1 Introduction
1.1 Historical p erspective and s cope of im munology
Progress in immunological science has been driven by the need to understand and exploit the generation of immune states exemplified now by the use of modern vaccines. From almost the first recorded observations, it was recognized that persons who had contracted and recovered from certain infectious diseases were not susceptible (i.e. were immune) to the effects of the same disease when re-exposed to the infection. Thucydides, over 2500 yearsago, described in detail an epidemic in Athens (which could have been typhus or plague) and noted that sufferers were ‘touched by the pitying care of those who had recovered because they were themselves free of apprehension, for no one was ever attacked a second time or with a fatal result’.
Since that time many attempts have been made to induce this immune state. In ancient times the process of variolation (the inoculation of live organisms of smallpox obtained from the diseased pustules of patients who were recovering from the disease) was practised extensively in India and China. The success rate was very variable and often depended on the skill of the variolator. In the late 18th century Edward Jenner, an English country doctor,observed the similarity between the pustules of smallpox and those of cowpox, a disease that affected the udders of cows. He also observed that milkmaids who had contracted cowpox by the handling of diseased udders were immune to smallpox. Jenner deliberately inoculated a young boy with cowpox, and after the boy’s recovery, inoculated him again with the contents of a pustule taken from a patient suffering from smallpox; the boy did not succumb to infection from this first, or any subsequent challenges, with the smallpox virus. Even though the mechanisms by which this protected against smallpox were not understood, Jenner’ s work had shown proof of principle that the harmless stimulation of our adaptive immune system (see section 1.2) was capable of generating an immune state against a specific disease, and thereby provided the basis for the process we now understand as vaccination. The cowpox virus is otherwise known as the vaccinia virus and the term vaccine was introduced by Pasteur to commemorate Jenner’ s work.
In 1801 Jenner prophesied the eradication of small-pox by the practice of vaccination. In 1967 smallpox infected 10 million people worldwide. The World Health Organization (WHO) initiated a programme of confinement and vaccination with the aim of eradicating the disease. In Somalia in 1977 the last case of naturally acquired smallpox occurred, and in 1979 WHO announced the total eradication of smallpox, thus fulfilling Jenner’s prophecy. Many vaccine products are now available designed to provide protection against a range of infectious diseases. Their value has been proven in national vaccination programmes leading to dramatic reductions in morbidity and mortality of such diseases as diphtheria, pertussis, mumps, measles, rubella, and hepatitis A and B.
Further progress in the understanding of the complex nature and functioning of the immune system has been gained through the recognition that many varied forms of pathology, beyond that of infectious disease per se, have an underlying immunological basis, including such diseases as asthma, diabetes, rheumatoid arthritis and many forms of cancer. A basic knowledge of how the immune system functions is essential for health professionals involved in understanding the nature of disease and rationalizing therapeutic strategies. This chapter aims to provide a sound overview of the structure and functioning of the immune system and impart the reader with knowledge which will serve as a platform for the study of more complex specialized texts if and when required.
1.2 Definitions and outline structure of the immune system
The primary function of the immune system is to defend against and eliminate ‘foreign’ material, and to minimize any damage that may be caused as a result of the presence of such material. The term ‘foreign’ includes not only potentially pathogenic microorganisms but also cells recognized as ‘non-self’ and therefore foreign such as the human body’s own virally infected or otherwise transformed (e.g. cancerous) host cells. Foreign material would also include allogeneic (within species) or xenogeneic (between species) transplant tissue and therapeutic proteins administered as medicines if they arose from a different species or were of human origin but had undergone inappropriate post-translational modifications during manufacture or contained impurities. It is also possible that small organic-based drugs may form adducts with endogenous proteins leading to the generation of an immunogen. A good example of such adduct formation is that between the serum protein albumin and the glucuronide metabolite of some non-steroidal antiinflammatory drugs. This adduct is proposed as the basis of some hypersensitivity reactions.
For clarity there are a number of terms that should be defined at this point. An organism which has the ability to cause disease is termed a pathogen. The term virulence is used to indicate the degree of pathogenicity of a given strain of microorganism. Reduction in the virulence of a pathogen is termed attenuation; this can eventually result in an organism losing its virulence completely and it is then said to be avirulent.
An antigen is a component of the ‘foreign ’ material that gives rise to the primary interaction with the body’s immune system. If the antigen elicits an immune response it may then be termed an immunogen. Within a given antigen, e.g. a protein, there will be antigenic determinants or epitopes, which actually represent the antigen recognition sites for our adaptive immune system (see below). For example, within a protein antigen, an epitope for an antibody response will consist of 5–20 amino acids that form either part of a linear chain or a cluster of amino acids brought together conformationally by the folding of the protein. Antibodies (otherwise known as immunoglobulins —Ig) are produced and secreted into biological fluids by our adaptive immune system, are widely used in in vitro diagnostics and have been investigated in therapeutics as a means of targeting drugs to specific sites in the body. A monoclonal antibody is an antibody nominally recognizing only a single antigen (e.g. a single protein) and within which only a single common epitope (e.g. clusters comprising a commonsingle specific amino acid sequence or pattern) is recognized. In contrast, a polyclonal antibody is an antibody nominally recognizing only a single antigen but within which a number of different epitopes (e.g. clusters comprising different amino acid sequences or patterns) are recognized.
The immune system is broadly considered to exhibit two forms of response:
- The innate immune response, which is non-specific, displays no time lag in responsiveness, and is not intrinsically affected by prior contact with infectious agent.
- The adaptive immune response, which displays a time lag in response, involves highly specific recognition of antigen and affords the generation of immunological memory. An example of immunological memory is that provided by the generation of specific lymphocyte memory cell populations following vaccination with an antigen (e.g. diphtheria toxoid). These memory cells reside over a long term in our lymphoid tissue and permit a more rapid and pronounced protective immunological response on future exposure to the same antigen. The adaptive immune system is further subdivided into:
- Humoral immunity, within which the effector cells are B-lymphocytes and where antigen recognition occurs through interactions with antibodies.
- Cell-mediated immunity, within which the effector cells are T-lymphocytes and where antigen recognition occurs through interactions of peptide antigen (presented on the surface of other cell types) with T-cell receptors (TCR) on the plasma membrane of T-lymphocytes. In cell-mediated immunity the peptide antigen must be presented to T-lymphocytes by other cell types in association with a class of plasma membrane molecules termed major histocompatibility complex (MHC) proteins.
- Humoral immunity, within which the effector cells are B-lymphocytes and where antigen recognition occurs through interactions with antibodies.
1.3 Cells of the immune system
A schematic overview of the cells involved in both the innate and adaptive components of the immune response is shown in Figure 9.1. Most of the cells involved in the immune system arise from progenitor cell populations within the bone marrow. The differentiation of these progenitor cells is under the control of a variety of growth factors, e.g. granulocyte-or macrophage-colony stimulating factors (G-CSF and M-CSF, respectively) released by monocyte and macrophage cells as well as by fibroblasts and activated endothelial cells. These growth factors promote the growth and maturation of monocyte and granulocyte populations within the bone marrow before their release into the lymphoid and blood circulations.
Figure 9.1 An overview of the cells involved in the immune response: both innate and adaptive components. The cells arise from a pluripotent progenitor cell within the bone marrow, with their growth and differentiation controlled by numerous growth factors. The T-lymphocytes differentiate in the thymus gland.
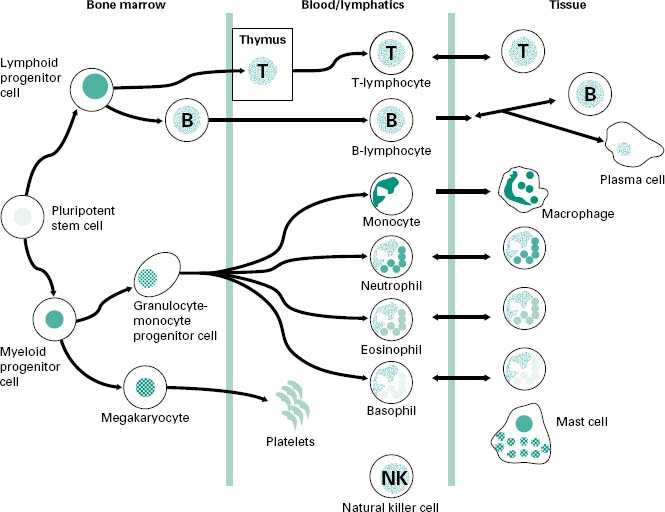
The principal cells of the innate immune system include the following:
• Mononuclear phagocytic cells, which are short-lived (< 8 hours) monocytes in the blood circulation that migrate into tissues and undergo further differentiation to give rise to the long-lived and key effector cell—the macrophage.
• The granulocyte cell populations which include the neutrophil, basophil and eosinophil.
• The mast cell which is a tissue-resident cell that is triggered by tissue damage or infection to release numerous initiating factors leading to an inflammatory response. Such initiating factors include histamine, leukotrienes B4, C4 and D4, proinflammatory cytokines (signal proteins released by leucocytes—white blood cells), such as tumour necrosis factor-α (TNF-α), and chemotactic substances such as interleukin-8 (IL-8). The sudden degranulation of the contents of mast cells is also responsible for the acute anaphylactic reactions to bee stings, penicillins, nuts, etc.
• The natural killer (NK) cell which has a phenotype similar to that of lymphocytes but lacks their specific recognition receptors. The NK cell exploits non-specific recognition to elicit cytotoxic actions against host cells infected with virus and those host cells that have acquired tumour cell characteristics.
The lymphocyte populations also arise from bone marrow progenitor cells. The B-lymphocytes mature or differentiate in the bone marrow before leaving to circulate in the blood and lymph, while T-lymphocytes undergo maturation in the thymus. Antibodies mediating the effector functions of the humoral immune system are produced and secreted from a differentiated B-lymphocyte cell population termed plasma cells.
2.1 Innate barriers at epidermal and mucosal surfaces
Innate defence against the passage of potentially pathogenic microorganisms across epidermal and mucosal barriers involves a range of non-specific mechanisms. Commensal microorganisms living on mucosal surfaces and on membranes such as skin and conjunctiva constitute one such mechanism (see Chapter 8). These commensals are, under normal circumstances, non-pathogenic, and help prevent colonization by pathogenic strains. There are also a number of physical and chemical barriers against microbial entry, including the flow of fluid secretions from tear ducts, the urogenital tract and the skin. Many of these secretions possess bacteristatic or bactericidal activity due to their low pH or the presence of hydrolytic enzymes such as lysozyme (a peptidoglycan hydrolase). Similarly, the mucus barrier covering mucosal surfaces such as the epithelium of the lung serves as a false binding platform for microorganisms, preventing them from interacting with the underlying host cells. In the normal state the hydrated mucus barrier is efficiently cleared under the driving force of beating cilia. The serious lung infections seen in cystic fibrosis arise because patients are unable to clear the bacteria-laden dehydrated mucus effectively.
2.2 Innate defence once epidermal or mucosal barriers have been compromised
The function of the innate defence system against microorganisms that have penetrated into interstitial tissues and the vascular compartment relies largely on the processes of phagocytosis (see section 2.2.3) and of activation of the alternative complement pathway (see section 2.2.4). However, the functions of the innate system when exposed to microbial infection are also critical in the recruitment and activation of cells of the adaptive immune response (see later).
The main cells mediating phagocytosis are the mononuclear phagocytic cells and granulocyte cell populations; of the latter, neutrophils are particularly important. For such cells to function, they must possess receptors to sense signals from their environment. In executing their effector functions they need to secrete a range of molecules that will recruit or activate other immune cells to a site of infection.
Before consideration of the process of phagocytosis, an overview of the mononuclear phagocytic cell and granulocyte cell populations is useful.
2.2.1 Mononuclear phagocytic cells
The mononuclear phagocytic cells include monocytes and macrophages. Monocytes make up approximately 5% of the circulating blood leucocyte population and are short-lived cells (circulating in blood for ≤ 8 hours), but migrate into tissue to give rise to tissue macrophages. The macrophages constitute a long-lived, widely distributed heterogeneous population of cell types which bear different names within different tissues, such as the migrating Kupffer cell within the liver or the fixed mesangial cell within the kidney glomerulus.
The mononuclear phagocytic cells secrete a wide range of molecules too numerous to list in full here. However, these secretions include:
• Molecules which can break down or permeabilize microbial membranes and thereby mediate extracellular killing of microorganisms, e.g. enzymes (lysozyme or cathepsin G), bactericidal reactive oxygen species and cationic proteins.
• Cytokines which can provide innate protective antiviral (e.g. interferon (IFN)-α or-β) and antitumour (e.g. TNF-α) activity against other host cells. A group of cytokines termed chemokines can also serve to chemoattract other leucocytes into an area of ongoing infection or inflammation, for example IL-8 which attracts neutrophils. Yet another group of cytokines has proinflammatory actions (e.g. IL-1 and TNF-α) which, among other outcomes, leads to activation of endothelial and leucocyte cells promoting increased leucocyte extravasation into tissues and, in the case of IL-1, activation of T-lymphocyte populations.
• Bioactive lipids (e.g. thromboxanes, prostaglandins and leukotrienes), which further promote the inflammatory response through actions to increase capillary vasodilation and permeability.
The mononuclear phagocytic cells also possess numerous receptors that interact with their environment. These cells possess, among others:
• Receptors for chemotaxis toward microorganisms, e.g. receptors for secreted bacterial peptides such as formylmethionyl peptide.
• Receptors for complement proteins that serve as leucocyte activators (e.g. C3a and C5a; see section 2.2.4) or complement proteins that serve to coat (opsonize) microorganisms (e.g. C3b). An opsonized microbial surface more readily adheres to a phagocyte membrane, with the opsonin triggering enhanced activity of the phagocyte itself.
• Receptors for promoting adherence, such as lectin receptors interacting with carbohydrate moieties on the surface of the microorganism, or receptors for Fc domains (non-antigen-recognition domains) of antibodies which opsonize microorganisms (e.g. the receptor for the Fc domain of IgG is Fcg), or integrin receptors for cell-cell adhesion (e.g. promoting interaction between a macrophage and T-lymphocyte).
• Receptors for cytokines including those involved in macrophage activation (e.g.IFN.-γ) or limiting macrophage mobility (e.g. macrophage inhibitory factor, MIF) and hence increasing cell retention at a site of infection.
2.2.2 Granulocyte cell populations
The granulocyte cell populations include the neutrophils, basophils and eosinophils. The short-lived (2–3 days) neutrophil is the most abundant granulocyte (comprising > 90% of all circulating blood granulocytes) and is the most important in terms of phagocytosis; indeed, this is the main function of the neutrophil. The receptors and secretions of the neutrophil are similar to those of the macrophage, although notably the neutrophil does not present antigen via MHC class II proteins (see later). The neutrophil is recruited to sites of tissue infection or inflammation by a neutrophil-specific chemotactic factor (IL-8) and is also chemoattracted and activated by some of the same factors described for mononuclear phagocytic cells, including complement protein C3a, bacterial formylmethionyl peptides and leukotrienes. Like macrophages, neutrophils undergo a respiratory burst and are very effective generators of reactive oxygen species.
Eosinophils are poor phagocytic cells and have a specialized role in the extracellular killing of parasites such as helminths, which cannot be physically phagocytosed. Basophils are non-phagocytic cells.
2.2.3 Phagocytosis
Macrophages and neutrophils in particular demonstrate a high capacity for the physical engulfment of particles such as microorganisms or microbial fragments from their immediate extracellular environment. This process (Figure 9.2) is made up of a number of steps:
Figure 9.2 Schematic of phagocytosis showing: (step A) adherence of the microorganism to the surface of the phagocyte; (step B) membrane activation of the phagocyte; (step C) enclosure of phagocytosed material within the phagosome and subsequently the phagolysosome. The mediators of the phagolysosome degradation of the microorganism are shown in the enlarged phagolysosome insert.
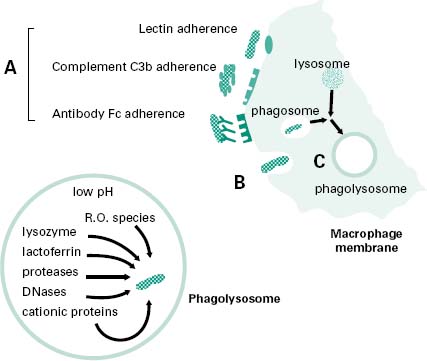
• Chemotaxis of the phagocyte toward the microorganism through signals arising from the microorganism itself (e.g. formylmethionyl peptide), signals arising from complement proteins (e.g. C3a and C5a) generated as part of the activation of the alternative complement pathway (see section 2.2.4), or signals due to release of inflammatory factors (e.g. leukotrienes) secreted by other leucocyte cells situated at the site of an infection.
• Adherence of the microorganism to the surface of the phagocyte (step A in Figure 9.2), involving adhesion through lectin receptors present on the surface of the phagocyte which interact with carbohydrate moieties on the surface of the microorganism; adhesion through complement C3b receptors present on the surface of the phagocyte interacting with C3b molecules that have opsonized the surface of the microorganism; and adhesion through Fc receptors which interact with the Fc domain of antibodies that have opsonized the surface of a microorganism.
• Membrane activation of the phagocyte actin-myosin contractile network to extend pseudopodia around the attached microorganism (step B in Figure 9.2). Membrane activation will also lead to the generation of a ‘respiratory burst’ by the phagocyte which involves an increase in the activity of the phagocyte membrane NADPH oxidase which converts molecular oxygen into bactericidal reactive oxygen species such as superoxide anion (• O−), hydrogen peroxide (H2O2), and in particular hydroxyl radicals (•O H) and halogenated oxygen metabolites (HOCl−).
• The enclosure of phagocytosed material, initially within a membranous vesicle termed a phagosome. Here, cationic proteins such as defensins and reactive oxygen species begin microbial membrane degradation. This is followed within minutes by fusion of the phagosome with a lysosome to form a phagolysosome, whose contents are at an acidic pH of about 5 which is optimal for the continued active breakdown of microbial structural components (step C in Figure 9.2).
2.2.4 Alternative complement pathway
The alternative complement pathway fulfils a critical role in innate immune defence. The complement system comprises at least 20 different serum proteins; many are known by the letter C and a number, e.g. C3. Many of the complement proteins are zymogens, i.e. proenzymes requiring proteolytic cleavage to be enzymically active themselves; some are regulatory in function. The cleavage products of complement proteins are distinguished from their precursor by the suffix ‘a ’ or ‘b ’, e.g. C3a and C3b, with the suffix ‘b’ generally denoting the larger fragment that stays associated with a microbial membrane, and the suffix ‘a’ generally denoting the smaller fragment that diffuses away. The activation of the complement pathway occurs in a cascade sequence, with amplification occurring at each stage, such that each individual enzyme molecule activated at one stage generates multiple activated molecules at the next. In the ‘resting’ state, in the absence of infection, the complement proteins are inactive or have only a low level of spontaneous activation. The cascade is tightly regulated by both soluble and membrane-bound associated proteins. The regulation of the complement pathway prevents inappropriate activation of the cascade (i.e. when no infection is present) and also minimizes damage to host cells during an appropriate complement response to a microbial infection. Complement activation is normally localized to the site(s) of infection.
There are three main biological functions of the alternative complement pathway.
• Opsonization of microbial membranes. This involves the covalent binding of complement proteins to the surface of microbial membranes. This opsonization or coating by complement proteins promotes adherence of the opsonized microbial component(s) to the cell membranes of phagocytic cells. The complement protein C3b is a potent opsonin.
• Activation of leucocytes. This involves complement proteins acting on leucocytes, either at the site of infection or at some distance away, with the result of raising the level of functioning of the leucocytes in immune defence. For example, C3a is a potent leucocyte chemoattractant and also an activator of the respiratory burst.
• Lysis of the target cell membrane. This involves a collection of complement proteins associating on the surface of a microbial membrane to form a membrane attack complex (MAC), which leads to the formation of membrane pores and, ultimately, microbial cell lysis.
Figure 9.3 shows a highly schematized view of the activation cascade for the alternative complement pathway on a microbial membrane surface. The activation steps in the alternative pathway are also shown in Figure 9.7, which contrasts with the activation steps in the classical complement pathway involving antibody.
Figure 9.3 A highly schematized overview of the activation cascade for the alternative complement pathway on a microbial membrane surface. In the presence of a microbial membrane the C3b formed by C3 tickover deposits on the microbial membrane (step A). C3a diffuses away, leading to leucocyte activation. The deposited C3b leads to the generation of a stabilized C3 convertase (step B) which, through a positive feedback loop, leads to the amplified cleavage of more C3. Some C3b associates with the C3 convertase to generate a C5 convertase (step C) which will eventually lead to the generation of an MAC.
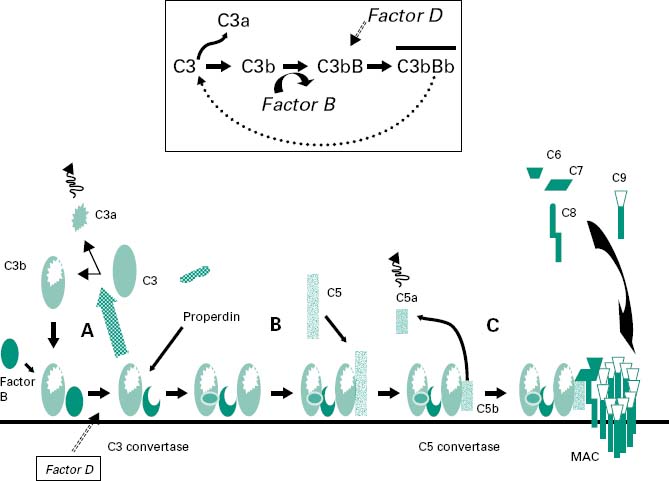
The pivotal protein in the alternative pathway is C3 (195 kDa). Under normal circumstances (in the absence of infection) C3 is cleaved very slowly through reaction with water or trace amounts of proteolytic enzyme to give C3b and C3a. The C3b formed is susceptible to nucleophilic attack by water and is rapidly inactivated to give iC3b. The C3a is not generated in sufficient amounts to lead to leucocyte activation and is rapidly inactivated. This normal low-level cleavage of the C3 molecule is termed ‘C3 tickover’ and it provides low levels of starting material, i.e. C3b, which will be required for full activation of the alternative complement pathway in the case of a microbial infection.
In the presence of a microbial membrane the C3b formed by C3 tickover will be susceptible to nucleophilic attack by hydroxyl or amine groups on the membrane surface, leading to the covalent attachment of C3b to the membrane (step A in Figure 9.3). Once C3b has attached to the membrane, factor B can bind to form a molecule termed C3bB. This complex is stabilized by a soluble protein called properdin. Factor D then enzymically cleaves the bound factor B to generate a molecule termed C3bBb which is the C3 convertase of the alternative pathway (Figure 9.3 inset).
This newly generated stable C3 convertase enzymically cleaves C3 to generate further C3b and C3a molecules, leading to leucocyte activation (by C3a) and greater deposition of C3b on the microbial membrane and hence further generation of C3 convertase molecules. In effect the microbial membrane has activated a positive feedback loop with cleavage of C3 to generate high amounts of C3b and C3a molecules.
The deposited C3b not only leads to the formation of the C3 convertase but also coats the microbial membrane as an opsonin and so promotes binding to phagocyte cell membranes. Some of the deposited C3b associates with the newly formed C3 convertase to generate a complex termed C3bBb3b, which is the C5 convertase of the alternative pathway (step B in Figure 9.3). This C5 convertase binds the complement protein C5 and cleaves it into C5a (a leucocyte activator) and C5b (an opsonin). The C5b remains associated with the membrane and acts as a plat-form for the sequential binding of complement proteins C6, C7, C8 (step C in Figure 9.3). The α-chain of the C8 molecule penetrates into the microbial membrane and mediates conformational changes in the incoming C9 molecules such that C9 becomes amphipathic (simultaneously containing hydrophilic and hydrophobic groups). In this form it is capable of insertion through the microbial membrane where it mediates a polymerization process that gives the MAC. The MAC generates transmembrane channels within the microbial membrane with the osmotic pressure of the cell leading to an influx of water and eventual microbial cell lysis.
Differences between host cell membranes and microbial cell membranes mean that the cascade is only activated in the prresence of microorganisms, so C3 tickover cannot give rise to full activation of the alternative pathway in the absence of microbial membrane. Stable deposition of a functional C3 convertase only occurs on the microbial cell surface. The differences that exist include, for example:
• lipopolysaccharide or peptidoglycan on microbial membranes that promote the binding of C3b
• the high sialic acid content of host cell membranes that promotes the dissociation of any C3 convertase formed on host surfaces
• the presence of specific host cell membrane proteins that also serve a key regulatory function.
Decay activating factor (DAF) or complement receptor type 1 (CR1) are host cell membrane proteins that serve to competitively block the binding of factor B with C3b and hence inhibit formation of a C3 convertase; they also promote disassembly of any C3 convertase formed. Membrane cofactor protein (MCP) and CR1 are further host cell membrane proteins that promote the displacement of factor B from its binding with C3b. Host cell membranes also possess a protein, CD59, which prevents the unfolding of C9—a required step for membrane insertion to form an effective MAC.
3 The humoral adaptive immune system
The humoral immune response is mediated through antibody–antigen interactions. B-lymphocytes in their naive state, unstimulated by antigen, possess antibody molecules on their cell membrane, which serve a surveillance function to recognize any invading antigen. A B-lymphocyte that has bound antigen is capable of differentiating into a plasma cell which, under the influence of signals from helper T-cells, produces a fuller repertoire of antibody molecules (i.e. a fuller range of antibody classes), which are then secreted from the plasma cell into the extracellular environment to bring about a range of humoral effector functions.
3.1 B-lymphocyte antigens
As briefly discussed in section 1.2, a B-cell antigen is a substance or molecule specifically interacting with an antibody, and which may lead to the further production of antibody and an immunological response. Typically B-lymphocyte antigens are proteins within which the epitopes each consist of clusters of 5–20 amino acid residues. B-lymphocyte epitopes arise most commonly from the three-dimensional folding of proteins (i.e. conformational epitopes), although they may also consist of a sequential linear sequence of amino acids within the polypeptide chain (linear epitopes). As a general rule, there is a gradient of increasing immunogenicity with increasing molecular weight of protein. Further, the higher the structural complexity of the protein or polypeptide antigen, the higher the level of immunogenicity it is likely to exhibit. Thus, a polypeptide comprising a single amino acid such as polylysine will be expected to be a weaker immunogen than a protein of equivalent molecular weight made up of a diverse range of amino acids.
Polysaccharides tend not to be good immunogens for B-lymphocytes. When a polysaccharide serves as the sole immunogen, the humoral response obtained is termed ‘T-cell-independent’ because the polysaccharide does not elicit helper T-lymphocyte cooperation (see section 4). The consequences of a T-cell-independent humoral response include the lack of production of memory B-cell populations and the lack of synthesis by the plasma cell of the full range of antibody subclasses, i.e. T-cell-independent humoral responses mainly involve the production of IgM antibody. For improved immunogenicity carbohydrate antigens are conjugated to proteins which allow a more effective ‘T-cell dependent’ humoral response, i.e. one that affords the generation of memory B-cell populations and of the synthesis of the full range of antibody subclasses. This strategy is used in a number of current vaccine products, e.g. meningococcal group C conjugate vaccine contains the capsular polysaccharide antigen of Neisseria meningitidis group C conjugated to Corynebacterium diphtheriae protein. Pure nucleic acid and lipid serve as very poor antigens.
3.2 Basic structure of antibody molecule
Figure 9.4 shows an antibody monomer with a fourpolypeptide subunit structure, where the subunits are linked through disulphide bonding. The basic monomer structure can be considered the same for all the different classes of antibody (see below) even though some may form higherorder structures, e.g. IgM is a pentamer made up of five antibody monomer units.
Figure 9.4 An antibody monomer consisting of a four-polypeptide subunit structure, where the subunits are linked through disulphide bonding. The Fab fragment is concerned with antigen recognition, while the Fc region determines the various effector functions of antibodies. A horizontal line of symmetry can be drawn through the antibody structure bisecting the molecule into a single heavy chain and a single light chain and clearly showing the bivalency in antigen recognition.
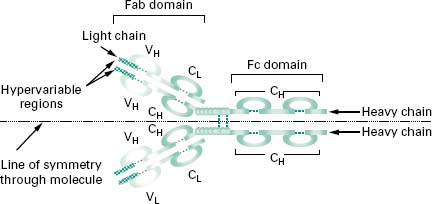
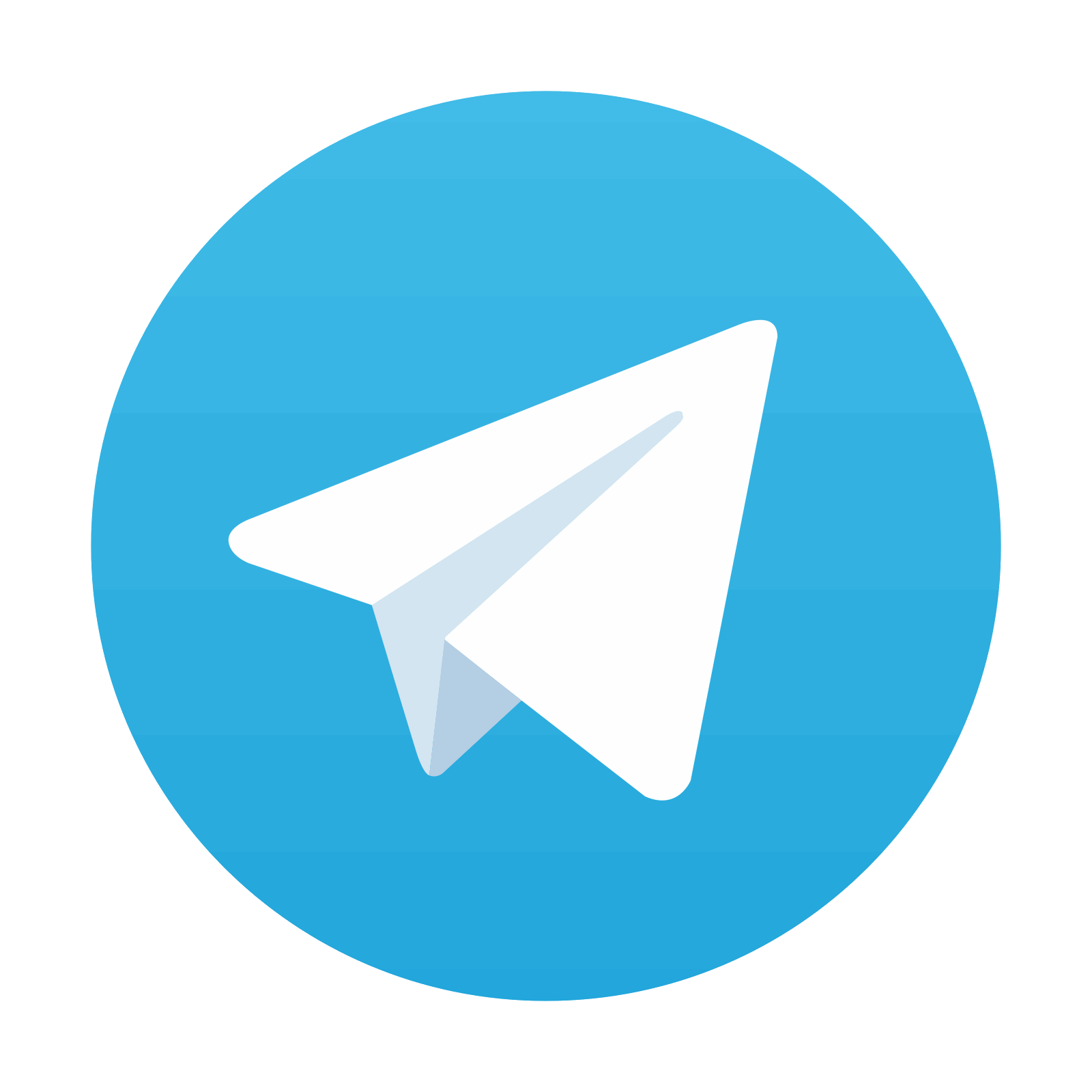