morphologic alterations have no apparent clinical consequence. In other disorders—such as tyrosinemia and glycogen storage disease (GSD) IV—progressive liver disease is common. A variety of histologic alterations—including hepatitis, steatosis, cirrhosis, cholestasis, ductopenia, ductular proliferation, neoplasia, and storage—can be seen in IEM, and many IEM cause similar morphologic alterations (see Chapter 15).
TABLE 5-1 CLINICAL SYMPTOMS IN PATIENTS WITH INBORN ERRORS OF METABOLISM | |
---|---|
|
TABLE 5-2 PLACENTAL FINDINGS IN LYSOSOMAL STORAGE DISEASES | |||||||||||||||||||||||||||||||||||||||||||||||||||||
---|---|---|---|---|---|---|---|---|---|---|---|---|---|---|---|---|---|---|---|---|---|---|---|---|---|---|---|---|---|---|---|---|---|---|---|---|---|---|---|---|---|---|---|---|---|---|---|---|---|---|---|---|---|
|
that every NBS program includes a uniform screening panel (Table 5-4) and advises on the addition of new tests to the screening panel (http://www.hrsa.gov/advisorycommittees/mchbadvisory/heritabledisorders/). The Web site has a list of conditions that have been nominated and are under current review.
TABLE 5-3 ULTRASTRUCTURAL APPEARANCE OF LYSOSOMAL STORAGE DISEASES IN SKIN AND CONJUNCTIVAL BIOPSIES | ||||||||||||||||||||||||||||||||||||||||||||||||||||||||
---|---|---|---|---|---|---|---|---|---|---|---|---|---|---|---|---|---|---|---|---|---|---|---|---|---|---|---|---|---|---|---|---|---|---|---|---|---|---|---|---|---|---|---|---|---|---|---|---|---|---|---|---|---|---|---|---|
|
includes the use of molecular techniques to detect a panel of common mutations. The initial screen is done by measurement of immunoreactive trypsinogen and then followed by molecular genetic testing of the CFTR gene and sweat testing. The genetic testing involves determination of a panel of common mutations, and a diagnosis is made based on a combination of sweat test results and genetic testing (5).
TABLE 5-4 RECOMMENDED UNIFORM SCREENING PANEL FOR ALL STATES | ||||||||||||
---|---|---|---|---|---|---|---|---|---|---|---|---|
|
TABLE 5-5 MASON CATEGORIES OF LYSOSOMAL STORAGE DISORDERS | ||||||||||||||||||||
---|---|---|---|---|---|---|---|---|---|---|---|---|---|---|---|---|---|---|---|---|
|
The central and peripheral nervous systems are particularly vulnerable to lysosomal storage diseases and therefore often affected. Neurologic manifestations include developmental delay, dementia, speech impairment, deafness, visual loss, ophthalmoplegia, leukodystrophy, seizures, ataxia, peripheral neuropathy, and macrocephaly (15).
Hepatosplenomegaly is a feature of many of these diseases including Gaucher disease, Niemann-Pick disease, and mucopolysaccharidosis (MPS). To what extent Kupffer cells or hepatocytes are affected varies depending on the disease process.
Deposition of storage material like glycosaminoglycans in growth plates and cartilage disrupts normal development and maturation of the skeleton. As a result, these patients often have coarse, dysmorphic facial features. “Dysostosis multiplex” is a term that is often used for the skeletal manifestations that include coarse, dysmorphic facial features, short stature, as well as bone and joint abnormalities.
Toxic effect: At least in some cases, cellular and neuronal dysfunction is independent of neuronal storage. In Krabbe disease, secondary increase of the lysosphingolipid psychosine is thought to have toxic effects (12).
Vesicle function and trafficking: Lysosomes are connected to many key vesicle and cell trafficking systems, including autophagy and exocytosis. Lysosomal storage diseases lead to disruption of pH gradients in the endomembrane system that can disrupt function of many proteins (18). Autophagy and exocytosis may be disrupted (18).
Lipid composition: Changes in the composition of lipids in cell membranes can alter membrane fluidity and affect receptor signaling. This is, for example, observed in Niemann-Pick disease type C.
Signaling and activation of apoptosis: Some of the accumulating storage products can act as ligands for cellular receptors. Examples include galactoceramide in Krabbe and glycosaminoglycans in mucopolysaccharidoses. These disruptions in cell signaling can lead to activation of proapoptotic pathways (12). Activation of the unfolded protein response resulting from disrupted function of the endoplasmic reticulum can also be a pathway leading to apoptosis.
Inflammation: Niemann-Pick disease (types A, B, C) and Gaucher disease as diseases associated with prominent involvement of the reticuloendothelial system can lead to release of inflammatory cytokines from cells like microglial cells.
Calcium homeostasis: Storage products can disrupt calcium handling in the endoplasmic reticulum affecting cellular calcium homeostasis.
Enzyme replacement therapies are approved and used for Pompe disease, adult-onset acid maltase deficiency, non-neuropathic type I Gaucher disease, Fabry disease, MPS I S, MPS I S/H, MPS II, and MPS VI. Replacement therapies for alpha-mannosidosis, GM1 gangliosidosis, Wolman disease, and MPS IV A are in clinical trials. Studies with intrathecal administration and with combination therapies are ongoing (12,18,27,28).
Substrate reduction therapies aim to reduce the upstream synthesis of accumulating storage products. Miglustat (N-butyl-deoxygalactonojirimycin) is a competitive inhibitor of ceramide glucosyltransferase and is of potential use in the treatment of Gaucher I and III as well as Niemann-Pick disease type C (27).
Pharmacologic chaperones stabilize a protein during the transport and processing in the endoplasmic reticulum, preventing mutant protein from being sent for early degradation and allowing for the expression of mutant proteins with residual activity. Miglustat has shown beneficial effects in mouse models of Fabry disease (27).
Bone marrow transplantation has been shown to benefit patients with Krabbe disease and Hurler disease and some patients with late-onset metachromatic leukodystrophy if performed before onset of symptoms. Skeletal dysplasia in Hurler disease is, however, not fully corrected, and treated children with Krabbe disease may still develop manifestations such as motor difficulty (15,29). Gene therapy is also being studied as another approach to treatment (30).
cardiac disease, or cerebrovascular disease (38). Late-onset manifestations with cardiac disease with or without renal involvement can be seen even into the 6th decade, and the above cited incidence may underestimate the overall disease burden (37,39). In contrast to other X-linked diseases, the phenotype in female heterozygotes is variable, but can be associated with significant medical problems mimicking those of the classic disease seen in males (40). Hemizygotes and heterozygotes with B or AB blood type are more severely affected due to the additional accumulation of B-specific glycolipid.
and tubules are seen in endothelial, perithelial, and smooth muscle cells (41,42). Glomerular podocytes, endothelial, mesangial, interstitial, and tubular epithelial cells all contain storage material. Podocyte storage causes cellular injury, followed by glomerular capillary wall thickening, progressive mesangial matrix expansion, glomerulosclerosis, and, eventually, end-stage renal disease (Figure 5-2A to C). Some patients develop hypertrophic obstructive cardiomyopathy. Storage material in arteries can lead to luminal obstruction and ischemic lesions that may, for example, be found in the brain. There is typically no clinically significant liver involvement, but morphologic studies may show Kupffer cells that have a tan appearance in H&E sections and storage that is birefringent and crystalline in frozen sections stained with the Schultz method.
endothelial cells, placental syncytiotrophoblasts, marrow histiocytes, lymphocytes, lymph nodes, thymus, lung, intestine, pancreas, pituitary, thyroid, salivary gland, skin (including sweat glands), and conjunctiva (45,46). Visceral storage material is fibrillogranular (45,46). Definitive diagnosis rests on demonstrating beta-galactosidase deficiency in leukocytes, fibroblasts, or amniocytes or on DNA analysis.
aniline dyes that gave rise to the historical naming of this entity. An atypical form is caused by mutations in the cofactor saposin B (69). Different mutations have been described, and patients have a variable course with progressive neurologic disease resulting in several clinical forms with infantile, juvenile, and adult types. The central and peripheral nervous systems show demyelination, and the cerebellum is atrophic with Purkinje and granular cell loss. Spherical aggregates of metachromatic material that are 15 to 20 µm in diameter occur in oligodendrocytes, in macrophages within Virchow-Robin spaces, and in Schwann cells. This material comprises sulfatide, cholesterol, and phosphatides. In frozen sections, it stains positive with PAS, Alcian blue, and colloidal iron, and it is brown metachromatic (with 1% cresyl violet at low pH) and stains purple with toluidine blue. On electron microscopic studies, the storage material in oligodendrocytes, astrocytes, and Schwann cells is composed of closely packed, lamellar, amorphous, or prismatic material with alternating leaflets and tubules giving it a “herringbone” or “tuffstone” pattern (Figure 5-5A,B).
mental retardation, seizures, recurrent infections, coarse facies, dysostosis multiplex and growth retardation, angiokeratoma corporis diffusum, and visceromegaly (73,77). The clinical course is variable. Most patients reach the second decade of life. The CNS, conjunctiva, muscle, skin and sweat gland epithelium, and peripheral blood lymphocytes all show granular lysosomal storage by EM (Figure 5-7). Diagnosis is based on demonstrating deficient alpha-l-fucosidase in leukocytes and fibroblasts. Some clinically normal individuals have low alpha-l-fucosidase levels in plasma.
heparan, chondroitin, and keratan sulfate, with resultant storage of undegraded GAG in lysosomes in a variety of cell types (12). The clinical presentation is variable but may include facial dysmorphism (Figure 5-9A,B), bone/joint dysplasia (Figure 5-9C), hepatosplenomegaly, neurologic impairment, developmental regression, and short life span to mild clinical phenotype with normal life expectancy (Table 5-6) (87,88). All MPS are autosomal recessive except X-linked Hunter syndrome. Hurler (type I) and Hunter (type II) syndromes are the most common types. Scheie and Hurler-Scheie syndromes are subtypes of MPS I with a milder disease. Some infants, particularly with MPS VII, present with hydrops (Table 5-2).
nerve or cord compression can be manifestations of soft tissue involvement (Figure 5-9C, G, and H) (Table 5-6) (89). Vessel walls and heart valves are often affected with storage with resultant sclerosis (Figure 5-9E,F), and endocardial fibroelastosis may occur. Neurons store both GAG and gangliosides leading to formation of membranous cytoplasmic bodies, zebra bodies, and fibrillogranular storage material (Figure 5-9I). Neuronal loss and gliosis are seen in some patients, and meningeal storage may contribute to hydrocephalus.
lysosomal storage. LM of thick sections of tissue prepared for EM is useful for identifying the multiple clear cytoplasmic vacuoles indicative of lysosomal storage. Enzyme assay of serum, leukocytes, or fibroblast culture provides definitive diagnosis, and carrier testing using DNA analysis is practical. Enzyme replacement therapy is now available for some forms (87,88).
TABLE 5-6 MUCOPOLYSACCHARIDOSES | |||||||||||||||||||||||||||||||||||||||||||||||||||||||||||
---|---|---|---|---|---|---|---|---|---|---|---|---|---|---|---|---|---|---|---|---|---|---|---|---|---|---|---|---|---|---|---|---|---|---|---|---|---|---|---|---|---|---|---|---|---|---|---|---|---|---|---|---|---|---|---|---|---|---|---|
|
Cholesterol and triglycerides in these cells can also be highlighted histochemically with the Schultz modification of the Lieberman-Burchard reaction. EM shows lipid droplets and membrane-bound angular cholesterol clefts in hepatocytes, Kupffer cells, fibroblasts, and macrophages (Figure 5-10D). The mucosa of the small intestine, particularly duodenum and ileum, is velvety yellow due to lamina propria storage. Adrenal glands are large, hard, and bright yellow, with dystrophic calcification and necrosis of the inner fasciculata and residual fetal cortex. Oligodendroglia, some neurons of the CNS, and Schwann cells of the peripheral nervous system contain lipid. Placental syncytiotrophoblasts may be affected. Demonstration of acid lipase deficiency in tissue, cultivated fibroblasts, or leukocytes confirms the diagnosis.
synthesized lysosomal hydrolases for their appropriate targeting toward lysosomes by adding mannose-6-phosphate as a signal in the Golgi network (93). Phosphotransferase deficiency results in abnormal lysosomal enzyme transport. As a result, newly synthesized enzymes are secreted out of the cell instead of being transferred to lysosomes. This results in elevated plasma levels of lysosomal enzymes. The GNPTAB gene affected in ML II encodes the alpha- and beta-subunits of the hexameric enzyme complex, and the GNPTG gene affected in ML III encodes the gamma subunit.
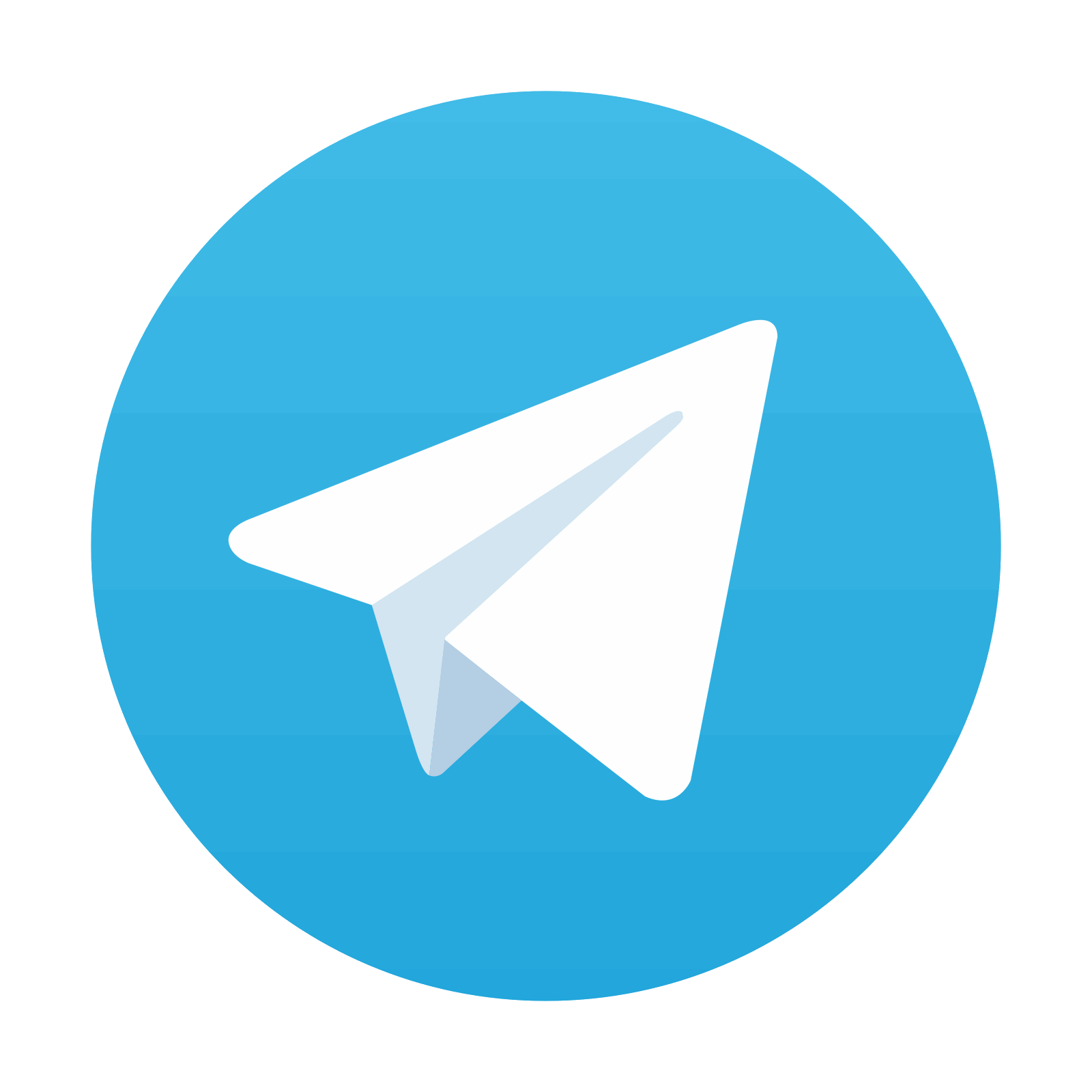
Stay updated, free articles. Join our Telegram channel

Full access? Get Clinical Tree
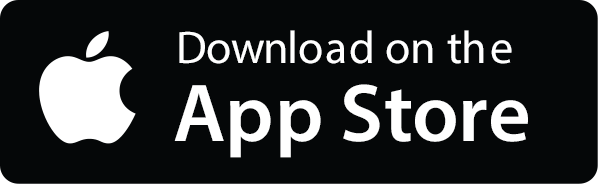
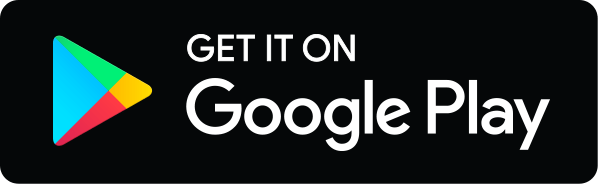
