Fig. 10.1
(a) Preoperative radiograph of ankle fracture. (b) Postoperative radiograph of plate fixation for ankle fracture
For transverse or oblique types of fracture of long bones such as femur and humerus, an intramedullary nail or rod is inserted into the medullary canal of the fractured long bone to stabilize the fracture site (Fig. 10.2) [7–9].
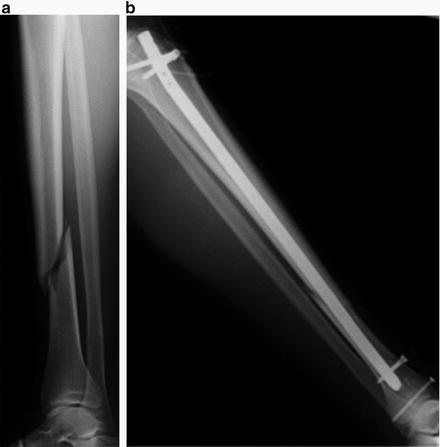
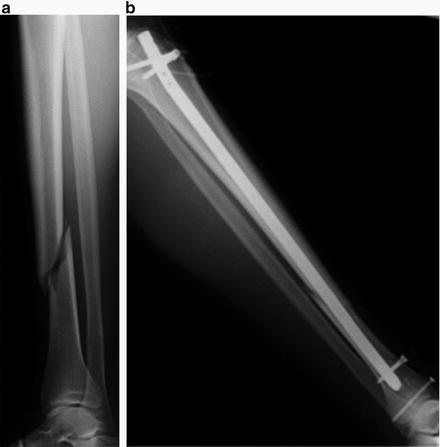
Fig. 10.2
(a) Preoperative radiograph of tibial shaft fracture. (b) Postoperative radiograph of intramedullary nail for the tibia
For open fractures which are exposed to the air outside the body by severe soft tissue injuries over the fractures, external fixators are best indicated not only to stabilize the comminuted fracture but also to handle the risk of postoperative infection (Fig. 10.3) [10].
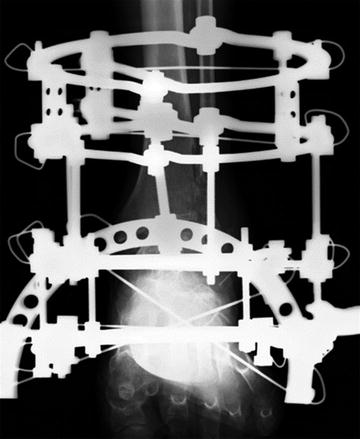
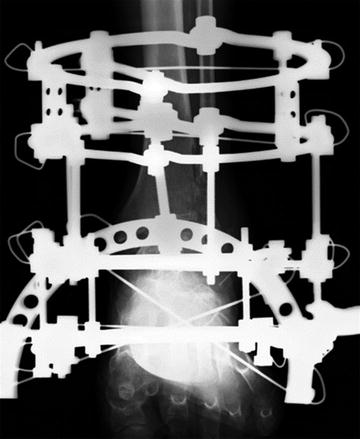
Fig. 10.3
External fixator for fusion at the ankle joint
Another indication of an external fixator is leg length discrepancy or severe deformity of the extremities due to malunion after fractures and prolonged infection [11]. Gradual lengthening by distraction is possible by using external fixators until the final goal of lengthening is achieved.
To obtain complete biological bony union, rigid stabilization at the site of the fracture should be maintained for several months. The excellent mechanical stiffness of metallic implants is beneficial to obtain biological solid union at the fracture site by providing better stability. The bony healing process at the fracture sites starts by the formation of soft immature woven bone between the gaps, which is followed by the remodeling phase with formation of rigid mature bone in which collagen fibers are perfectly aligned to provide mechanical strength to the fracture sites. Physiological mechanical stress may lead immature soft bone to develop more rigid cortical bone to sustain the load at the fracture site.
10.2.2 Stress-Shielding Effect
Since metallic implants have significantly higher mechanical properties than human cortical bones, long-term fixation by rigid metallic implants over the fracture sites would decrease mechanical stress and end up enhancing bone resorption at the bone under the metallic implants [12, 13]. This effect is called device-related osteopenia or osteoporosis by activating osteoclasts by stress-shielding effect. This stress-shielding effect should be avoided to maintain the bone quality, so the removal of metallic implants is recommended especially in the weight-bearing bones after solid bony fusion is obtained at the fracture site after a certain period of time. In clinical situations, removal of metallic implants such as screws and plates over fracture sites in the upper or lower extremities is commonly performed later than postoperative 1 year. Removal of metallic implants in the extremities is pretty simple and does not require difficult surgical interventions. Many orthopedic surgeons, therefore, recommend patients for removal of metallic implants overt the fracture sites to enhance bone remodeling and solid biological fusion. Stress-shielding effect occurs not only at the extremities but also at the spinal column. Removal of metallic implants from the spine, however, often requires invasive surgical procedures for back muscles so that many spine surgeons ask patients for removal of spinal implants only when serious implant-related problems are seen.
10.2.3 Biocompatibility
Though stainless steel had been the most commonly used in clinics for fracture fixation, fracture fixation devices made of titanium alloys have become most popular in recent years. Since titanium alloys have excellent biocompatibility, new bone formation can be observed over the titanium fixation devices, which makes removal of implants difficult in some patients when abundant new bone formation occurs over the implants [14]. New bone formation over stainless steel is much less than that of titanium alloys so that stainless steel devices are preferable in some patients who need removal of metallic implants long after surgery. Absorbable screws and plates made of absorbable PLLA are now clinically available to prevent stress-shielding effect in order to avoid implant removal after the initial surgery [15, 16].
10.2.4 Artifacts and Heat Production in MRI (Magnetic Resonance Image) Scan
One of the demerits of using metallic implants for orthopedic surgery is a formation of artifacts around the metallic implants on MR images, which disturb clinical assessment at the surrounding tissues (Fig. 10.4) [17].
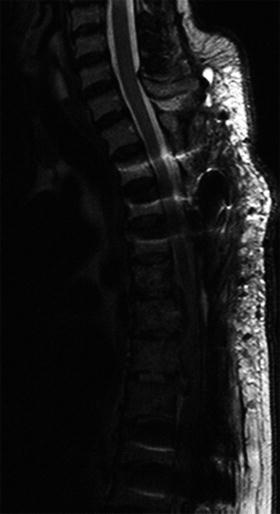
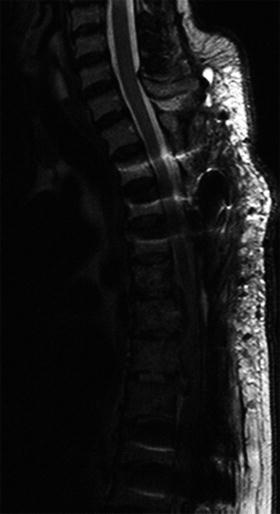
Fig. 10.4
Metal artifact on MR image in the thoracic spine
When metal implants are placed in the spine, device-related artifacts interfere with the ability of clinicians to assess further neural disorders next to the implants. Another concern is that metallic implants may impart heat to the surrounding tissues while taking MR images (Fig. 10.5) [18].
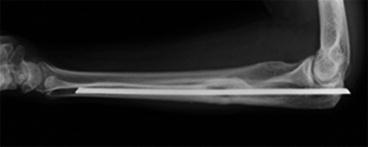
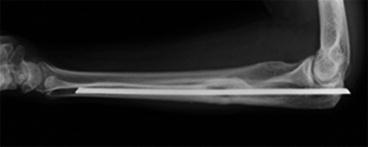
Fig. 10.5
A patient with heat production around a metallic implant during MRI scan
Higher magnetic fields have a higher risk of producing significant heat affecting the surrounding soft tissue, so radiologists should be aware of this effect in patients with orthopedic metal implants.
10.3 Artificial Joints
10.3.1 Indications of Joint Replacement
Artificial joints are indicated for patients with severe destruction of any joints from small finger joints to large weight-bearing joints due to trauma, osteoarthritis, or tumors. The biggest role of artificial joints is to restore the damaged joint functions to maintain the ambulatory functions of those who became unable to stand or walk with severely damaged joints. Artificial joints are now available for hip (Fig. 10.6), knee (Fig. 10.7) shoulder (Fig. 10.8), elbow, ankle (Fig. 10.9), wrist, and even fingers (Fig. 10.10).
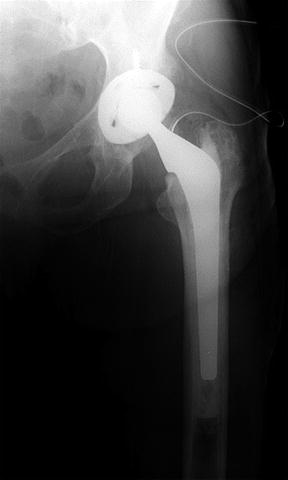
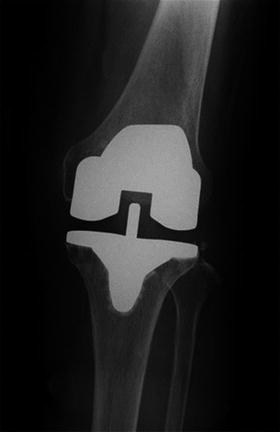
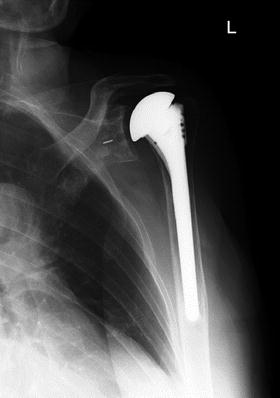
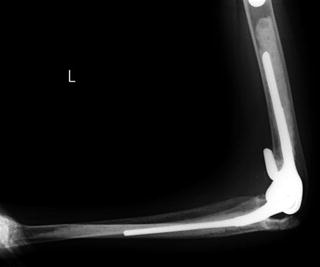
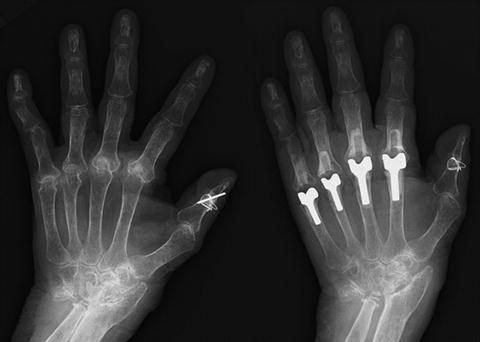
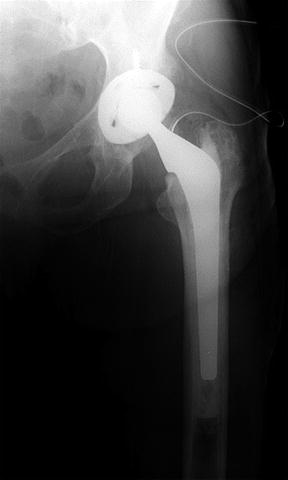
Fig. 10.6
Postoperative radiograph of total hip replacement
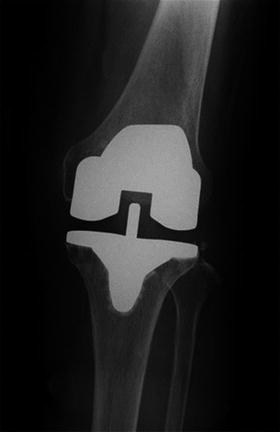
Fig. 10.7
Postoperative radiograph of total knee replacement
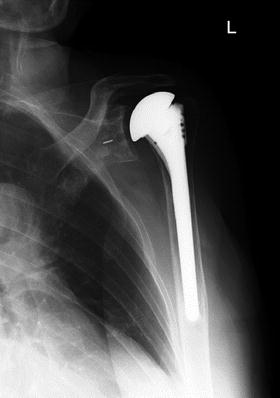
Fig. 10.8
Postoperative radiograph of total shoulder replacement
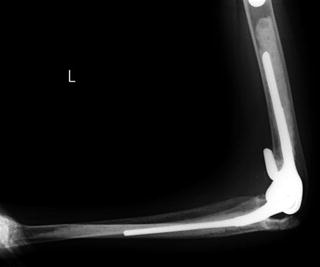
Fig. 10.9
Postoperative radiograph of total elbow replacement
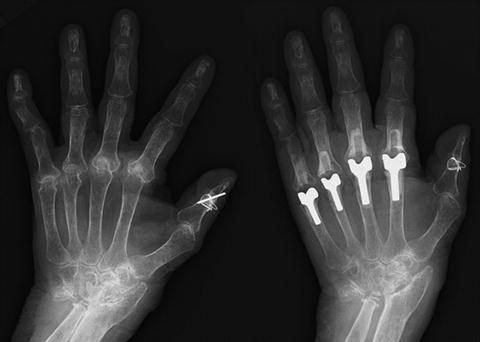
Fig. 10.10
Pre- and postoperative radiograph of total finger joint replacement
The major sales of artificial joints in the whole world were hip and knee joints, and the value of the market in 2011 was worth $12.8 billion [19]. Due to a rapid and steady increase in the aged population, the demand for artificial joints is increasing to treat degenerative joint disorders in the elderly.
10.3.2 Total Hip Arthroplasty
The first total hip arthroplasty (THA) was invented and introduced in the 1960s by Sir Charnley, and the Charnley system is still widely used in the present orthopedic field [20, 21]. Artificial hip joints are formed by two components, corresponding to the femoral side and acetabular side. The acetabular component consists of cup and liner. The femoral component of the Charnley THA consists of a stem and head made of stainless steel and Co-Cr-Mo or Ti alloys. The acetabular component is a socket made of ultrahigh molecular weight polyethylene (UHMWPE) which is fixed to the pelvis with bone cement (polymethylmethacrylate, PMMA). Due to the friction between the cup (polymer) and femoral head (metal) under repetitive motion of the hip joints, wear debris produced at the contact area of polymer and metal would lead to osteolysis around the components and end up with loosening of the artificial joints long after the initial surgery (Fig. 10.11) [22–29].
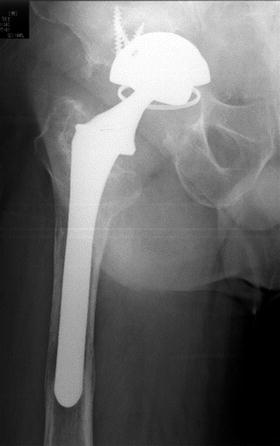
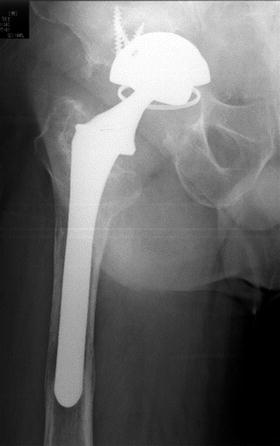
Fig. 10.11
Loosening of the acetabular component of artificial hip joint
The survival rate of the Charnley THA at postoperative 25 years was 90 % with the femoral component and 75 % of the acetabular component [29, 30]. Since many patients who underwent THA would live longer than 25 years after the first surgery, there are increasing numbers of patients who need revision surgery due to the loosening of the prostheses in recent years. Longer survival of prosthesis function is a major clinical goal for the manufacturers of artificial joints.
10.3.3 Prosthetic Loosening
The predisposing factors of prosthesis loosening in THA are not only debris due to the friction between the acetabular and femoral components but also the dysfunction of lubrication between the two components. The amount of debris formed at the joint surface depends on the combination of the materials used for the acetabular and femoral components. The worst combination of the two components is that of a metal head and a polyethylene cup [23, 24]. Recently, combination of ceramics on ceramics and metal on metal is under investigation to establish artificial joints with less debris formation. Ceramics, however, do not have sufficient fracture toughness and may easily break under physiological loading conditions. Metal on metal would be acceptable from the mechanical point of view, but may produce metal debris in the joints. Co-Cr-Mo is now used for acetabular components and has gained popularity in European countries due to its wear resistance. Another attempt to improve the wear resistance of UHMWPE is gamma ray exposure followed by heat treatment, which needs further investigation to prove its long-term clinical efficacy and safety [31].
10.3.4 PMMA and Surface Modifications
PMMA cement has been extensively used for attachment between the host bone and the femoral or acetabular components. PMMA cement has benefits to fix bone and both components within a very short period of time, but PMMA creates heat over 60° which can damage the surrounding tissues, and toxic residual monomers of PMMA are of significant clinical concern. PMMA injection to the intramedullary channel in the long bones has a great potential risk of lung or brain embolism which could cause serious life-threatening complications in the patients. In order to prevent such catastrophic complications, surface modifications of the metal and more osteoconductive materials have gained their popularity to achieve direct bonding between the host bone and the components without PMMA cement. Artificial joints without PMMA cement are called cementless systems, and those requiring very small amount of PMMA are hybrid systems [32]. One of the surface modifications to obtain better bone ingrowth into the surface of the component is plasma spray coating on Ti to create an irregular surface on the component and fine grooves for bony ingrowth to have a strong bone and metal attachment (Fig. 10.12) [33, 34].
