1 Microorganisms and m edicines
The opening paragraph of the previous edition of this book published in 2004 stated that ‘despite continuing poverty in many parts of the world and the devastating effects of HIV and AIDS, the health of the world’s population is progressively improving ’. That trend has been sustained in recent years with the number of AIDS deaths reaching a peak in 2006 and the number of new HIV infections falling 16% between 2000 and 2008. During that same period life expectancy rose in 157 out of the 193 countries reporting data to the World Health Organization and declined in only 9. Much of this improvement is due to better nutrition and sanitation, but improved health care and the greater availability of effective medicines with which to treat common human and animal diseases are also major contributing factors. Substantial inroads have been made in both the prevention and treatment of cancer, cardiovascular disease and other major causes of death in Western society, and of infections and diarrhoeal disease that remain the big killers in developing countries. Several infectious diseases have been eradicated completely, and others from substantial parts of the world. The global eradication of smallpox in 1977 is well documented, and in 2011 rinderpest, the high-mortality cattle disease which, for centuries, has contributed to poverty and famine in Africa and Asia, will also formally be declared extinct; polio and guinea-worm infection are expected to follow in the next few years.
The development of the many vaccines and other medicines that have been so crucial to the improvement in world health has been the result of the large investment in research by the major international pharmaceutical companies. This has led to the manufacture of pharmaceuticals becoming one of the most consistently successful and important industries in many countries, not only in the traditional strongholds of North America, Western Europe and Japan but, increasingly, in Eastern Europe, the Indian subcontinent and the Far East. Worldwide sales of medicines and medical devices are estimated to have exceeded $711 billion in 2007 (the latest year for which statistics are available), and in the UK pharmaceuticals was the industry sector with the largest trade surplus in 2007 having exports of £14.6 billion—a figure that translates into more than £235 000 for each employee in the industry. The growth of the pharmaceutical industry in recent decades has been paralleled by rising standards for product quality and more rigorous regulation of manufacturing procedures. In order to receive a manufacturing licence, a modern medicine must be shown to be effective, safe and of good quality. Most medicines consist of an active ingredient that is formulated with a variety of other materials (excipients) that are necessary to ensure that the medicine is effective and remains stable, palatable and safe during storage and use. While the efficacy and safety aspects of the active ingredient are within the domain of the pharmacologist and toxicolo-gist respectively, many other disciplines contribute to the quality of the manufactured product as a whole. Analytical chemists and pharmacists take lead responsibility for ensuring that the components of the medicine are present in the correct physical form and concentration, but quality is not judged solely on the physicochemical properties of the product: microorganisms also have the potential to influence efficacy and safety.
It is obvious that medicines contaminated with potentially pathogenic (disease-causing) microorganisms are a safety hazard, so medicines administered by vulnerable routes (e.g. injections) or to vulnerable areas of the body (e.g. the eyes) are manufactured as sterile products. What is less predictable is that microorganisms can, in addition to initiating infections, cause product spoilage by chemically decomposing the active ingredient or the excipients. This may lead to the product being under-strength, physically or chemically unstable, or possibly contaminated with toxic materials. Thus, it is clear that pharmaceutical microbiology must encompass the subjects of sterilization and preservation against microbial spoilage, and a pharmacist with responsibility for the safe, hygienic manufacture and use of medicines must know where microorganisms arise in the environment, i.e. the sources of microbial contamination, and the factors that predispose to, or prevent, product spoilage. In these respects, the pharmaceutical microbiologist has a lot in common with food and cosmetics microbiologists, and there is substantial scope for transfer of knowledge between these disciplines.
Disinfection and the properties of chemicals (biocides) used as antiseptics, disinfectants and preservatives are subjects of which pharmacists and other persons responsible for the manufacture of medicines should be familiar, both from the perspective of biocide use in product formulation and manufacture, and because antiseptics and disinfectants are pharmaceutical products in their own right. However, they are not the only antimicrobial substances that are relevant to medicine: antibiotics are of major importance and represent a product category that regularly features among the top five most frequently prescribed. The term ‘antibiotic ’ is used in several different ways: originally an antibiotic was defined as a naturally occurring substance that was produced by one microorganism that inhibited the growth of, or killed, other microorganisms, i.e. an antibiotic was a natural product, a microbial metabolite. More recently the term has come to encompass certain synthetic agents that are normally used systemically (throughout the body) to treat infection. The manufacture, quality control and, in the light of current concerns about resistance of microorganisms, the use of antibiotics, are other areas of knowledge that contribute to the discipline of pharmaceutical microbiology.
Commercial antibiotic production began with the manufacture of penicillin in the 1940s, and for many years antibiotics were the only significant example of a medicinal product that was made using microorganisms. Following the adoption in the 1950s of microorganisms to facilitate the manufacture of steroids and the development of recombinant DNA technology in the last three decades of the 20th century, the use of microorganisms in the manufacture of medicines has gathered great momentum. It led to more than 100 biotechnology-derived products on the market by the year 2000 with another 300 or more in clinical trials. While it is true to say that traditionally the principal pharmaceutical interest in microorganisms is that of controlling them, exploiting microbial metabolism in the manufacture of medicines is a burgeoning area of knowledge that will become increasingly important, not only in the pharmacy curriculum but also in those of other disciplines employed in the pharmaceutical industry. Table 1.1 summarizes these benefits and uses of microorganisms in pharmaceutical manufacturing, together with the more widely recognized hazards and problems that they present.
Looking ahead to the second decade of the 21st century, it is clear that an understanding of the physiology and genetics of microorganisms will also become more important, not just in the production of new therapeutic agents but in the understanding of infections and other diseases. Genetic techniques such as ribotyping are becoming increasingly used to identify cross-infection, reduce transmission and optimize management of hospital-acquired infections, e.g. those due to Clostridium difficile, and, because of the traditional breadth of their science education and their accessibility to the public, pharmacists are not infrequently called upon to explain the terminology and concepts of genetics and other biological sciences to both work colleagues and patients. Several of the traditional diseases that were major causes of death before the antibiotic era, e.g. tuberculosis and diphtheria, are now re-emerging in resistant form—even in developed countries—adding to the problems posed by infections in which antibiotic resistance has long been a problem, and those like Creutzfeldt–Jakob disease, West Nile virus and severe acute respiratory syndrome (SARS) that have only been recognized or have changed in character in recent years.
Not only has the development of resistance to established antibiotics become a challenge, so too has the ability of microorganisms to take advantage of changing practices and procedures in medicine and surgery. Microorganisms are found almost everywhere in our surroundings and they possess the potential to reproduce extremely rapidly; it is quite possible for cell division to occur every 20 minutes under favourable conditions. These characteristics mean that they can adapt readily to a changing environment and colonize new niches. One feature of modern surgery is the ever-increasing use of plastic, ceramic and metal devices that are introduced into the body for a wide variety of purposes, including the commonly encountered urinary or venous catheters and the less common intraocular lenses, heart valves, pacemakers and hip prostheses. Many bacteria have the potential to produce substances or structures that help them to attach to, and grow as biofilms over, the surfaces of these devices, even while combating the immune system of the body. Thus, colonization often necessitates removal and replacement of the device in question—often leading to great discomfort for the patient and substantial monetary cost to the healthcare service. It has been estimated that, on average, a hospital-acquired infection results in an extra 14 days in hospital, a 10% increase in the chance of dying and an additional health-care cost per patient of between £1700 and £4120. The development of strategies for eliminating, or at least restricting, the severity or consequences of these device-related infections is a challenge for pharmacists and microbiologists within the industry, and for many other healthcare professionals.
In addition to an improved understanding of the mechanisms of antibiotic resistance, of the links between antibiotic resistance and misuse, and of the factors influencing the initiation of infections in the body, our insights into the role of microorganisms in other disease states have broadened significantly in recent years. Until about 1980 it was probably true to say that there was little or no recognition of the possibility that microorganisms might have a role to play in human diseases other than clear-cut infections. In recent years, however, our perception of the scope of microorganisms as agents of disease has been changed by the discovery that Helicobacter pylori is intimately involved in the development of gastric or duodenal ulcers and stomach cancer; by the findings that viruses can cause cancers of the liver, blood and cervix; and by the suspected involvement of microorganisms in diverse conditions like chronic fatigue syndrome and Alzheimer ’ s disease. These, and other conditions like Bell ’ s palsy, atherosclerosis and multiple sclerosis, were amongst 16 chronic diseases suspected of having infectious origins that were named in a 2005 report published by the American Academy of Microbiology.
Clearly, a knowledge of the mechanisms whereby microorganisms are able to resist antibiotics, colonize medical devices and cause or predispose humans to other disease states is essential in the development not only of new antibiotics, but of other medicines and healthcare practices that minimize the risks of these adverse situations developing.
2 Scope and content of the book
Criteria and standards for the microbiological quality of medicines depend upon the route of administration of the medicine in question. The vast majority of medicines that are given by mouth or placed on the skin are non-sterile, i.e. they may contain some microorganisms (within limits on type and concentration), whereas all injections and ophthalmic products must be sterile, i.e. containing no living organisms. Products for other anatomical sites (e.g. nose, ear, vagina and bladder) are often sterile but not invariably so (Chapter 22). The microbiological quality of non-sterile medicines is controlled by specifications defining the concentration of organisms that may be present and requiring the absence of specific, potentially hazardous organisms. Thus the ability to identify the organisms present, to detect those that are prohibited from particular product categories, and to enumerate microbial contaminants in the manufacturing environment, raw materials and finished product are clearly skills that a pharmaceutical microbiologist should possess (Chapters 2–6). So, too, is a familiarity with the characteristics of antimicrobial preservatives that may be a component of the medicine required to minimize the risk of microbial growth and spoilage during storage and use by the patient (Chapters 17 and 19).
For a sterile product the criterion of quality is simple: there should be no detectable microorganisms whatsoever. The product should, therefore, be able to pass a test for sterility, and a knowledge of the procedures and interpretation of results of such tests is an important aspect of pharmaceutical microbiology (Chapter 21). Injections are also subject to a test for pyrogens; these are substances that cause a rise in body temperature when introduced into the body. Strictly speaking, any substance which causes fever following injection is a pyrogen, but in reality the vast majority are of bacterial origin, and it is for this reason that the detection, assay and removal of bacterial pyrogens (endotoxins) are considered within the realm of microbiology (Chapter 22).
Sterile medicines may be manufactured by two different strategies. The most straightforward and preferred option is to make the product, pack it in its final container and sterilize it by heat, radiation or other means (terminal sterilization, Chapter 21). The alternative is to manufacture the product from sterile ingredients under conditions that do not permit the entry of contaminating organisms (aseptic manufacture, Chapters 17 and 23); this latter option is usually selected when the ingredients or physical form of the product render it heat-or radiation-sensitive. Those responsible for the manufacture of sterile products must be familiar with the sterilization or aseptic manufacturing procedures available for different product types, and those who have cause to open, use or dispense sterile products (in a hospital pharmacy, for example) should be aware of the aseptic handling procedures to be adopted in order to minimize the risk of product contamination.
The spoilage of medicines as a result of microbial contamination, although obviously undesirable, has as its main consequence financial loss rather than ill health on the part of the patient. The other major problem posed by microbial contamination of medicines, that of the risk of initiating infection, although uncommon, is far more important in terms of risk to the patient and possible loss of life (Chapters 7 and 17). Infections arising by this means also have financial implication of course, not only in additional treatment costs but in terms of product recalls, possible litigation and damage to the reputation of the manufacturer.
The range of antimicrobial drugs used to prevent and treat microbial infections is large; for example, a contemporary textbook of antimicrobial chemotherapy lists no fewer than 43 different cephalosporin antibiotics that were already on the market or the subject of clinical trials at the time of publication. Not only are there many antibiotic products, but increasingly, these products really have properties that make them unique. It is far more difficult now than it was, say, 25 years ago, for a manufacturer to obtain a licence for a ‘copycat ’ product, as licensing authorities now emphasize the need to demonstrate that a new antibiotic (or any new medicine) affords a real advantage over established drugs. Because of this range and diversity of products, pharmacists are now far more commonly called upon to advise on the relative merits of the antibiotics available to treat particular categories of infection than was the case hitherto (Chapters 11, 12, 14 and 15). A prerequisite to providing this information is a knowledge not only of the drug in question, but of the infectious disease it is being used to treat and the factors that might influence the success of antibiotic therapy in that situation, e.g. the potential of the infecting organism to grow as a biofilm in which it is protected both from antibiotics and from the immune system by extracellular polymers or slime layers and, as a consequence, is much more difficult to eradicate (Chapters 7 and 8).
While there was a belief among some commentators a generation ago that infectious disease was a problem that was well on the way to permanent resolution owing to the development of effective vaccines and antibiotics, such complacency has now completely disappeared. Although cardiovascular and malignant diseases are more frequent causes of death in many developed countries, infectious diseases remain of paramount importance in many others, and they account for approximately 23% of the global 58 million deaths per year, with respiratory infection, HIV/AIDS, diarrhoeal disease, tuberculosis and malaria being the prime causes. The confidence that antibiotics would be produced to deal with the vast majority of infections has been replaced by a recognition that the development of resistance to them is likely to substantially restrict their value in the control of certain infections (Chapter 13). Resistance to antibiotics has increased in virtually all categories of pathogenic microorganisms and is now so prevalent that there are some infections, particularly among those acquired in hospitals, for which, it is feared, there will soon be no effective antibiotics. The UK National Audit Office estimated that the cost to the National Health Service of treating hospital-acquired infections exceeded £1 billion in 2007 and the scale of the problem is such that dramatically increasing attention is being paid to infection control procedures that are designed to minimize the risk of infection being transmitted from one patient to another within a hospital (Chapter 16). The properties of disinfectants and antiseptics, the measurement of their antimicrobial activity and the factors influencing their selection for use in hospital infection control strategies or contamination control in the manufacturing setting are topics with which both pharmacists and industrial microbiologists should be familiar (Chapters 18 and 20). Another consequence of increasing antibiotic resistance is that the public are becoming better informed about the need to preserve the antibiotics we presently possess and to avoid their unnecessary use. This has stimulated interest in alternative forms of antimicrobial chemotherapy including the use of materials of plant origin, e.g. tea tree oil, and novel strategies like phage therapy (Chapter 27).
It has long been recognized that microorganisms are valuable, if not essential, in the maintenance of our ecosystems. Their role and benefits in the carbon and nitrogen cycles in terms of recycling dead plant and animal material and in the fixation of atmospheric nitrogen are well understood. The uses of microorganisms in the food, dairy and brewing industries are also well established, but until the late 20th century advances in genetics, immunology and biotechnology, their benefits and uses in the pharmaceutical industry were far more modest. For many years the production of antibiotics and microbial enzyme-mediated production of steroids were the only significant pharmaceutical examples of the exploitation of metabolism of microorganisms. The value of these applications, in both monetary and healthcare terms, has been immense. Antibiotics currently have estimated global sales of $42 billion per annum, and by this criterion they are surpassed as products of biotechnology only by cheese and alcoholic beverages, but the benefits they afford in terms of improved health and life expectancy are incalculable. The discovery of the anti-inflammatory effects of corticosteroids had a profound impact on the treatment of rheumatoid arthritis in the 1950s, but it was the use of enzymes possessed by common fungi that made cortisone widely available to rheumatism sufferers. The synthesis of cortisone by traditional chemical methods involved 31 steps, gave a yield of less than 0.2% of the starting material and resulted in a product costing, even in 1950s terms, $200 per gram. Exploiting microbial enzymes reduced the synthesis to 11 steps and the cost rapidly fell to $6 per gram.
Apart from these major applications, however, the uses of microorganisms in the manufacture of medicines prior to 1980 were very limited. Enzymes were developed for use in cancer chemotherapy (asparaginase) and to digest blood clots (streptokinase), and polysaccharides also found therapeutic applications (e.g. dextran, used as a plasma expander). These were of relatively minor importance, however, compared with the products that followed the advances in recombinant DNA technology in the 1970s. This technology permitted human genes to be inserted into microorganisms, which were thus able to manufacture the gene products far more efficiently than traditional methods of extraction from animal or human tissues. Insulin, in 1982, was the first therapeutic product of DNA technology to be licensed for human use, and it has been followed by human growth hormone, inter-feron, erythropoietin, blood clotting factors and many other products (Chapter 25). DNA technology has also permitted the development of vaccines which, like that for the prevention of hepatitis B, use genetically engineered surface antigens rather than whole natural virus particles, so these vaccines are more effective and safer than those produced by traditional means (Chapters 10 and 24).
All these developments, together with miscellaneous applications in the detection of mutagenic and carcinogenic activity in drugs and chemicals and in the assay of antibiotics, vitamins and amino acids (Chapter 26), have ensured that the role of microorganisms in the manufacture of medicines is now well recognized, and that a basic knowledge of immunology (Chapter 9), gene cloning and other biotechnology disciplines (Chapter 25) is an integral part of pharmaceutical microbiology.
< div class='tao-gold-member'>
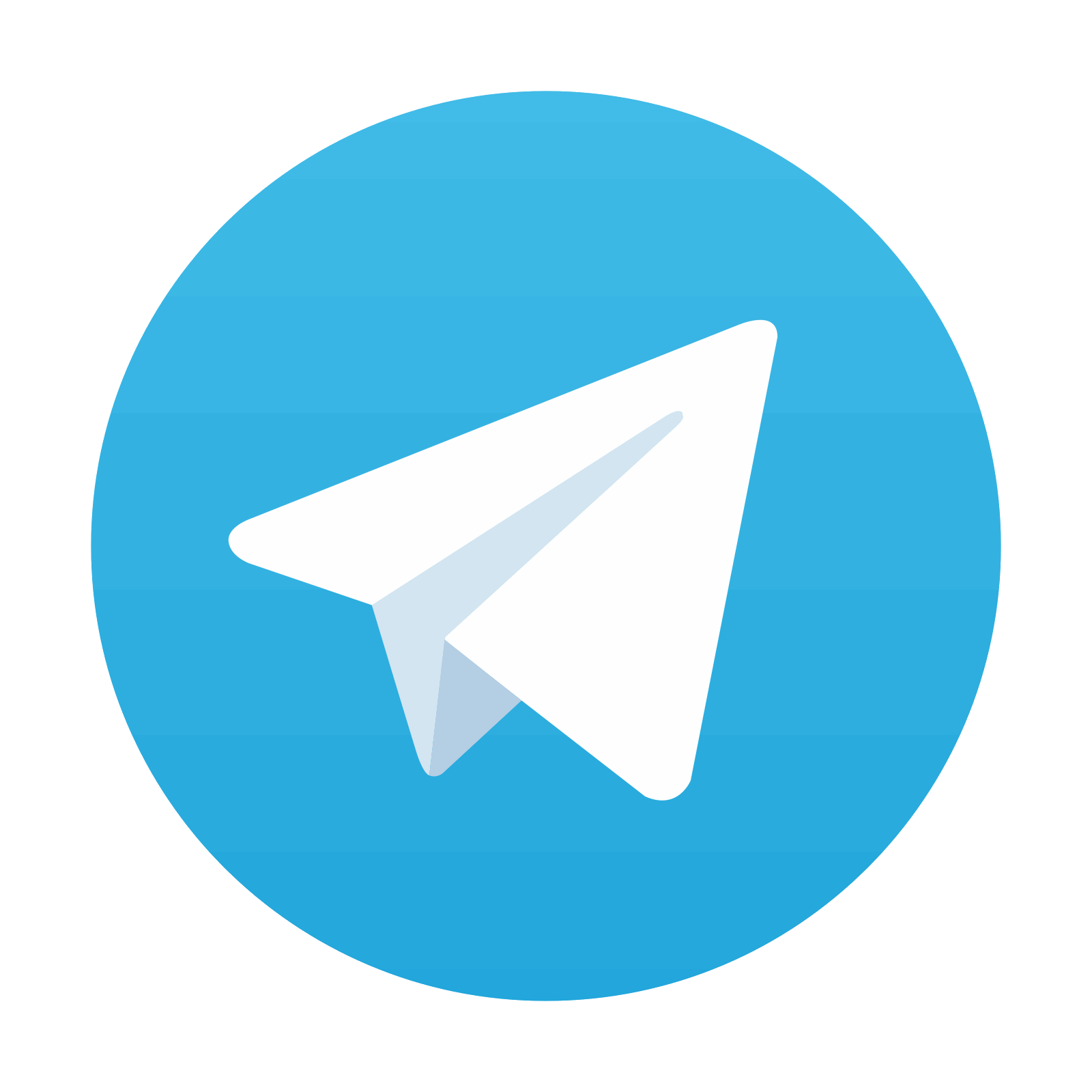