20
Drugs Used in Asthma
CASE STUDY
A 10-year-old girl with a history of poorly controlled asthma presents to the emergency department with severe shortness of breath and audible inspiratory and expiratory wheezing. She is pale, refuses to lie down, and appears extremely frightened. Her pulse is 120 bpm and respirations 32/min. Her mother states that the girl has just recovered from a mild case of flu and had seemed comfortable until this afternoon. The girl uses an inhaler (albuterol) but “only when really needed” because her parents are afraid that she will become too dependent on medication. She administered two puffs from her inhaler just before coming to the hospital, but “the inhaler doesn’t seem to have helped.” What emergency measures are indicated? How should her long-term management be altered?
A consistent increase in the prevalence of asthma over the past 60 years has made it an extraordinarily common disease. The reasons for this increase—shared across all modern, “westernized” societies—are poorly understood, but in the United States alone, 18.9 million adults and 7.1 million children currently have asthma. The condition accounts for 15 million outpatient visits, 1.8 million emergency department visits, and 440,000 hospitalizations each year. Despite substantial improvements in the treatment for the disease, asthma still accounts for 3400 deaths per year in the USA.
The clinical features of asthma are recurrent bouts of shortness of breath, chest tightness, and wheezing, often associated with coughing. Its hallmark physiologic features are widespread, reversible narrowing of the bronchial airways and a marked increase in bronchial responsiveness to inhaled stimuli; and its pathologic features are lymphocytic, eosinophilic inflammation of the bronchial mucosa. These changes are accompanied by “remodeling” of the bronchial wall, with thickening of the lamina reticularis beneath the epithelium and hyperplasia of the bronchial vasculature, smooth muscle, secretory glands, and goblet cells.
In mild asthma, symptoms occur only intermittently, as on exposure to allergens or air pollutants, on exercise, or after viral upper respiratory infection. More severe forms of asthma are associated with more frequent and severe symptoms, especially at night. Chronic airway constriction causes persistent respiratory impairment, punctuated by frequent acute asthmatic attacks, or “asthma exacerbations.” These attacks are most often associated with viral respiratory infections and are characterized by severe airflow obstruction from intense contraction of airway smooth muscle, inspissation of mucus plugs in the airway lumen, and thickening of the bronchial mucosa from edema and inflammatory cell infiltration. The spectrum of asthma’s severity is wide, and patients are classified as having “mild intermittent,” “mild persistent,” “moderate persistent,” and “severe persistent,” either based on the frequency and severity of symptoms and the severity of airflow obstruction on pulmonary function testing or by the minimal medical therapy required to keep their asthma well-controlled, and as “exacerbation-prone” or “exacerbation-resistant” based on the frequency of asthma exacerbations.
Until recently, the entire range of asthma severity was regarded as eminently treatable, because treatments for quick relief of symptoms of acute bronchoconstriction (“short-term relievers”) and treatments for reduction in symptoms and prevention of attacks (“long-term controllers”) have been shown effective in many large, well-designed clinical trials, case-control studies, and evidence-based reviews. The persistence of high medical costs for asthma, driven largely by the costs of emergency department and hospital treatment of asthma exacerbations, was thus believed to reflect underutilization of the treatments available. Reconsideration of this view was driven by recognition that the term “asthma” is applied to a variety of different disorders sharing common clinical features but fundamentally different pathophysiologic mechanisms. Attention has thus turned to the possibility that there are different asthma forms or phenotypes, some of which are less responsive to the current mainstays of asthma controller therapy. The current view of asthma treatment may be summarized as follows: that the treatments commonly used at present are indeed effective for the most common form of the disease, as it presents in children and young adults with allergic asthma, but that there are other phenotypes of asthma for which these therapies are less effective, and that represent an unmet medical need. Accordingly, this chapter first reviews the pathophysiology of the most common form of asthma and the basic pharmacology of the agents used in its treatment. It will then turn to discussion of different forms or phenotypes of asthma and the efforts to develop effective therapies for them.
PATHOGENESIS OF ASTHMA
Classic allergic asthma is regarded as mediated by immune globulin (IgE), produced in response to exposure to foreign proteins, like those from house dust mite, cockroach, animal danders, molds, and pollens. These qualify as allergens on the basis of their induction of IgE antibody production in people exposed to them. The tendency to produce IgE is at least in part genetically determined, and asthma clusters with other allergic diseases (allergic rhinitis, eczema) in family groups. Once produced, IgE binds to high-affinity receptors (FcεR-1) on mast cells in the airway mucosa (Figure 20–1), so that re-exposure to the allergen triggers the release of mediators stored in the mast cells’ granules and the synthesis and release of other mediators. The histamine, tryptase, leukotrienes C4 and D4, and prostaglandin D2 released cause the smooth muscle contraction and vascular leakage responsible for the acute bronchoconstriction of the “early asthmatic response.” This response is often followed in 3–6 hours by a second, more sustained phase of bronchoconstriction, the “late asthmatic response,” associated with an influx of inflammatory cells into the bronchial mucosa and with an increase in bronchial reactivity. This late response is thought to be due to cytokines characteristically produced by TH2 lymphocytes, especially interleukins 5, 9, and 13. These cytokines are thought to attract and activate eosinophils, stimulate IgE production by B lymphocytes, and stimulate mucus production by bronchial epithelial cells. It is not clear whether lymphocytes or mast cells in the airway mucosa are the primary source of the mediators responsible for the late inflammatory response, but the benefits of corticosteroid therapy are attributed to their inhibition of the production of pro-inflammatory cytokines in the airways.
FIGURE 20–1 Conceptual model for the immunopathogenesis of asthma. Exposure to allergen causes synthesis of IgE, which binds to mast cells in the airway mucosa. On re-exposure to allergen, antigen-antibody interaction on mast cell surfaces triggers release of mediators of anaphylaxis: histamine, tryptase, prostaglandin D2 (PGD2), leukotriene (LT) C4, and platelet-activating factor (PAF). These agents provoke contraction of airway smooth muscle, causing the immediate fall in forced expiratory volume in 1 sec (FEV1). Re-exposure to allergen also causes the synthesis and release of a variety of cytokines: interleukins (IL) 4 and 5, granulocyte-macrophage colony-stimulating factor (GM-CSF), tumor necrosis factor (TNF), and tissue growth factor (TGF) from T cells and mast cells. These cytokines in turn attract and activate eosinophils and neutrophils, whose products include eosinophil cationic protein (ECP), major basic protein (MBP), proteases, and platelet-activating factor. These mediators cause the edema, mucus hypersecretion, smooth muscle contraction, and increase in bronchial reactivity associated with the late asthmatic response, indicated by a second fall in FEV1 3–6 hours after the exposure.
The allergen challenge model does not reproduce all the features of asthma. Most asthma attacks are not triggered by inhalation of allergens, but instead by viral respiratory infections. Some adults with asthma have no evidence of allergic sensitivity to allergens, and bronchospasm can be provoked by nonallergenic stimuli such as distilled water aerosol, exercise, cold air, cigarette smoke, and sulfur dioxide. This tendency to develop bronchospasm on encountering nonallergenic stimuli—assessed by measuring the fall in maximal expiratory flow provoked by inhaling serially increasing concentrations of the aerosolized cholinergic agonist methacholine—is described as “bronchial hyper-reactivity.” It is considered fundamental to asthma’s pathogenesis because it is nearly ubiquitous in patients with asthma, and its degree roughly correlates with the clinical severity of the disease.
The mechanisms underlying bronchial hyper-reactivity are incompletely understood but appear to be related to inflammation of the airway mucosa. The anti-inflammatory activity of inhaled corticosteroid (ICS) treatment is credited with preventing the increase in reactivity associated with the late asthmatic response (Figure 20–1).
Whatever the mechanisms responsible for bronchial hyper-reactivity, bronchoconstriction itself results not simply from the direct effect of the released mediators but also from their activation of neural pathways. This is suggested by the effectiveness of muscarinic receptor antagonists, which have no direct effect on smooth muscle contractility, in inhibiting the bronchoconstriction caused by inhalation of allergens and airway irritants.
The hypothesis suggested by this conceptual model—that asthmatic bronchospasm results from a combination of release of mediators and an exaggeration of responsiveness to their effects—predicts that drugs with different modes of action may effectively treat asthma. Asthmatic bronchospasm might be reversed or prevented, for example, by drugs that reduce the amount of IgE bound to mast cells (anti-IgE antibody), prevent mast cell degranulation (cromolyn or nedocromil, sympathomimetic agents, calcium channel blockers), block the action of the products released (antihistamines and leukotriene receptor antagonists), inhibit the effect of acetylcholine released from vagal motor nerves (muscarinic antagonists), or directly relax airway smooth muscle (sympathomimetic agents, theophylline).
The second approach to the treatment of asthma is aimed at reducing the level of bronchial responsiveness. Because increased responsiveness appears to be linked to airway inflammation and because airway inflammation is a feature of late asthmatic responses, this strategy is implemented both by reducing exposure to the allergens that provoke inflammation and by prolonged therapy with anti-inflammatory agents, especially inhaled corticosteroids (ICS).
BASIC PHARMACOLOGY OF AGENTS USED IN THE TREATMENT OF ASTHMA
The drugs most used for management of asthma are adrenoceptor agonists, or sympathomimetic agents (used as “relievers” or bronchodilators) and inhaled corticosteroids (used as “controllers” or anti-inflammatory agents). Their basic pharmacology is presented elsewhere (see Chapters 9 and 39). In this chapter, we review their pharmacology relevant to asthma.
SYMPATHOMIMETIC AGENTS
Adrenoceptor agonists are mainstays in the treatment of asthma. Their binding to β receptors—abundant on airway smooth muscle cells—stimulates adenylyl cyclase and increases the formation of intracellular cAMP (Figure 20–2), thereby relaxing airway smooth muscle and inhibiting release of bronchoconstricting mediators from mast cells. They may also inhibit microvascular leakage and increase mucociliary transport. Adverse effects, especially of adrenoceptor agonists that activate β1 as well as β2 receptors, include tachycardia, skeletal muscle tremor, and decreases in serum potassium levels.
FIGURE 20–2 Bronchodilation is promoted by cAMP. Intracellular levels of cAMP can be increased by β-adrenoceptor agonists, which increase the rate of its synthesis by adenylyl cyclase (AC); or by phosphodiesterase (PDE) inhibitors such as theophylline, which slow the rate of its degradation. Bronchoconstriction can be inhibited by muscarinic antagonists and possibly by adenosine antagonists.
Sympathomimetic agents that have been widely used in the treatment of asthma include epinephrine, ephedrine, isoproterenol, and albuterol and other β2-selective agents (Figure 20–3). Because epinephrine and isoproterenol increase the rate and force of cardiac contraction (mediated mainly by β1 receptors), they are reserved for special situations (see below).
FIGURE 20–3 Structures of isoproterenol and several β2-selective analogs.
In general, adrenoceptor agonists are best delivered by inhalation. This results in the greatest local effect on airway smooth muscle with the least systemic toxicity. Aerosol deposition depends on the particle size, the pattern of breathing, and the geometry of the airways. Even with particles in the optimal size range of 2–5 mm, 80–90% of the total dose of aerosol is deposited in the mouth or pharynx. Particles under 1–2 μm remain suspended and may be exhaled. Bronchial deposition of an aerosol is increased by slow inhalation of a nearly full breath and by 5 or more seconds of breath-holding at the end of inspiration.
Epinephrine is an effective, rapidly acting bronchodilator when injected subcutaneously (0.4 mL of 1:1000 solution) or inhaled as a microaerosol from a pressurized canister (320 mcg per puff). Maximal bronchodilation is achieved 15 minutes after inhalation and lasts 60–90 minutes. Because epinephrine stimulates α and β1 as well as β2 receptors, tachycardia, arrhythmias, and worsening of angina pectoris are troublesome adverse effects. The cardiovascular effects of epinephrine are of value for treating the acute vasodilation and shock as well as the bronchospasm of anaphylaxis, but other, more β2-selective agents have displaced its use in asthma.
Ephedrine was used in China for more than 2000 years before its introduction into Western medicine in 1924. Compared with epinephrine, ephedrine has a longer duration, oral activity, more pronounced central effects, and much lower potency. Because of the development of more efficacious and β2-selective agonists, ephedrine is now used infrequently in treating asthma.
Isoproterenol is a potent nonselective β1 and β2 bronchodilator. When inhaled as a microaerosol from a pressurized canister, 80–120 mcg isoproterenol causes maximal bronchodilation within 5 minutes and has a 60- to 90-minute duration of action. An increase in asthma mortality in the United Kingdom in the mid-1960s was attributed to cardiac arrhythmias resulting from the use of high doses of inhaled isoproterenol. It is now rarely used for asthma.
Beta2-Selective Drugs
The β2-selective adrenoceptor agonist drugs, particularly albuterol, are now the most widely used sympathomimetics for the treatment of the bronchoconstriction of asthma (Figure 20–3). These agents differ structurally from epinephrine in having a larger substitution on the amino group and in the position of the hydroxyl groups on the aromatic ring. They are effective after inhaled or oral administration and have a longer duration of action than epinephrine or isoproterenol.
Albuterol, terbutaline, metaproterenol, and pirbuterol are available as metered-dose inhalers. Given by inhalation, these agents cause bronchodilation equivalent to that produced by isoproterenol. Bronchodilation is maximal within 15 minutes and persists for 3–4 hours. All can be diluted in saline for administration from a hand-held nebulizer. Because the particles generated by a nebulizer are much larger than those from a metered-dose inhaler, much higher doses must be given (2.5–5.0 mg vs 100–400 mcg) but are no more effective. Nebulized therapy should thus be reserved for patients unable to coordinate inhalation from a metered-dose inhaler.
Most preparations of β2-selective drugs are a mixture of R and S isomers. Only the R isomer activates the β-agonist receptor. Reasoning that the S isomer may promote inflammation, a purified preparation of the R isomer of albuterol has been developed (levalbuterol). Whether this actually presents significant advantages in clinical use is still unproven.
Albuterol and terbutaline are also available in oral form. One tablet two or three times daily is the usual regimen; the principal adverse effects are skeletal muscle tremor, nervousness, and occasional weakness. This route of administration presents no advantage over inhaled treatment and is rarely prescribed.
Of these agents, only terbutaline is available for subcutaneous injection (0.25 mg). The indications for this route are similar to those for subcutaneous epinephrine—severe asthma requiring emergency treatment when aerosolized therapy is not available or has been ineffective—but it should be remembered that terbutaline’s longer duration of action means that cumulative effects may be seen after repeated injections. Large doses of parenteral terbutaline are sometimes used to inhibit the uterine contractions associated with premature labor.
A newer generation of long-acting β2-selective agonists includes salmeterol (a partial agonist) and formoterol (a full agonist). These long-acting β agonists (LABA) are potent selective β2 agonists that achieve their long duration of action (12 hours or more) as a result of high lipid solubility. This permits them to dissolve in the smooth muscle cell membrane in high concentrations or, possibly, attach to “mooring” molecules in the vicinity of the adrenoceptor. These drugs appear to interact with inhaled corticosteroids to improve asthma control. Because they have no anti-inflammatory action, they should not be used as monotherapy for asthma. Ultra-long-acting β agonists, indacaterol, olodaterol, and vilanterol, need to be taken only once a day but are currently FDA-approved only for the treatment of chronic obstructive pulmonary disease (COPD). Other long-acting β agonists approved in Europe, but not yet in the United States include bambuterol.
Toxicities
Concerns over the potential toxicities of acute treatment of asthma with inhaled sympathomimetic agents—worsened hypoxemia and cardiac arrhythmia—have been largely put to rest. It is true that the vasodilating action of β2-agonist treatment may increase perfusion of poorly ventilated lung units, transiently decreasing arterial oxygen tension (PaO2), but this effect is small, is easily overcome by the routine administration of supplemental oxygen in the treatment of severe attacks of asthma, and is soon made irrelevant by the increase in oxygen tension that follows β-agonist-induced bronchodilation. The other concern, precipitation of cardiac arrhythmias, appears unsubstantiated. In patients presenting for emergency treatment of severe asthma, irregularities in cardiac rhythm improve with the improvements in gas exchange effected by bronchodilator treatment and oxygen administration.
Not all of the concerns over the potential toxicities of chronic treatment with an inhaled β agonist—made easy by the introduction of long-acting β agonists—have been as easily resolved. One that has been resolved is the induction of tachyphylaxis to their bronchodilator action. A reduction in the bronchodilator response to low-dose β-agonist treatment can indeed be shown after several days of regular β-agonist use, but maximal bronchodilation is still achieved well within the range of doses usually given. Tachyphylaxis is more clearly reflected in a loss of the protection afforded by acute treatment with a β agonist against a later challenge by exercise or inhalation of allergen or an airway irritant. It remains to be demonstrated in a clinical trial, however, whether this loss of bronchoprotective efficacy is associated with adverse outcomes.
The demonstration of genetic variations in the β receptor raised the possibility that the risks of adverse effects might not be uniformly distributed among asthmatic patients. Attention has focused on the receptor’s B16 locus. Retrospective analyses of studies of regular β-agonist treatment suggested that asthma control deteriorated among patients homozygous for arginine at this locus, a genotype found in 16% of the Caucasian population but more commonly in African Americans. It was thus tempting to speculate that a genetic variant may underlie the report of an increase in asthma mortality from regular use of a long-acting β agonist in studies involving very large numbers of patients (see below), but several studies of LABA treatment have since shown the differences in multiple measures of asthma control to be nil or very small in asthmatics with different Arg/Gly variations at the B16 locus. One large study of COPD patients has even suggested that regular use of salmeterol reduced the risk of exacerbations in patients homozygous for arginine at the B16 locus. The importance of genetic variants in the gene for the B16 locus in the β receptor is thus uncertain. It is nonetheless certain that pharmacogenetic studies of asthma treatment will continue to be an active focus of research, as an approach to the development of “personalized therapy.”
METHYLXANTHINE DRUGS
The three important methylxanthines are theophylline, theobromine, and caffeine. Their major source is beverages (tea, cocoa, and coffee, respectively). The use of theophylline, once a mainstay of asthma treatment, has waned with demonstration of the greater efficacy of inhaled adrenoceptor agonists for acute asthma and of inhaled anti-inflammatory agents for chronic asthma. Accelerating this decline in theophylline’s use are its toxicities (nausea, vomiting, tremulousness, arrhythmias) and the requirement for monitoring serum levels because of the narrowness of its therapeutic index. This monitoring is made all the more necessary by individual and drug-associated differences in theopylline metabolism.
Chemistry
As shown below (Figure 20–4), theophylline is 1,3-dimethylxanthine; theobromine is 3,7-dimethylxanthine; and caffeine is 1,3,7-trimethylxanthine. A theophylline preparation commonly used for therapeutic purposes is aminophylline, a theophylline-ethylenediamine complex. The pharmacokinetics of theophylline are discussed below (see Clinical Uses of Methylxanthines). Its metabolic products, partially demethylated xanthines (not uric acid), are excreted in the urine.
FIGURE 20–4 Structures of theophylline and other methylxanthines.
Mechanism of Action
Several mechanisms have been proposed for the actions of methylxanthines, but none has been firmly established. At high concentrations, they can be shown in vitro to inhibit several members of the phosphodiesterase (PDE) enzyme family thereby increasing concentrations of intracellular cAMP and, in some tissues, cGMP (Figure 20–2). Cyclic AMP regulates many cellular functions including, but not limited to, stimulation of cardiac function, relaxation of smooth muscle, and reduction in the immune and inflammatory activity of specific cells.
Of the various isoforms of PDE identified, inhibition of PDE3 appears to be the most involved in relaxing airway smooth muscle and inhibition of PDE4 in inhibiting release of cytokines and chemokines, which in turn results in a decrease in immune cell migration and activation. This anti-inflammatory effect is achieved at doses lower than those necessary for bronchodilation.
In an effort to reduce toxicity while maintaining therapeutic efficacy, selective inhibitors of PDE4 have been developed. Many were abandoned after clinical trials showed that their toxicities of nausea, headache, and diarrhea restricted dosing to subtherapeutic levels, but one, roflumilast, has been approved by the FDA as a treatment for COPD, though not for asthma.
Another proposed mechanism is inhibition of cell surface receptors for adenosine. These receptors modulate adenylyl cyclase activity, and adenosine has been shown to provoke contraction of isolated airway smooth muscle and histamine release from airway mast cells. It has been shown, however, that xanthine derivatives devoid of adenosine antagonism (eg, enprofylline) can inhibit bronchoconstriction in asthmatic subjects.
A third mechanism of action may underlie theophylline’s efficacy: enhancement of histone deacetylation. Acetylation of core histones is necessary for activation of inflammatory gene transcription. Corticosteroids act, at least in part, by recruiting histone deacetylases to the site of inflammatory gene transcription, an action enhanced by low-dose theophylline. This interaction should predict that low-dose theophylline treatment would enhance the effectiveness of corticosteroid treatment, and some clinical trials indeed support the idea that theophylline treatment is effective as add-on therapy in patients with asthma or COPD uncontrolled by ICS plus LABA therapy.
Pharmacodynamics
The methylxanthines have effects on the central nervous system, kidney, and cardiac and skeletal muscle as well as smooth muscle. Of the three agents, theophylline is most selective in its smooth muscle effects, whereas caffeine has the most marked central nervous system effects.
A. Central Nervous System Effects
All methylxanthines—but especially caffeine—cause mild cortical arousal with increased alertness and deferral of fatigue. The caffeine contained in beverages—eg, 100 mg in a cup of coffee—is sufficient to cause nervousness and insomnia in sensitive individuals and slight bronchodilation in patients with asthma. The larger doses necessary for more effective bronchodilation cause nervousness and tremor. Very high doses, from accidental or suicidal overdose, cause medullary stimulation, convulsions, and even death.
B. Cardiovascular Effects
Methylxanthines have positive chronotropic and inotropic effects on the heart. At low concentrations, these effects result from inhibition of presynaptic adenosine receptors in sympathetic nerves, increasing catecholamine release at nerve endings. The higher concentrations (> 10 μmol/L, 2 mg/L) associated with inhibition of phosphodiesterase and increases in cAMP may result in increased influx of calcium. At much higher concentrations (> 100 μmol/L), sequestration of calcium by the sarcoplasmic reticulum is impaired.
The clinical expression of these effects on cardiovascular function varies among individuals. Ordinary consumption of methylxanthine-containing beverages usually produces slight tachycardia, an increase in cardiac output, and an increase in peripheral resistance, raising blood pressure slightly. In sensitive individuals, consumption of a few cups of coffee may result in arrhythmias. High doses of these agents relax vascular smooth muscle except in cerebral blood vessels, where they cause contraction.
Methylxanthines decrease blood viscosity and may improve blood flow under certain conditions. The mechanism of this action is not well defined, but the effect is exploited in the treatment of intermittent claudication with pentoxifylline, a dimethylxanthine agent.
C. Effects on Gastrointestinal Tract
The methylxanthines stimulate secretion of both gastric acid and digestive enzymes. However, even decaffeinated coffee has a potent stimulant effect on secretion, which means that the primary secretagogue in coffee is not caffeine.
D. Effects on Kidney
The methylxanthines—especially theophylline—are weak diuretics. This effect may involve both increased glomerular filtration and reduced tubular sodium reabsorption. The diuresis is not of sufficient magnitude to be therapeutically useful.
E. Effects on Smooth Muscle
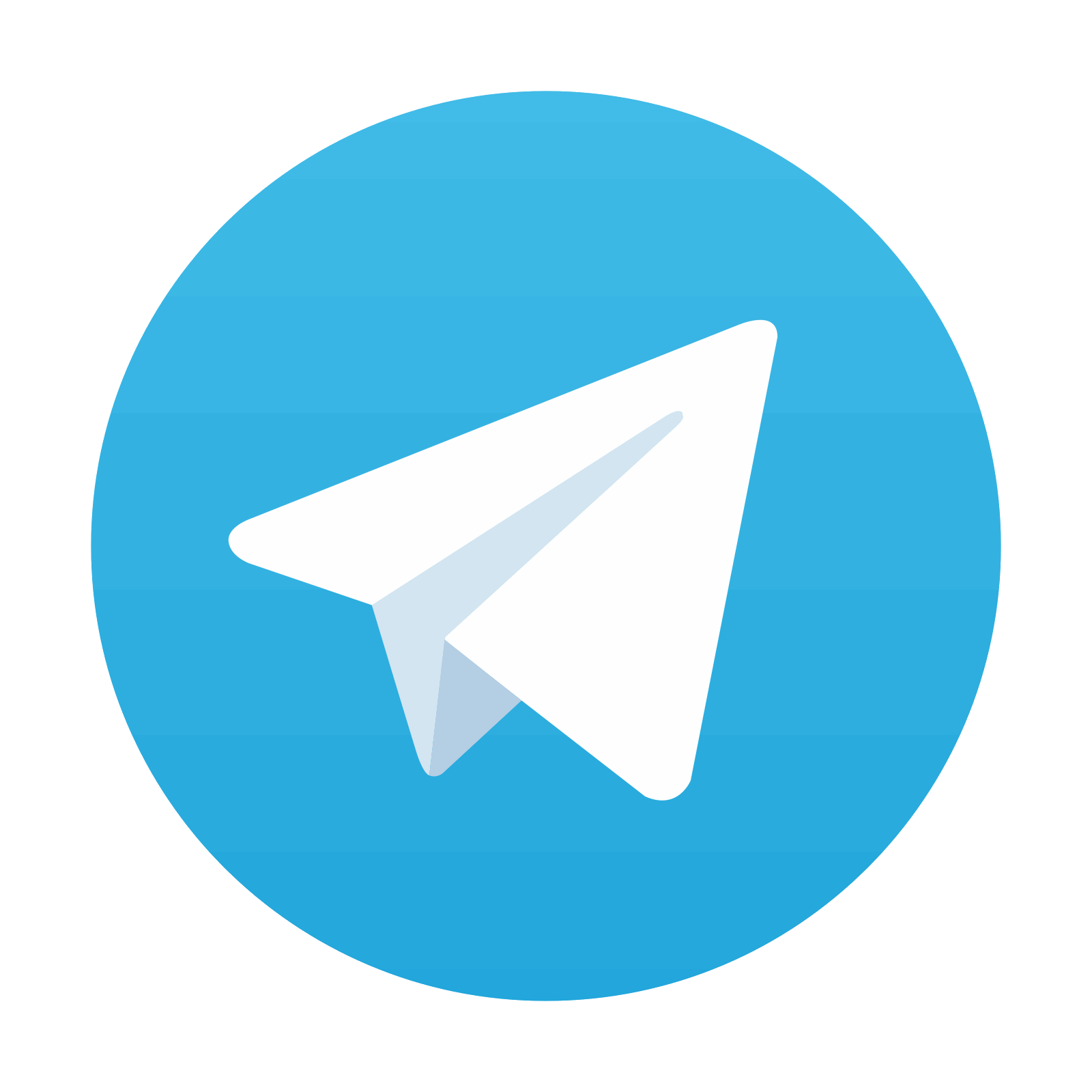
Stay updated, free articles. Join our Telegram channel

Full access? Get Clinical Tree
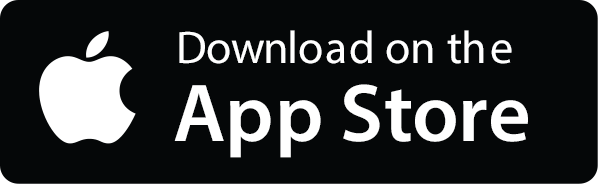
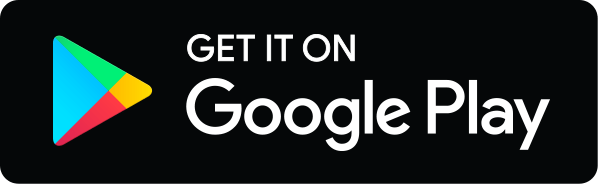