62
Major Histocompatibility Complex & Transplantation
CHAPTER CONTENTS
INTRODUCTION
The success of tissue and organ transplants depends on the donor’s and recipient’s human leukocyte antigens (HLA) encoded by the HLA genes. These proteins are alloantigens (i.e., they differ among members of the same species). If the HLA proteins on the donor’s cells differ from those on the recipient’s cells, then an immune response occurs in the recipient. The genes for the HLA proteins are clustered in the major histocompatibility complex (MHC), located on the short arm of chromosome 6. Three of these genes (HLA-A, HLA-B, and HLA-C) code for the class I MHC proteins. Several HLA-D loci determine the class II MHC proteins (i.e., DP, DQ, and DR) (Figure 62–1). The features of class I and class II MHC proteins are compared in Table 62–1.
FIGURE 62–1 The human leukocyte antigen (HLA)–gene complex. A, B, and C are class I loci. DP, DQ, and DR are class II loci. C2 and C4 are complement loci. LT, lymphotoxin; TNF, tumor necrosis factor. PGM3, GLO, and Pg5 are adjacent, unrelated genes. (Reproduced with permission from Stites DP, Terr A, Parslow T, eds. Basic & Clinical Immunology. 9th ed. Originally published by Appleton & Lange. Copyright 1997 McGraw-Hill.)
Each person has two haplotypes (i.e., two sets of these genes: one on the paternal and the other on the maternal chromosome 6). These genes are very diverse (polymorphic) (i.e., there are many alleles of the class I and class II genes). For example, there are at least 47 HLA-A genes, 88 HLA-B genes, 29 HLA-C genes, and more than 300 HLA-D genes, but any individual inherits only a single allele at each locus from each parent and thus can make no more than two class I and II proteins at each gene locus. Expression of these genes is codominant (i.e., the proteins encoded by both the paternal and maternal genes are produced). Each person can make as many as 12 different HLA proteins: 3 at class I loci and 3 at class II loci, from both chromosomes. A person can make fewer than 12 different HLA proteins if the person is homozygous at any of the 6 loci (i.e., if both parents have the same HLA allele).
In addition to the major antigens encoded by the HLA genes, there is an unknown number of minor antigens encoded by genes at sites other than the HLA locus. These minor antigens can induce a weak immune response that can result in slow rejection of a graft. The cumulative effect of several minor antigens can lead to a more rapid rejection response. These minor antigens are various normal body proteins that have one or more amino acid differences from one person to another (i.e., they are “allelic variants”). Because these proteins have an amino acid difference, they are immunogenic when introduced as part of the donor graft tissue. There are no laboratory tests for minor antigens.
Between the class I and class II gene loci is a third locus (Figure 62–1), sometimes called class III. This locus contains several immunologically important genes, encoding two cytokines (tumor necrosis factor and lymphotoxin) and two complement components (C2 and C4), but it does not have any genes that encode histocompatibility antigens.
MHC PROTEINS
Class I MHC Proteins
These are glycoproteins found on the surface of virtually all nucleated cells. There are approximately 20 different proteins encoded by the allelic genes at the A locus, 40 at the B locus, and 8 at the C locus. The complete class I protein is composed of a 45,000-molecular-weight heavy chain noncovalently bound to a β2-microglobulin. The heavy chain is highly polymorphic and is similar to an immunoglobulin molecule; it has hypervariable regions in its N-terminal region. The polymorphism of these molecules is important in the recognition of self and nonself. Stated another way, if these molecules were more similar, our ability to accept foreign grafts would be correspondingly improved. The heavy chain also has a constant region where the CD8 protein of the cytotoxic T cell binds.
Class II MHC Proteins
These are glycoproteins found on the surface of certain cells, including macrophages, B cells, dendritic cells of the spleen, and Langerhans’ cells of the skin. They are highly polymorphic glycoproteins composed of two polypeptides that are noncovalently bound. Like class I proteins, they have hypervariable regions that provide much of the polymorphism. Unlike class I proteins, which have only one chain encoded by the MHC locus (β2-microglobulin is encoded on chromosome 15), both chains of the class II proteins are encoded by the MHC locus. The two peptides also have a constant region where the CD4 proteins of the helper T cells bind.
BIOLOGIC IMPORTANCE OF MHC
The ability of T cells to recognize antigen is dependent on association of the antigen with either class I or class II proteins. For example, cytotoxic T cells respond to antigen in association with class I MHC proteins. Thus a cytotoxic T cell that kills a virus-infected cell will not kill a cell infected with the same virus if the cell does not also express the appropriate class I proteins. This finding was determined by mixing cytotoxic T cells bearing certain class I MHC proteins with virus-infected cells bearing different class I MHC proteins and observing that no killing of the virus-infected cells occurred. Helper T cells recognize class II proteins. Helper cell activity depends in general on both the recognition of the antigen on antigen-presenting cells and the presence on these cells of “self” class II MHC proteins. This requirement to recognize antigen in association with a “self” MHC protein is called MHC restriction. Note that T cells recognize antigens only when the antigens are presented on the surface of cells (in association with either class I or II MHC proteins), whereas B cells do not have that requirement and can recognize soluble antigens in plasma with their surface monomer IgM acting as the antigen receptor.
MHC genes and proteins are also important in two other medical contexts. One is that many autoimmune diseases occur in people who carry certain MHC genes (see Chapter 66), and the other is that the success of organ transplants is, in large part, determined by the compatibility of the MHC genes of the donor and recipient (see below).
TRANSPLANTATION
An autograft (transfer of an individual’s own tissue to another site in the body) is always permanently accepted (i.e., it always “takes”). A syngeneic graft1 is a transfer of tissue between genetically identical individuals (i.e., identical twins) and almost always “takes.” A xenograft,1 a transfer of tissue between different species, is always rejected by an immunocompetent recipient.
An allograft1 is a graft between genetically different members of the same species (e.g., from one human to another). Allografts are usually rejected unless the recipient is given immunosuppressive drugs. The severity and rapidity of the rejection will vary depending on the degree of the differences between the donor and the recipient at the MHC loci.
Allograft Rejection
Unless immunosuppressive measures are taken, allografts are rejected by a process called the allograft reaction. In an acute allograft reaction, vascularization of the graft is normal initially, but in 11 to 14 days, marked reduction in circulation and mononuclear cell infiltration occurs, with eventual necrosis. This is called a primary (first-set) reaction. A T-cell–mediated reaction is the main cause of rejection of many types of grafts (e.g., skin), but antibodies contribute to the rejection of certain transplants, especially bone marrow. In experimental animals, rejection of most types of grafts can be transferred by cells, not serum. Also, T-cell–deficient animals do not reject grafts, but B-cell–deficient animals do. The role of cytotoxic T cells in allograft rejection is described on page 497.
If a second allograft from the same donor is applied to a sensitized recipient, it is rejected in 5 to 6 days. This accelerated (second-set) reaction is caused primarily by presensitized cytotoxic T cells.
The acceptance or rejection of a transplant is determined, in large part, by the class I and class II MHC proteins on the donor cells, with class II playing the major role. The proteins encoded by the DR locus are especially important. These alloantigens activate T cells, both helper and cytotoxic, which bear T-cell receptors specific for the alloantigens. The activated T cells proliferate and then react against the alloantigens on the donor cells. CD8-positive cytotoxic T cells do most of the killing of the allograft cells.
Foreign MHC proteins typically activate many more T cells (i.e., they elicit a much stronger reaction) than do foreign proteins that are not MHC proteins. The strength of the response to foreign MHC proteins can be explained by the observation that there are three processes by which the recipient’s immune response is stimulated. These processes are as follows: (1) antigen-presenting cells (e.g., macrophages and dendritic cells) in the graft can present self (the donor’s) proteins in association with their class I and class II MHC proteins and activate the recipient’s immune response; (2) antigen-presenting cells in the graft can present the recipient’s proteins and activate the recipient’s immune response (because the recipient’s proteins are recognized as foreign when presented by a foreign MHC protein); and (3) the donor’s self proteins and class I and class II MHC proteins can be shed and subsequently processed by the recipient’s antigen-presenting cells, which activates the recipient’s immune response.
A graft that survives an acute allograft reaction can nevertheless become nonfunctional as a result of chronic rejection. This can occur months to years after engraftment. The main pathologic finding in grafts undergoing chronic rejection is atherosclerosis of the vascular endothelium. The immunologic cause of chronic rejection is unclear, but incompatibility of minor histocompatibility antigens and side effects of immunosuppressive drugs are likely to play a role.
In addition to acute and chronic rejection, a third type called hyperacute rejection can occur. Hyperacute rejection typically occurs within minutes of engraftment and is due to the reaction of preformed anti-ABO antibodies in the recipient with ABO antigens on the surface of the endothelium of the graft. Hyperacute rejection is often called the “white graft” reaction, because the graft turns white as a result of the loss of blood supply caused by spasm and occlusion of the vessels serving the graft. In view of this severe rejection reaction, the ABO blood group of donors and recipients must be matched and a cross-matching test (see later) must be done. The laboratory tests used to determine ABO blood groups are described in Chapter 64.
HLA Typing in the Laboratory
Prior to transplantation surgery, laboratory tests, commonly called HLA typing or tissue typing, are performed to determine the closest MHC match between the donor and the recipient.
There are two methods commonly used in the laboratory to determine the haplotype (i.e., the class I and class II alleles on both chromosomes) of both the potential donors and the recipient. One method is DNA sequencing using polymerase chain reaction (PCR) amplification and specific probes to detect the different alleles. This method is highly specific and sensitive and is the method of choice when available. The other method is serologic assays, in which cells from the donor and recipient are reacted with a battery of antibodies, each one of which is specific for a different class I and class II protein. Complement is then added, and any cell bearing an MHC protein homologous to the known antibody will lyse. This method is satisfactory in most instances but has failed to identify certain alleles that have been detected by DNA sequencing.
If sufficient data cannot be obtained by DNA sequencing or serologic assays, then additional information regarding the compatibility of the class II MHC proteins can be determined by using the mixed lymphocyte culture (MLC) technique. This test is also known as the mixed lymphocyte reaction (MLR). In this test, “stimulator” lymphocytes from a potential donor are first killed by irradiation and then mixed with live “responder” lymphocytes from the recipient; the mixture is incubated in cell culture to permit DNA synthesis, which is measured by incorporation of tritiated thymidine. The greater the amount of DNA synthesis in the responder cells, the more foreign are the class II MHC proteins of the donor cells. A large amount of DNA synthesis indicates an unsatisfactory “match” (i.e., donor and recipient class II [HLA-D] MHC proteins are not similar and the graft is likely to be rejected). Therefore, the best donor is the person whose cells stimulated the incorporation of the least amount of tritiated thymidine in the recipient cells.
In addition to the tests used for matching, preformed cytotoxic antibodies in the recipient’s serum reactive against the graft are detected by observing the lysis of donor lymphocytes by the recipient’s serum plus complement. This is called cross-matching and is done to prevent hyperacute rejections from occurring. The donor and recipient are also matched for the compatibility of their ABO blood groups. The laboratory tests used to determine ABO blood groups are described in Chapter 64.
Among siblings in a single family, there is a 25% chance for both haplotypes to be shared, a 50% chance for one haplotype to be shared, and a 25% chance for no haplotypes to be shared. For example, if the father is haplotype AB, the mother is CD, and the recipient child is AC, there is a 25% chance for a sibling to be AC (i.e., a two-haplotype match), a 50% chance for a sibling to be either BC or AD (i.e., a one-haplotype match), and a 25% chance for a sibling to be BD (i.e., a zero-haplotype match).
The Fetus is an Allograft that is Not Rejected
A fetus has MHC genes inherited from the father that are foreign to the mother, yet allograft rejection of the fetus does not occur. This is true despite many pregnancies from the same mother–father combination that produce offspring with the same MHC haplotypes. The reason that the mother fails to reject the fetus is unclear. The mother forms antibodies against the foreign paternal MHC proteins; therefore, the reason is not that the mother is not exposed to fetal antigens. One possible explanation is that the trophoblast layer of the placenta does not allow maternal T cells to enter the fetus.
Results of Organ Transplants
If the donor and recipient are well-matched by mixed-lymphocyte culture and histocompatibility antigen typing, the long-term survival of a transplanted organ or tissue is enhanced. Also, long-term survival is better if the donor is living rather than deceased. In 2010, the 5-year survival rate of kidney transplants from living donors, who are often family members and share at least one haplotype with the recipient, is approximately 90%, whereas the 5-year survival rate of kidney transplants from deceased donors (i.e., a zero-haplotype match) is approximately 80%. The 5-year survival rate of heart transplants that are from deceased donors (i.e., a zero-haplotype match) is approximately 75%. Corneal transplants have a very high rate of success for a different reason, namely, because corneas are avascular and the lymphatic supply of the eye prevents many antigens from triggering an immune response. Because corneal transplants elicit a weak rejection response, immunosuppression is usually minimal. In contrast, most other transplants require long-term immunosuppression, although the dose of immunosuppressive drugs typically decreases with time, and in some recipients, a state of tolerance ensues and the drugs can be stopped.
Graft-Versus-Host Reaction
Well-matched transplants of bone marrow may establish themselves initially in 85% of recipients, but subsequently a graft-versus-host (GVH) reaction develops in about two-thirds of the recipients.2
This reaction occurs because grafted immunocompetent T cells proliferate in the irradiated, immunocompromised host and reject host cells with “foreign” proteins, resulting in severe organ dysfunction. The donor’s cytotoxic T cells play a major role in destroying the recipient’s cells. Among the main symptoms are maculopapular rash, jaundice, hepatosplenomegaly, and diarrhea. Many GVH reactions end in overwhelming infections and death.
There are three requirements for a GVH reaction to occur: (1) the graft must contain immunocompetent T cells, (2) the host must be immunocompromised, and (3) the recipient must express antigens (e.g., MHC proteins) foreign to the donor (i.e., the donor T cells recognize the recipient cells as foreign). Note that even when donor and recipient have identical class I and class II MHC proteins (i.e., identical haplotypes), a GVH reaction can occur because it can be elicited by differences in minor antigens. The GVH reaction can be reduced by treating the donor tissue with antithymocyte globulin or monoclonal antibodies before grafting; this eliminates mature T cells from the graft. Cyclosporine (see later) is also used to reduce the GVH reaction.
EFFECT OF IMMUNOSUPPRESSION ON GRAFT REJECTION
To reduce the rejection of transplanted tissue, immunosuppressive measures (e.g., cyclosporine, tacrolimus [FK506, Prograf], sirolimus [rapamycin, Rapamune], corticosteroids, azathioprine, monoclonal antibodies, belatacept, and radiation) are used. Cyclosporine prevents the activation of T lymphocytes by inhibiting the synthesis of interleukin (IL)-2 and IL-2 receptor. It does so by inhibiting calcineurin—a protein (a serine phosphatase) involved in the activation of transcription of the genes for IL-2 and the IL-2 receptor. Cyclosporine is well-tolerated and is remarkably successful in preventing the rejection of transplants. Cyclosporine and tacrolimus have the same mode of action; tacrolimus is more immunosuppressive but causes more side effects. Rapamycin also inhibits signal transduction but at a site different from that of cyclosporine and tacrolimus.
Corticosteroids act primarily by inhibiting cytokine (e.g., IL-1 and tumor necrosis factor) production by macrophages and by lysing certain types of T cells. Corticosteroids inhibit cytokine production by blocking transcription factors, such as NFκB and AP-1, which prevents the mRNA for these cytokines from being synthesized. Azathioprine is an inhibitor of DNA synthesis and blocks the growth of T cells. Mycophenolate mofetil also inhibits DNA synthesis and has fewer side effects than azathioprine. However, some recipients of mycophenolate have contracted progressive multifocal leukoencephalopathy—an often fatal disease (see Chapter 44).
Monoclonal antibodies are used in immunosuppressive regimens, both to prevent rejection and to treat rejection episodes. Muromonab (OKT3) is a monoclonal antibody against CD3, and basiliximab (Simulect) is a monoclonal antibody against the IL-2 receptor. Table 62–2 describes these monoclonal antibodies as well as others in clinical use.
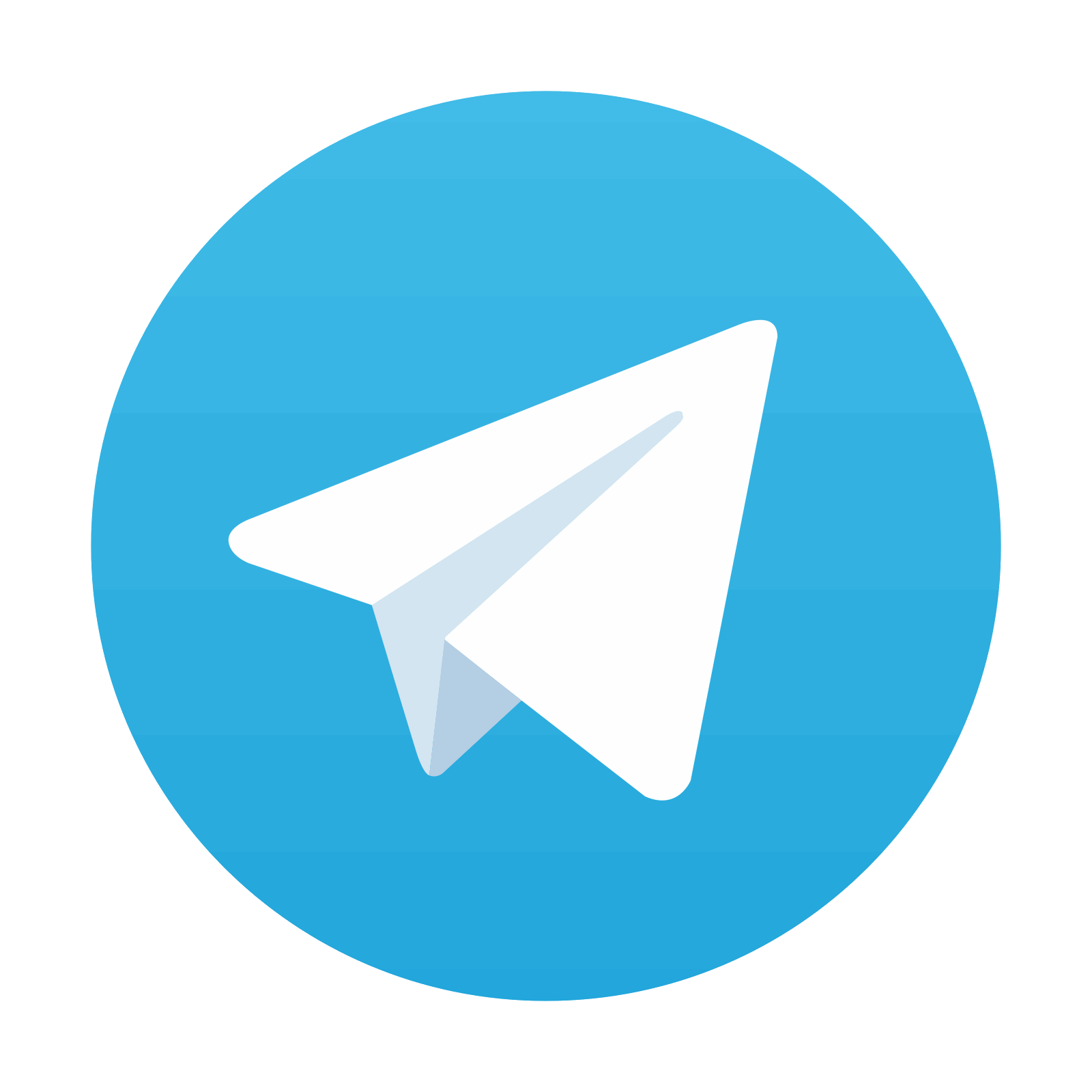
Stay updated, free articles. Join our Telegram channel

Full access? Get Clinical Tree
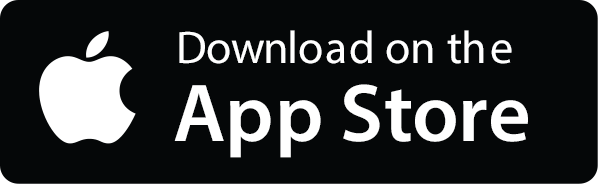
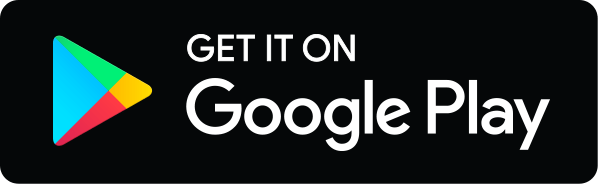