Fuel Metabolism. We obtain our fuel primarily from the macronutrients (i.e., carbohydrates, fats, and proteins) in our diet. As we eat, our foodstuffs are digested and absorbed. The products of digestion circulate in the blood, enter various tissues, and are eventually taken up by cells and oxidized to produce energy. To completely convert our fuels to carbon dioxide (CO2) and water (H2O), molecular oxygen (O2) is required. We breathe to obtain this oxygen and to eliminate the carbon dioxide (CO2) that is produced by the oxidation of our food.
Fuel Stores. Any dietary fuel that exceeds the body’s immediate energy needs is stored, mainly as triacylglycerol (fat) in adipose tissue; as glycogen (a carbohydrate) in muscle, liver, and other cells; and, to some extent, as protein in muscle. When we are fasting, between meals and overnight while we sleep, fuel is drawn from these stores and is oxidized to provide energy (Fig. 1.1).
FIGURE 1.1 Fate of excess dietary fuel in fed and fasting states.
Fuel Requirements. We require enough energy each day to drive the basic functions of our bodies and to support our physical activity. If we do not consume enough food each day to supply that much energy, the body’s fuel stores supply the remainder and we lose weight. Conversely, if we consume more food than is required for the energy we expend, our body’s fuel stores enlarge and we gain weight.
Other Dietary Requirements. In addition to providing energy, the diet provides precursors for the biosynthesis of compounds necessary for cellular and tissue structure, function, and survival. Among these precursors are the essential fatty acids and essential amino acids (those that the body needs but cannot synthesize). The diet must also supply vitamins, minerals, and water.
Waste Disposal. Dietary components that we can utilize are referred to as nutrients. However, both the diet and the air we breathe contain xenobiotic compounds, compounds that have no use or value in the human body and may be toxic. These compounds are excreted in the urine and feces together with metabolic waste products.
THE WAITING ROOM 
Percy V. is a 59-year-old school teacher who was in good health until his wife died suddenly. Since that time, he has experienced an increasing degree of fatigue and has lost interest in many of the activities he previously enjoyed. Shortly after his wife’s death, one of his married children moved far from home. Since then, he has had little appetite for food. When a neighbor found him sleeping in his clothes, unkempt, and somewhat confused, she called an ambulance. Percy V. was admitted to the hospital psychiatry unit with a diagnosis of depression related to bereavement, dehydration, and malnutrition.
Otto S. is a 25-year-old medical student who was very athletic during high school and college but is now out of shape. Since he started medical school, he has been gaining weight. He is 5 ft 10 in tall and began medical school weighing 154 lb, within his ideal weight range. By the time he finished the last examination in his first year, he weighed 187 lb. He has decided to consult a physician at the student health service before the problem gets worse, as he would like to reduce his weight (at 187 lb, his body mass index [BMI] is 27) to his previous level of 154 lb (which would reduce his BMI to 23, in the middle of the healthy range of BMI values).
Ivan A. is a 56-year-old accountant who has been obese for a number of years. He exhibits abdominal obesity, which is caused by excess adipose tissue being disproportionally deposited around the organs in the abdomen. His major recreational activities are watching TV while drinking scotch and soda and doing occasional gardening. At a company picnic, he became very “winded” while playing softball and decided it was time for a general physical examination. At the examination, he weighed 264 lb at 5 ft 10 in tall. His blood pressure was elevated, 155 mm Hg systolic and 95 mm Hg diastolic (for Ivan A.’s age hypertension is defined as >130 mm Hg systolic and >80 mm Hg diastolic). For a male of these proportions, a BMI of 18.5 to 24.9 would correspond to a weight between 129 and 173 lb. Ivan A. is almost 100 lb overweight, and his BMI of 37.9 is in the range defined as obesity.
Ann R. is a 23-year-old buyer for a woman’s clothing store. Despite the fact that she is 5 ft 7 in tall and weighs 99 lb, she is convinced she is overweight. Two months ago, she started a daily physical activity program that consists of 1 hour of jogging every morning and 1 hour of walking every evening. She also decided to consult a physician about weight loss. If patients are above (like Ivan A.) or below (like Ann R.) their ideal weight, the physician, often in consultation with a registered dietitian, prescribes a diet designed to bring the weight into the ideal range.
I. Dietary Fuels
The major fuels we obtain from our diet are the macronutrients, namely carbohydrates, proteins, and fats. When these fuels are oxidized to CO2 and H2O in our cells (the process of catabolism), energy is released by the transfer of electrons to O2. The energy from this oxidation process generates heat and adenosine triphosphate (ATP) (Fig. 1.2). Carbon dioxide travels in the blood to the lungs where it is expired, and water is excreted in urine, sweat, and other secretions. Although the heat that is generated by fuel oxidation is used to maintain body temperature, the main purpose of fuel oxidation is to generate ATP. Adenosine triphosphate provides the energy that drives most of the energy-consuming processes in the cell, including biosynthetic reactions (anabolic pathways), muscle contraction, and active transport across membranes. As these processes utilize energy, ATP is converted back to adenosine diphosphate (ADP) and inorganic phosphate (Pi). The generation and utilization of ATP is referred to as the ATP–ADP cycle.
FIGURE 1.2 The ATP–ADP cycle. The energy-generating pathways are shown in red; the energy-utilizing pathways in blue. ADP, adenosine diphosphate; ATP, adenosine triphosphate; Pi, inorganic phosphate.
The oxidation of fuels to generate ATP is called respiration (Fig. 1.3). Prior to oxidation, carbohydrates are converted principally to glucose, fat to fatty acids, and protein to amino acids. The pathways for oxidizing glucose, fatty acids, and amino acids have many features in common. They first oxidize the fuels to acetyl coenzyme A (acetyl CoA), a precursor of the tricarboxylic acid (TCA) cycle. The TCA cycle is a series of reactions that completes the oxidation of fuels to CO2 (see Chapter 23). Electrons lost from the fuels during oxidative reactions are transferred to O2 by a series of proteins in the electron transport chain (see Chapter 24). The energy of electron transfer is used to convert ADP and Pi to ATP by a process known as oxidative phosphorylation.
FIGURE 1.3 Generation of ATP from fuel components during respiration. Glucose, fatty acids, and amino acids are oxidized to acetyl CoA, a substrate for the TCA cycle. In the TCA cycle, they are completely oxidized to CO2. As fuels are oxidized, electrons (e−) are transferred to O2 by the electron transport chain, and the energy gained by the electron transfers is used to generate ATP. acetyl CoA, acetyl coenzyme A; ATP, adenosine triphosphate; TCA, tricarboxylic acid.
In discussions of metabolism and nutrition, energy is often expressed in units of calories. A calorie in this context (nutritional calorie) is equivalent to 1 kilocalorie (kcal) in energy terms. “Calorie” was originally spelled with a capital C, but the capitalization was dropped as the term became popular. Thus, a 1-calorie soft drink actually has 1 kcal of energy. Energy is also expressed in joules. One kilocalorie equals 4.18 kilojoules (kJ). Physicians tend to use units of calories, in part because that is what their patients use and understand. One kilocalorie of energy is the amount of energy required to raise the temperature of 1 L of water by 1°C at atmospheric pressure.
A. Carbohydrates
The major carbohydrates in the human diet are starch, sucrose, lactose, fructose, maltose, galactose, and glucose. The polysaccharide starch is the storage form of carbohydrates in plants. Sucrose (table sugar), maltose, and lactose (milk sugar) are disaccharides, and fructose, galactose, and glucose are monosaccharides. Digestion converts the larger carbohydrates to monosaccharides, which can be absorbed into the bloodstream. Glucose, a monosaccharide, is the predominant sugar in human blood (Fig. 1.4).
FIGURE 1.4 Structure of starch and glycogen. Starch, our major dietary carbohydrate, and glycogen, the body’s storage form of glucose, have similar structures. They are polysaccharides (many sugar units) composed of glucose, which is a monosaccharide (one sugar unit).
Oxidation of carbohydrates to CO2 and H2O in the body produces approximately 4 kcal/g (Table 1.1). In other words, every gram of carbohydrate we eat yields approximately 4 kcal of energy. Note that carbohydrate molecules contain a significant amount of oxygen and are already partially oxidized before they enter our bodies (see Fig. 1.4).
TABLE 1.1 Caloric Content of Fuels
FUEL | kcal/g |
Carbohydrate | 4 |
Fat | 9 |
Protein | 4 |
Alcohol | 7 |
B. Proteins
Proteins are composed of amino acids that are joined to form linear chains (Fig. 1.5). In addition to carbon, hydrogen, and oxygen, proteins contain approximately 16% nitrogen by weight. The digestive process breaks down proteins to their constituent amino acids, which enter the blood. The complete oxidation of proteins to CO2, H2O, and NH4+ in the body yields approximately 4 kcal/g (see Table 1.1).
FIGURE 1.5 General structure of proteins and amino acids. Each amino acid in this figure is indicated by a different color. R = side chain. Different amino acids have different side chains. For example, R1 might be –CH3; R2, –CH2OH; R3, –CH2–COO−. In a protein, the amino acids are linked by peptide bonds.
C. Fats
Fats are lipids composed of triacylglycerols (also called triglycerides). A triacylglycerol molecule contains three fatty acids esterified to one glycerol moiety (Fig. 1.6).
FIGURE 1.6 Structure of a triacylglycerol. Palmitate and stearate are saturated fatty acids—that is, they have no double bonds in the carbon chain. Oleate is monounsaturated (one double bond). Polyunsaturated fatty acids have more than one double bond.
Fats contain much less oxygen than is contained in carbohydrates or proteins. Therefore, fats are more reduced and yield more energy when oxidized. The complete oxidation of triacylglycerols to CO2 and H2O in the body releases approximately 9 kcal/g, more than twice the energy yield from an equivalent amount of carbohydrate or protein (see Table 1.1).
D. Alcohol
Alcohol (ethanol, in the context of the diet) has considerable caloric content. Ethanol (CH3CH2OH) is oxidized to CO2 and H2O in the body and yields approximately 7 kcal/g—that is, more than carbohydrate or protein but less than fat.
II. Body Fuel Stores
Humans carry supplies of fuel within their bodies (Table 1.2), which are similar to the fuel supplies in the plants and animals we eat. These fuel stores are light in weight, large in quantity, and readily converted into oxidizable substances. Most of us are familiar with fat, our major fuel store, which is located in adipose tissue. Although fat is distributed throughout our bodies, it tends to increase in quantity in our hips, thighs, and abdomens as we advance into middle age. In addition to our fat stores, we have important, although much smaller, stores of carbohydrates in the form of glycogen located primarily in our liver and muscles. Glycogen consists of glucose molecules joined together to form a large, branched polysaccharide (see Fig. 1.4). Body protein, particularly the protein of our large muscle masses, also serves to some extent as a fuel store, and we draw on it for energy when we fast.
TABLE 1.2 Fuel Composition of the Average 70 kg Mana after an Overnight Fast
FUEL | AMOUNT (KG) | PERCENTAGE OF TOTAL STORED CALORIES |
Glycogen | ||
Muscle | 0.15 | 0.4 |
Liver | 0.08 | 0.2 |
Protein | 6.0 | 14.4 |
Triglyceride | 15 | 85 |
aIn biochemistry and nutrition, the standard reference is often the 70-kg (154 lb) man. This standard was probably chosen because in the first half of the 20th century, when many nutritional studies were performed, young healthy medical and graduate students (who were mostly men) volunteered to serve as subjects for these experiments.
A. Fat
Our major fuel store is adipose triacylglycerol (triglyceride), a lipid more commonly known as fat. The average 70-kg man has approximately 15 kg of stored triacylglycerol, which accounts for approximately 85% of his total stored calories (see Table 1.2).
Two characteristics make adipose triacylglycerol a very efficient fuel store: the fact that triacylglycerol contains more calories per gram than carbohydrate or protein (9 kcal/g vs. 4 kcal/g) and the fact that adipose tissue does not contain much water. Adipose tissue contains only about 15% water, compared to tissues such as muscle that contain about 80%. Thus, the 70-kg man with 15 kg of stored triacylglycerol has only about 18 kg of adipose tissue.
B. Glycogen
Our stores of glycogen in liver, muscle, and other cells are relatively small in quantity but are nevertheless important. Liver glycogen is used to maintain blood glucose levels between meals, which is necessary for optimal functioning of the nervous system. Thus, the size of this glycogen store fluctuates during the day; an average 70-kg man might have 200 g or more of liver glycogen after a meal but only 80 g after an overnight fast. Muscle glycogen supplies energy for muscle contraction during exercise. At rest, the 70-kg man has approximately 150 g of muscle glycogen. Almost all cells, including neurons, maintain a small emergency supply of glucose as glycogen.
C. Protein
Protein serves many important roles in the body; unlike fat and glycogen, it is not solely a fuel store like fat or glycogen. Muscle protein is essential for body movement. Other proteins serve as enzymes (catalysts of biochemical reactions) or as structural components of cells and tissues. Only a limited amount of body protein can be degraded, approximately 6 kg in the average 70-kg man, before our body functions are compromised.
III. Daily Energy Expenditure
If we want to stay in energy balance, neither gaining nor losing weight, we must, on average, consume an amount of food equal to our daily energy expenditure (DEE). The daily energy expenditure includes the energy to support our basal metabolism (basal metabolic rate [BMR] or resting metabolic rate [RMR]) and our physical activity, plus the energy required to process the food we eat (diet-induced thermogenesis [DIT]). Thus, the DEE, in kilocalories per day = BMR (or RMR) + the energy needed for physical activity + DIT.
A. Basal Metabolic Rate
Two terms have been used to define the energy required by the body: the BMR and the RMR. The BMR is a measure of the energy required to maintain life: the functioning of the lungs, kidneys, and brain; the pumping of the heart; the maintenance of ionic gradients across membranes; and the reactions of biochemical pathways. The BMR was originally defined as the energy expenditure of a person mentally and bodily at rest in a thermoneutral environment 12 to 18 hours after a meal. However, when a person is awakened and their heat production or oxygen consumption is measured, they are no longer sleeping or totally at mental rest, and their metabolic rate is called the resting metabolic rate (RMR). It is also sometimes called the resting energy expenditure (REE). The RMR and BMR differ very little in value, and for the purposes of this text, we will focus on the BMR.
The BMR, which is usually expressed in kcal/day, is affected by body size, age, sex, and other factors (Table 1.3). It is proportional to the amount of metabolically active tissue (including the major organs) and the lean (or fat-free) body mass. Obviously, the amount of energy required for basal functions in a large person is greater than the amount required in a small person. However, the BMR is usually lower for women than for men of the same weight because women usually have more adipose tissue and less muscle mass. Body temperature also affects the BMR, which increases by 12% with each degree centigrade (7% with each degree Fahrenheit) increase in body temperature (i.e., “feed a fever; starve a cold”). The ambient temperature affects the BMR, which increases slightly in colder climates as thermogenesis is activated. Excessive secretion of thyroid hormone (hyperthyroidism) causes the BMR to increase, whereas diminished secretion (hypothyroidism) causes it to decrease. The BMR increases during pregnancy and lactation. Growing children have a higher BMR per kilogram body weight than adults because a greater proportion of their bodies is composed of brain, muscle, and other more metabolically active tissues. The BMR declines in aging individuals because their metabolically active tissue is shrinking and body fat is increasing. In addition, large variations exist in BMR from one adult to another, determined by genetic factors.
TABLE 1.3 Factors Affecting BMR Expressed per kg Body Weight
Gender (males higher than females) |
Body temperature (increased with fever) |
Environmental temperature (increased in cold) |
Thyroid status (increased in hyperthyroidism) |
Pregnancy and lactation (increased) |
Age (decreased with age) |
Body composition (increased with muscle mass) |
A rough estimate of the BMR may be obtained by assuming it is either 24 or 21.6 kcal/day/kg body weight (for men or women, respectively) and multiplying by the body weight. An easy way to remember this is 1 kcal/kg/hr for men and 0.9 kcal/kg/hr for women. This estimate works best for young individuals who are near their healthy weight. More accurate methods for calculating the BMR use empirically derived equations for different gender and age groups (Table 1.4). Even these calculations do not take into account variation among individuals.
B. Physical Activity
In addition to the BMR, the energy required for physical activity contributes to the DEE. The difference in physical activity between a student and a lumberjack is enormous, and a student who is relatively sedentary during the week may be much more active during the weekend. Table 1.5 gives factors for calculating the approximate energy expenditures associated with typical activities.
TABLE 1.5 Typical Activities with Corresponding Hourly Activity Factorsa
ACTIVITY CATEGORY | HOURLY ACTIVITY FACTOR (FOR TIME IN ACTIVITY) |
Resting: sleeping, reclining | 1.0 |
Very light: seated and standing activities, driving, laboratory work, typing, sewing, ironing, cooking, playing cards, playing a musical instrument | 1.5 |
Light: walking on a level surface at 2.5–3 mph, garage work, electrical trades, carpentry, restaurant trades, house cleaning, golf, sailing, table tennis | 2.5 |
Moderate: walking 3.5–4 mph, weeding and hoeing, carrying loads, cycling, skiing, tennis, dancing | 5.0 |
Heavy: walking uphill with a load, tree felling, heavy manual digging, mountain climbing, basketball, football, soccer | 7.0 |
aThe hourly activity factor is multiplied by the basal metabolic rate per hour times the number of hours engaged in the activity to give the caloric expenditure for that activity. If this is done for all of the hours in a day, the sum over 24 hours will approximately equal the daily energy expenditure.
National Research Council. Recommended Dietary Allowances. 10th ed. National Academy Press; 1989. https://doi.org/10.17226/1349. Adapted and reproduced with permission from the National Academy of Sciences. Courtesy of the National Academies Press, Washington, D.C.
A rough estimate of the energy required per day for physical activity can be made by using a value of 30% of the BMR (per day) for a very sedentary person (such as a medical student who does little but study) and a value of 60% to 70% of the BMR (per day) for a person who engages in about 2 hours of moderate physical activity per day (see Table 1.5). A value of 100% or more of the BMR is used for a person who does several hours of vigorous physical activity per day.
C. Diet-Induced Thermogenesis
Our DEE includes a component related to the intake of food known as diet-induced thermogenesis or the thermic effect of food (TEF). DIT was formerly called the specific dynamic action (SDA). After the ingestion of food, our metabolic rate increases because energy is required to digest, absorb, distribute, and store nutrients.
The energy required to process the types and quantities of food in the typical American diet is probably equal to approximately 10% of the kilocalories ingested. This amount is roughly equivalent to the error involved in rounding off the caloric content of carbohydrate, fat, and protein to 4, 9, and 4, respectively. Therefore, DIT is often ignored, and calculations are based simply on the BMR and the energy required for physical activity.
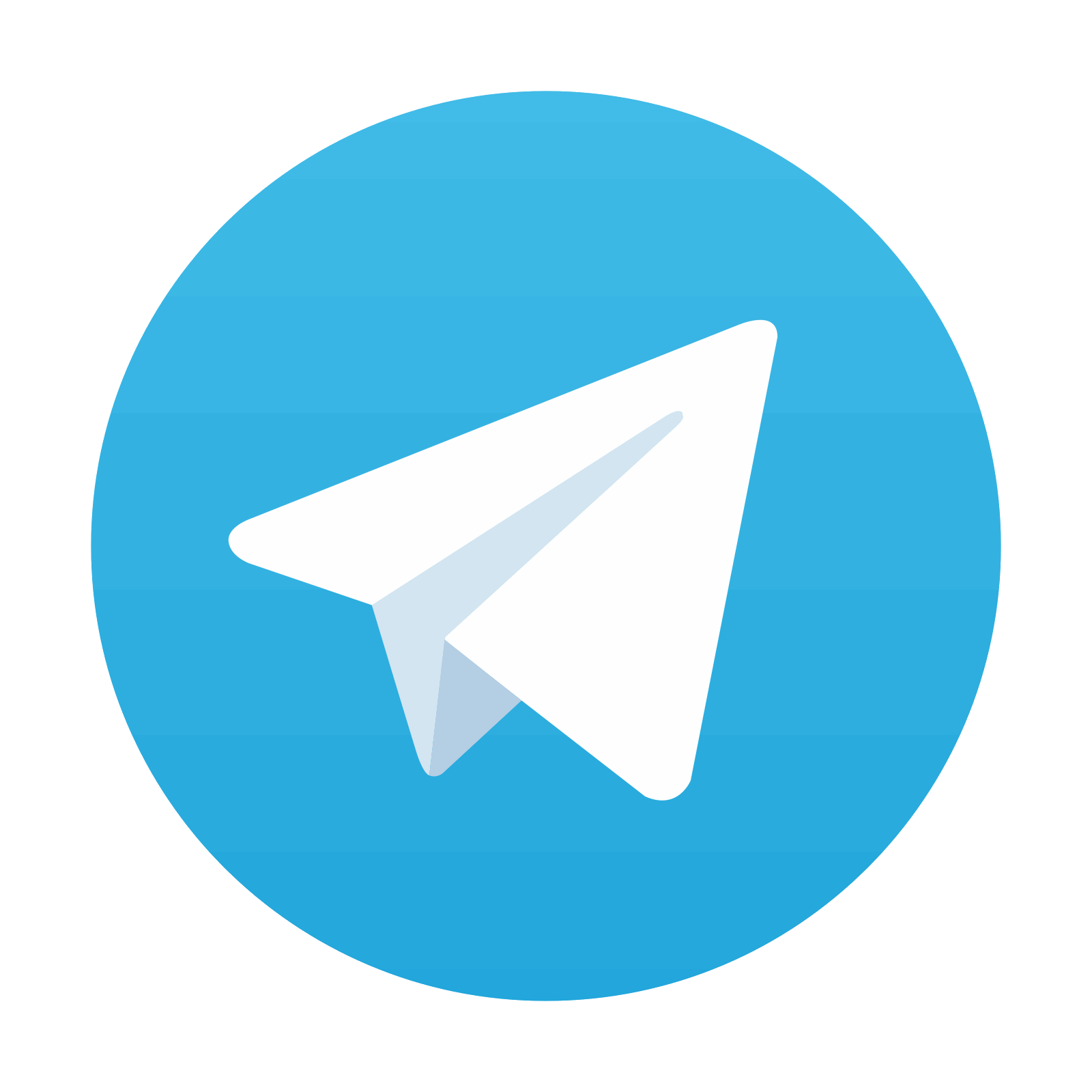
Stay updated, free articles. Join our Telegram channel

Full access? Get Clinical Tree
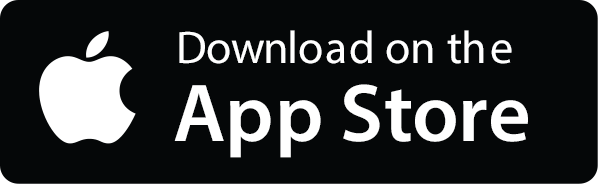
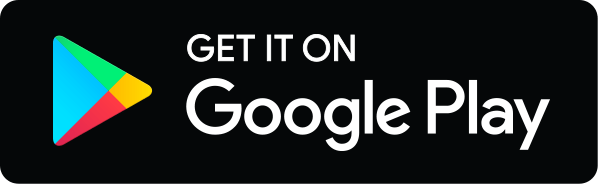