56
Introduction to Toxicology: Occupational & Environmental
Humans live in a chemical world. They inhale, ingest, and absorb through the skin many of these chemicals. The occupational-environmental toxicologist is primarily concerned with adverse effects in humans resulting from exposure to chemicals encountered at work or in the general environment. In clinical practice, the occupational-environmental toxicologist must identify and treat the adverse health effects of these exposures. In addition, the trained occupational-environmental toxicologist will be called upon to assess and identify hazards associated with chemicals used in the workplace or introduced into the human environment.
Occupational and environmental toxicology cases present unusually complex problems. Occupational and environmental exposure is rarely limited to a single type of molecule. Most workplace or environmental materials are compounds or mixtures, and the ingredients are often poorly described in the documentation that is available for physician review. Moreover, although regulatory agencies in many countries have requirements for disclosure of hazardous materials and their health impacts, proprietary information exclusions often make it difficult for those who treat occupationally and environmentally poisoned patients to understand the nature and scope of the presenting illness. Because many of these illnesses have long latency periods before they become manifest, it is often a matter of detective work, when patients finally present with disease, to ascertain exposure and relate it to clinical effect. Monitoring of exposure concentrations both in the workplace and in the general environment has become more common, but it is far from widespread, and so it is often very difficult to establish the extent of exposure, its duration, and its dose rate when this information is critical to the identification of the toxic disorder and its management.
Occupational Toxicology
Occupational toxicology deals with the chemicals found in the workplace. The major emphasis of occupational toxicology is to identify the agents of concern, identify the acute and chronic diseases that they cause, define the conditions under which they may be used safely, and prevent absorption of harmful amounts of these chemicals. The occupational toxicologist will also be called upon to treat the diseases caused by these chemicals if he or she is a physician. Occupational toxicologists may also define and carry out programs for the surveillance of exposed workers and the environment in which they work. They frequently work hand in hand with occupational hygienists, certified safety professionals, and occupational health nurses in their activities.
Regulatory limits and voluntary guidelines have been elaborated to establish safe ambient air concentrations for many chemicals found in the workplace. Governmental and supragovernmental bodies throughout the world have generated workplace health and safety rules, including short- and long-term exposure limits for workers. These permissible exposure limits (PELs) have the power of law in the United States. Copies of the U.S. Occupational Safety and Health Administration (OSHA) standards may be found on OSHA’s website at http://www.osha.gov. Copies of the U.S. Mine Safety and Health Administration (MSHA) standards may be found at http://www.msha.gov. In addition to the PELs that appear in the OSHA publications and on the website, OSHA promulgates standards for specific materials of particularly serious toxicity. These standards are developed following extensive scientific study, stakeholder input at hearings, public comment, and other steps such as publication in the Federal Register. Such standards have the force of law and employers who use these materials are obligated to comply with the standards. OSHA standards may be found in full on the OSHA website at http://www.osha.gov.
Voluntary organizations, such as the American Conference of Governmental Industrial Hygienists (ACGIH), periodically prepare lists of recommended threshold limit values (TLVs) for many chemicals. These guidelines are periodically updated. Regulatory imperatives in the United States may also be updated from time to time when new information about toxicity becomes available. However, this process is slow and requires input from many sources except under certain extraordinary circumstances. In those cases, emergency alterations to standards may be made and an emergency temporary standard may be promulgated after appropriate regulatory procedures. The ACGIH TLV guidelines are useful as reference points in the evaluation of potential workplace exposures. Compliance with these voluntary guidelines is not a substitute for compliance with the OSHA requirements in the United States. TLVs do not have the force of law. Current TLV lists may be obtained from the ACGIH at http://www.acgih.org.
Environmental Toxicology
Environmental toxicology deals with the potentially deleterious impact of chemicals, present as pollutants of the environment, on living organisms. The term environment includes all the surroundings of an individual organism, but particularly the air, soil, and water. Although humans are considered a target species of particular interest, other species are of considerable importance as potential biologic targets. Scientific study of signal occurrences in animals often provides early warning of impending human events as a result of ecotoxic impacts.
Air pollution is usually a product of industrialization, technologic development, and increased urbanization. On rare occasions, natural phenomena such as volcanic eruptions may result in air pollution with gases, vapors, or particulates that are harmful to humans. Humans may also be exposed to chemicals used in the agricultural environment as pesticides or in food processing that may persist as residues or ingredients in food products. Air contaminants are regulated in the United States by the Environmental Protection Agency (EPA) based on both health and esthetic considerations. Tables of primary and secondary regulated air contaminants and other regulatory issues that relate to air contaminants in the United States may be found at http://www.epa.gov. Many states within the USA also have individual air contaminant regulations that may be more rigorous than those of the EPA. Many other nations and some supragovernmental organizations regulate air contaminants. In the case of adjoining countries, transborder air and water pollution problems have been of concern in recent years. Particulates, radionuclides, acid rain, and similar problems have resulted in cross-contamination of air and water in different countries. Maritime contamination, too, has raised concern about oceanic pollution and has had an impact on the fisheries of some countries. This type of pollution is now the subject of much research and of new international treaties.
The United Nations Food and Agriculture Organization and the World Health Organization (FAO/WHO) Joint Expert Commission on Food Additives adopted the term acceptable daily intake (ADI) to denote the daily intake of a chemical from food that, during an entire lifetime, appears to be without appreciable risk. These guidelines are reevaluated as new information becomes available. In the United States, the FDA and the Department of Agriculture are responsible for the regulation of contaminants such as pesticides, drugs, and chemicals in foods. Major international problems have occurred because of traffic among nations in contaminated or adulterated foods from countries whose regulations and enforcement of pure food and drug laws are lax or nonexistent. Recently, for example, both human and animal illnesses have resulted from ingestion of products imported from China that contained melamine.
Ecotoxicology
Ecotoxicology is concerned with the toxic effects of chemical and physical agents on populations and communities of living organisms within defined ecosystems; it includes the transfer pathways of those agents and their interactions with the environment. Traditional toxicology is concerned with toxic effects on individual organisms; ecotoxicology is concerned with the impact on populations of living organisms or on ecosystems. Ecotoxicology research has become one of the foremost areas of study for toxicologists.
TOXICOLOGIC TERMS & DEFINITIONS
Hazard & Risk
Hazard is the ability of a chemical agent to cause injury in a given situation or setting; the conditions of use and exposure are primary considerations. To assess hazard, one needs to have knowledge about both the inherent toxicity of the substance and the amounts to which individuals are liable to be exposed. Hazard is often a description based on subjective estimates rather than objective evaluation.
Risk is defined as the expected frequency of the occurrence of an undesirable effect arising from exposure to a chemical or physical agent. Estimation of risk makes use of dose-response data and extrapolation from the observed relationships to the expected responses at doses occurring in actual exposure situations. The quality and suitability of the biologic data used in such estimates are major limiting factors. Risk assessment has become an integral part of the regulatory process in most countries. However, many of the assumptions of risk assessment scientists remain unproven, and only long-term observation of population causes and outcomes will provide the basis for validation of newer risk assessment technologies.
Routes of Exposure
The route of entry for chemicals into the body differs in different exposure situations. In the industrial setting, inhalation is the major route of entry. The transdermal route is also quite important, but oral ingestion is a relatively minor route. Consequently, primary prevention should be designed to reduce or eliminate absorption by inhalation or by topical contact. Atmospheric pollutants gain entry by inhalation and by dermal contact. Water and soil pollutants are absorbed through inhalation, ingestion, and dermal contact.
Quantity, Duration, & Intensity of Exposure
Toxic reactions may differ depending on the quantity of exposure, its duration, and the rate at which the exposure takes place. An exposure to a toxic substance that is absorbed by the target human or animal results in a dose. A single exposure, or multiple exposures that occur over a brief period from seconds to 1-2 days, represents acute exposure. Intense, rapidly absorbed acute doses of substances that may ordinarily be detoxified by enzymatic mechanisms in small doses may overwhelm the body’s ability to detoxify the substance and may result in serious or even fatal toxicity. The same amount of the substance, absorbed slowly, may result in little or no toxicity. This is the case with cyanide exposure. Rhodanese, a mitochondrial enzyme present in humans, effectively detoxifies cyanide to relatively nontoxic thiocyanate when cyanide is presented in small amounts, but the enzyme is overwhelmed by large, rapidly encountered cyanide doses, with lethal effect.
Single or multiple exposures over a longer period of time represent chronic exposure. In the occupational setting, both acute (eg, accidental discharge) and chronic (eg, repetitive handling of a chemical) exposures occur. Exposures to chemicals found in the environment such as air and water pollutants are often chronic, resulting in chronic disease, as in the Minamata Bay, Japan, methyl mercury disaster. Sudden large chemical releases may result in acute massive population exposure with serious or lethal consequences. The tragedy in Bhopal, India, was such an event, in which methyl isocyanate was released into a crowded population area, resulting in almost 4000 deaths and more than half a million injuries. The release of dioxin in Seveso, Italy, contaminated a populated area with a persistent organic chemical having both acute and long-term chronic effects. More recently, the massive oil spill caused by the explosion of BP’s Deepwater Horizon drilling rig in the Gulf of Mexico highlighted the potential for long-term ecotoxic impacts involving widespread geographic areas.
ENVIRONMENTAL CONSIDERATIONS
Certain chemical and physical characteristics are important for the estimation of the potential hazard of environmental toxicants. Data on toxic effects of different organisms, along with knowledge about degradability, bioaccumulation, and transport and biomagnification through food chains, help in this estimation. (See Box: Bioaccumulation & Biomagnification, for a classic example involving the Great Lakes.) Poorly degraded chemicals (by abiotic or biotic pathways) exhibit environmental persistence and can accumulate. Such chemicals include the persistent organic pollutants (POPs), polychlorinated biphenyls, dioxins and furans, and similar substances. Lipophilic substances such as the largely banned or abandoned organochlorine pesticides tend to bioaccumulate in body fat. This results in tissue residues that are slowly released over time. These residues and their metabolites may have chronic adverse effects such as endocrine disruption. When the toxicant is incorporated into the food chain, biomagnification occurs as one species feeds on others. This concentrates the chemical in organisms higher on the food chain. Humans stand at the apex of the food chain. They may be exposed to highly concentrated pollutant loads as bioaccumulation and biomagnification occur. The pollutants that have the widest environmental impact are poorly degradable; are relatively mobile in air, water, and soil; exhibit bioaccumulation; and also exhibit biomagnification.
SPECIFIC CHEMICALS
AIR POLLUTANTS
Air pollution may result from vapors, aerosols, smokes, particulates, and individual chemicals. Five major substances have been said to account for about 98% of air pollution: carbon monoxide (about 52%); sulfur oxides (about 14%); hydrocarbons (about 14%); nitrogen oxides (about 14%) and ozone, their breakdown product; and particulate matter (about 4%). Agriculture, especially industrial-scale farming, contributes a variety of air pollutants: dusts as particulates, pesticidal chemicals, hydrogen sulfide, and others. Sources of pollutants include fossil fuel burning, transportation, manufacturing, other industrial activities, generation of electric power, space heating, refuse disposal, and others. Studies in Helsinki and other cities have shown that uncatalyzed automobile traffic emissions are larger contributors to ground-level air pollution than any other source. The introduction of catalytic converters on automobiles and their mandatory use in many countries has greatly reduced automobile-released air pollution. In addition, the ban on tetraethyl lead in gasoline has eliminated a major source of lead contamination and childhood lead poisoning in urban environments. In emerging economies, the use of transport based on two-cycle engines creates heavy ground-level air pollution in very crowded cities. The introduction of “clean, low-sulfur” diesel fuels is helping to reduce urban and highway pollutants such as sulfur oxides.
If the intake of a long-lasting contaminant by an organism exceeds the latter’s ability to metabolize or excrete the substance, the chemical accumulates within the tissues of the organism. This is called bioaccumulation.
Although the concentration of a contaminant may be virtually undetectable in water, it may be magnified hundreds or thousands of times as the contaminant passes up the food chain. This is called biomagnification.
The biomagnification of polychlorinated biphenyls (PCBs) in the Great Lakes of North America is illustrated by the following residue values available from a classic Environment Canada report published by the Canadian government, and elsewhere.
The biomagnification for this substance in the food chain, beginning with phytoplankton and ending with the herring gull, is nearly 50,000-fold. Domestic animals and humans may eat fish from the Great Lakes, resulting in PCB residues in these species as well.
Sulfur dioxide and smoke from incomplete combustion of coal have been associated with acute adverse effects among children, the elderly, and individuals with preexisting cardiac or respiratory disease. Ambient air pollution has been implicated as a cause of cardiac disease, bronchitis, obstructive ventilatory disease, pulmonary emphysema, bronchial asthma, and airway or lung cancer. Extensive basic science and clinical epidemiologic literature on air pollutant toxicology has been published and has led to modifications of regulatory standards for air pollutants. EPA standards for these substances apply to the general environment, and OSHA standards apply to workplace exposure. Ambient air standards for carbon monoxide and five other harmful pollutants—particulate matter, nitrogen dioxide, ozone, sulfur dioxide, and lead—may be found at http://www.epa.gov/air/criteria.html.
Carbon Monoxide
Carbon monoxide (CO) is a colorless, tasteless, odorless, and non-irritating gas, a byproduct of incomplete combustion. The average concentration of CO in the atmosphere is about 0.1 ppm; in heavy traffic, the concentration may exceed 100 ppm. Current recommended permissible exposure limit (PEL) values are shown in Table 56–1 (see also http://www.osha.gov, 1910.1000, Table Z-1).
TABLE 56–1 Examples of permissible exposure limit values (PELs) of some common air pollutants and solvents in parts per million (ppm).1
1. Mechanism of action—CO combines tightly but reversibly with the oxygen-binding sites of hemoglobin and has an affinity for hemoglobin that is about 220 times that of oxygen. The product formed—carboxyhemoglobin—cannot transport oxygen. Furthermore, the presence of carboxyhemoglobin interferes with the dissociation of oxygen from the remaining oxyhemoglobin as a result of the Bohr effect. This reduces the transfer of oxygen to tissues. Organs with the highest oxygen demand (the brain, heart, and kidneys) are most seriously affected. Normal nonsmoking adults have carboxyhemoglobin levels of less than 1% saturation (1% of total hemoglobin is in the form of carboxyhemoglobin); this has been attributed to the endogenous formation of CO from heme catabolism. Smokers may exhibit 5–10% CO saturation. The level depends on their smoking habits. A person who breathes air that contains 0.1% CO (1000 ppm) would have a carboxyhemoglobin level of about 50% in a short period of time.
2. Clinical effects—The principal signs of CO intoxication are those of hypoxia. They progress in the following sequence: (1) psychomotor impairment; (2) headache and tightness in the temporal area; (3) confusion and loss of visual acuity; (4) tachycardia, tachypnea, syncope, and coma; and (5) deep coma, convulsions, shock, and respiratory failure. There is great variability in individual responses to carboxyhemoglobin concentration. Carboxyhemoglobin levels below 15% may produce headache and malaise; at 25% many workers complain of headache, fatigue, decreased attention span, and loss of fine motor coordination. Collapse and syncope may appear at around 40%; and with levels above 60%, death may ensue as a result of irreversible damage to the brain and myocardium. The clinical effects may be aggravated by heavy labor, high altitudes, and high ambient temperatures. CO intoxication is usually thought of as a form of acute toxicity. There is evidence that chronic exposure to low CO levels may lead to adverse cardiac effects, neurologic disturbance, and emotional disorders. The developing fetus is quite susceptible to the effects of CO exposure. Exposure of a pregnant woman to elevated CO levels at critical periods of fetal development may cause fetal death or serious and irreversible but survivable birth defects.
3. Treatment—Patients who have been exposed to CO must be removed from the exposure source immediately. Respiration must be maintained and high flow and concentration of oxygen—the specific antagonist to CO—should be administered promptly. If respiratory failure is present, mechanical ventilation is required, High concentrations of oxygen may be toxic and may contribute to the development of acute respiratory distress syndrome. Therefore, patients should be treated with high concentrations only for a short period. With room air at 1 atm, the elimination half-time of CO is about 320 minutes; with 100% oxygen, the half-time is about 80 minutes; and with hyperbaric oxygen (2–3 atm), the half-time can be reduced to about 20 minutes. Although some controversy exists about hyperbaric oxygen for CO poisoning, it may be used if it is readily available. It is particularly recommended for the management of pregnant women exposed to CO. Hypothermic therapy to reduce metabolic demand of the brain has also been useful. Cerebral edema that results from CO poisoning does not seem to respond to either mannitol or steroid therapy and may be persistent. Progressive recovery from treated CO poisoning, even of a severe degree can be complete but some patients manifest neuropsychological and motor dysfunction for a long time after recovery from acute CO poisoning.
Sulfur Dioxide
Sulfur dioxide (SO2) is a colorless irritant gas generated primarily by the combustion of sulfur-containing fossil fuels. The current OSHA PEL (Table 56–1) is given on the OSHA website (see http://www.osha.gov, 1910.1000, Table Z-1).
1. Mechanism of action—At room temperature, the solubility of SO2 is approximately 200 g SO2/L of water. Because of its high solubility, when SO2 contacts moist membranes, it transiently forms sulfurous acid. This acid has severe irritant effects on the eyes, mucous membranes, and skin. Approximately 90% of inhaled SO2 is absorbed in the upper respiratory tract, the site of its principal effect. The inhalation of SO2 causes bronchial constriction and produces profuse bronchorrhea; parasympathetic reflexes and altered smooth muscle tone appear to be involved. The clinical outcome is an acute irritant asthma. Exposure to 5 ppm SO2 for 10 minutes leads to increased resistance to airflow in most humans. Exposures of 5–10 ppm are reported to cause severe bronchospasm; 10–20% of the healthy young adult population is estimated to be reactive to even lower concentrations. The phenomenon of adaptation to irritating concentrations has been reported in workers. However, current studies have not confirmed this phenomenon. Asthmatic individuals are especially sensitive to SO2.
2. Clinical effects and treatment—The signs and symptoms of intoxication include irritation of the eyes, nose, and throat, reflex bronchoconstriction, and increased bronchial secretions. In asthmatic subjects, exposure to SO2 may result in an acute asthmatic episode. If severe exposure has occurred, delayed-onset pulmonary edema may be observed. Cumulative effects from chronic low-level exposure to SO2 are not striking, particularly in humans, but these effects have been associated with aggravation of chronic cardiopulmonary disease. When combined exposure to high respirable particulate loads and SO2 occurs, the mixed irritant load may increase the toxic respiratory response. Treatment is not specific for SO2 but depends on therapeutic maneuvers used to treat irritation of the respiratory tract and asthma. In some severely polluted urban air basins, elevated SO2 concentrations combined with elevated particulate loads has led to air pollution emergencies and a marked increase in cases of acute asthmatic bronchitis. Children and the elderly seem to be at greatest risk. The principal source of urban SO2 is the burning of coal, both for domestic heating and in coal-fired power plants. High-sulfur transportation fuels also contribute. Both also contribute to the respirable fine particulate load and to increased urban cardiorespiratory morbidity and mortality.
Nitrogen Oxides
Nitrogen dioxide (NO2) is a brownish irritant gas sometimes associated with fires. It is formed also from fresh silage; exposure of farmers to NO2 in the confines of a silo can lead to silo-filler’s disease, a severe and potentially lethal form of acute respiratory distress syndrome. The disorder is uncommon today. Miners who are regularly exposed to diesel equipment exhaust have been particularly affected by nitrogen oxide emissions with serious respiratory effects. Today, the most common source of human exposure to oxides of nitrogen, including NO2, is automobile and truck traffic emissions. Recent air pollution inventories in cities with high traffic congestion have demonstrated the important role that internal combustion engines have in the increasing NO2 urban air pollution. A variety of disorders of the respiratory system, cardiovascular system, and other problems have been linked to NO2 exposure.
1. Mechanism of action—NO2 is a relatively insoluble deep lung irritant. It is capable of producing pulmonary edema and acute adult respiratory distress syndrome (ARDS). Inhalation damages the lung infrastructure that produces the surfactant necessary to allow smooth and low effort lung alveolar expansion. The type I cells of the alveoli appear to be the cells chiefly affected by acute low to moderate inhalation exposure. At higher exposure, both type I and type II alveolar cells are damaged. If only type I cells are damaged, after an acute period of severe distress, it is likely that treatment with modern ventilation equipment and medications will result in recovery. Some patients develop nonallergic asthma, or “twitchy airway” disease, after such a respiratory insult. If severe damage to the type I and type II alveolar cells occurs, replacement of the type I cells may be impaired; progressive fibrosis may ensue that eventually leads to bronchial ablation and alveolar collapse. This can result in permanent restrictive respiratory disease. In addition to the direct deep lung effect, long-term exposure to lower concentrations of nitrogen dioxide has been linked to cardiovascular disease, increased incidence of stroke, and other chronic disease.
The current PEL for NO2 is given in Table 56–1. Exposure to 25 ppm of NO2 is irritating to some individuals; 50 ppm is moderately irritating to the eyes and nose. Exposure for 1 hour to 50 ppm can cause pulmonary edema and perhaps subacute or chronic pulmonary lesions; 100 ppm can cause pulmonary edema and death.
2. Clinical effects—The signs and symptoms of acute exposure to NO2 include irritation of the eyes and nose, cough, mucoid or frothy sputum production, dyspnea, and chest pain. Pulmonary edema may appear within 1–2 hours. In some individuals, the clinical signs may subside in about 2 weeks; the patient may then pass into a second stage of abruptly increasing severity, including recurring pulmonary edema and fibrotic destruction of terminal bronchioles (bronchiolitis obliterans). Chronic exposure of laboratory animals to 10–25 ppm NO2 has resulted in emphysematous changes; thus, chronic effects in humans are of concern.
3. Treatment—There is no specific treatment for acute intoxication by NO2; therapeutic measures for the management of deep lung irritation and noncardiogenic pulmonary edema are used. These measures include maintenance of gas exchange with adequate oxygenation and alveolar ventilation. Drug therapy may include bronchodilators, sedatives, and antibiotics. New approaches to the management of NO2-induced ARDS have been developed and considerable controversy now exists about the precise respiratory protocol to use in any given patient.
Ozone & Other Oxides
Ozone (O3) is a bluish irritant gas found in the earth’s atmosphere, where it is an important absorbent of ultraviolet light at high altitude. At ground level, ozone is an important pollutant. Atmospheric ozone pollution is derived from photolysis of oxides of nitrogen, volatile organic compounds, and CO. These compounds are produced primarily when fossil fuels such as gasoline, oil, or coal are burned or when some chemicals (eg, solvents) evaporate. Nitrogen oxides are emitted from power plants, motor vehicles, and other sources of high-heat combustion. Volatile organic compounds are emitted from motor vehicles, chemical plants, refineries, factories, gas stations, paint, and other sources. An EPA fact sheet on ground-level ozone, its sources, and consequences may be found at http://www.epa.gov/glo/.
Ozone can be generated in the workplace by high-voltage electrical equipment, and around ozone-producing devices used for air and water purification. Agricultural sources of ozone are also important. There is a near-linear gradient between exposure to ozone (1-hour level, 20–100 ppb) and bronchial smooth muscle response. See Table 56–1 for the current PEL for ozone.
1. Mechanism of action and clinical effects—
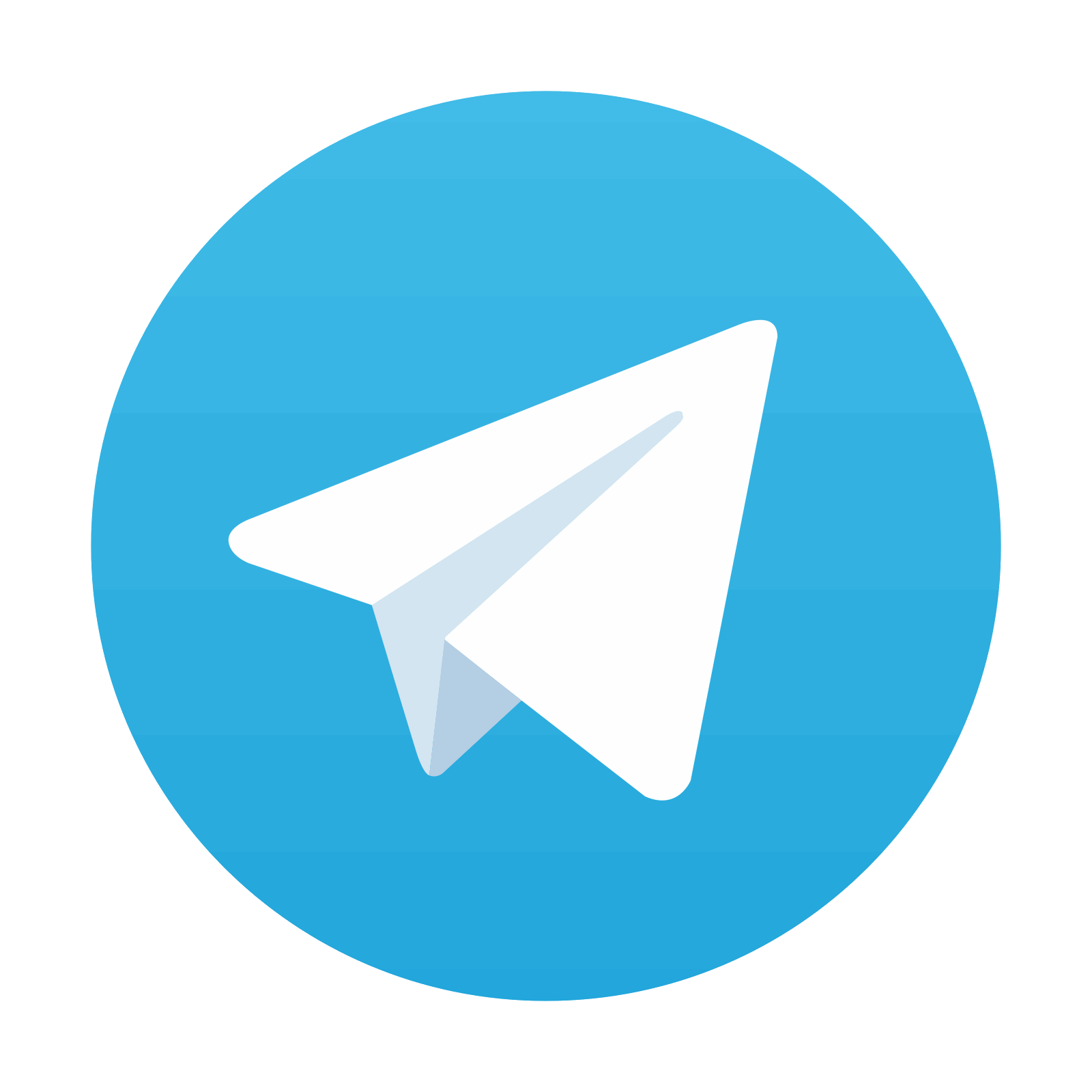
Stay updated, free articles. Join our Telegram channel

Full access? Get Clinical Tree
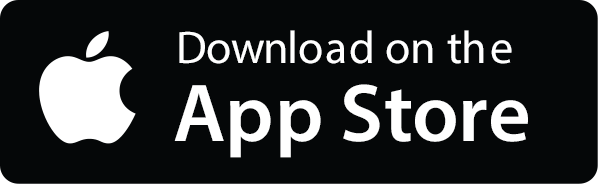
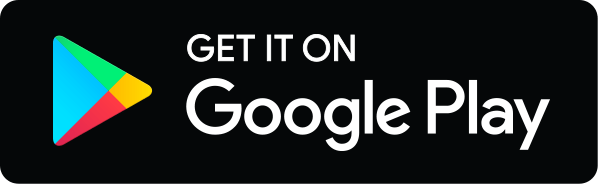