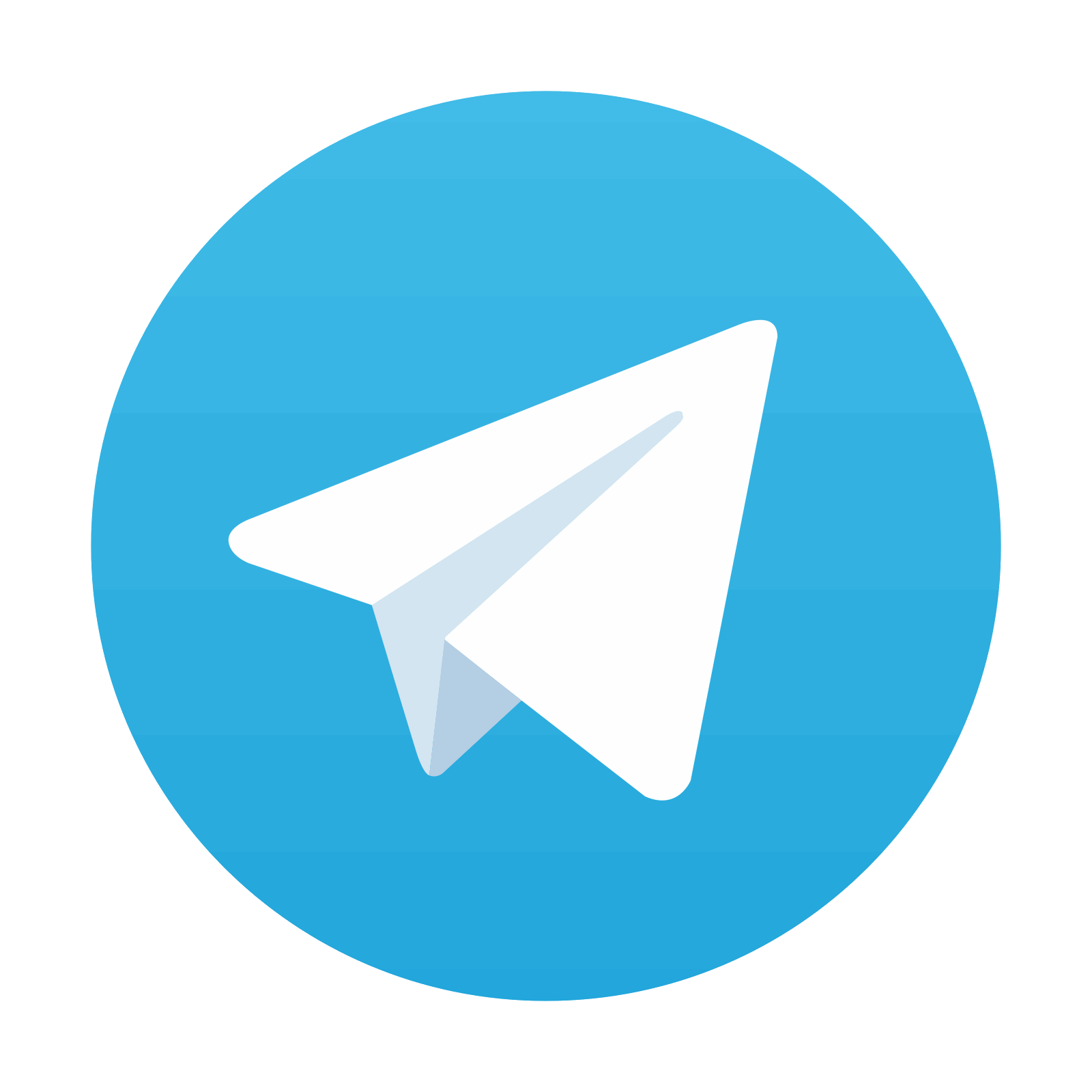
Stay updated, free articles. Join our Telegram channel

Full access? Get Clinical Tree
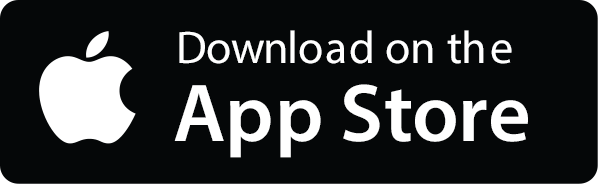
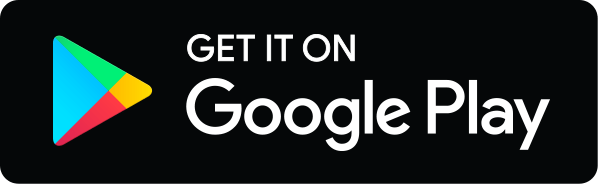