12 High-performance liquid chromatography
Structural factors which govern rate of elution of compounds from HPLC columns
More advanced consideration of solvent selectivity in reverse-phase chromatography
Summary of stationary phases used in HPLC
A more advanced consideration of reverse-phase stationary phases
Summary of detectors used in HPLC
Performance of a diode array detector
Applications of HPLC to the quantitative analysis of drugs in formulations
Assays involving more specialised HPLC techniques
Applications
• The combination of high-performance liquid chromatography (HPLC) with monitoring by UV/visible detection provides an accurate, precise and robust method for quantitative analysis of pharmaceutical products and is the industry standard method for this purpose.
• Monitoring of the stability of pure drug substances and of drugs in formulations, with quantitation of any degradation products.
• Measurement of drugs and their metabolites in biological fluids.
• Determination of partition coefficients and pKa values of drugs and of drug protein binding.
Strengths
• Easily controlled and precise sample introduction ensures quantitative precision.
• HPLC is the chromatographic technique which has seen the most intensive development in recent years, leading to improved columns, detectors and software control.
• The variety of columns and detectors means that the selectivity of the method can be readily adjusted.
• Compared to gas chromatography (GC) there is less risk of sample degradation because heating is not required in the chromatographic process.
Introduction
High-performance liquid chromatography (HPLC) is the technique most commonly used for the quantitation of drugs in formulations (Fig. 12.1). Pharmacopoeial assays still rely quite heavily on direct UV spectroscopy but, in industry, detection by UV spectrophotometry is usually combined with a preliminary separation by HPLC. The theoretical background of HPLC has been dealt with in Chapter 10. There are many comprehensive books on this technique.1–5
Instrumentation
A standard instrumental system for isocratic elution consists of:
(ii) a pump capable of pumping solvent up to a pressure of 4000 psi and at flows of up to 10 ml/min
(iii) a loop injector, which may be fitted with a fixed-volume loop of between 1 and 200 μl (20 μl is often used as standard)
(iv) a column, which is usually a stainless steel tube packed, usually, with octadecylsilane-coated (ODS-coated) silica gel with an average particle diameter (3, 5 or 10 μm)
(v) a detector, which is usually a UV/visible detector, although for specialist applications a wide range of detectors is available
(vi) a data capture system, which may be a computing integrator or a PC with software suitable for processing chromatographic data
(vii) the column is connected to the injector and detector with tubing of narrow internal diameter, ca 0.2 mm, in order to minimise ‘dead volume’, i.e. empty space in the system where chromatography is not occurring and band broadening can occur by longitudinal diffusion
(viii) more advanced instruments may have automatic sample injection and a column oven and are capable of mixing two or more solvents in varying proportions with time to produce a mobile-phase gradient.
Stationary and mobile phases
There are two principal mechanisms which produce retardation of a compound passing through a column. These are illustrated in Figure 12.2 for silica gel, which is a straight-phase packing, where the mechanism of retardation is by adsorption of the polar groups of a molecule onto the polar groups of the stationary phase and for an ODS-coated silica gel, which is a reversed-phase packing, where the mechanism of retardation is due to partitioning of the molecule into the stationary phase according to its lipophilicity.
Silica gel and ODS silica gel are two of the most commonly used packings for straight- and reversed-phase chromatography applications, respectively, but there is a variety of straight- and reversed-phase packings available, most of which are based on chemical modification of the silica gel surface, although in recent years stationary phases which are based on organic polymers have become available. The extent to which a compound is retained will depend primarily upon its polarity, in the case of silica gel, and primarily upon its lipophilicity in the case of a reversed-phase packing such as ODS silica gel. Most drug molecules have both lipophilic and polar groups. The other factor to consider with regard to the degree of retention of a particular compound, apart from the stationary phase, is the nature of the mobile phase. The more polar a mobile phase, the more quickly it will elute a compound from a silica gel column, and the more lipophilic a mobile phase, the more quickly it will elute a compound from a reversed-phase column. Figure 12.3 shows the effect of increasing the % of organic solvent on the elution of a series of alkyl benzenes. In methanol/water mixture Dolan’s rule of 3 applies where, in reversed phase chromatography, a 10% decrease in methanol content produces a 3 times increase in capacity factor for an analyte. Taking the to value for both columns as 1.1 min then the capacity factors for propylbenzene can be calculated as follows:
Thus supporting the rule of 3.
Prednisolone (see Fig. 12.4 for the structure) is to be eluted from an ODS column. List the following solvent systems in order of decreasing rate at which they will elute prednisolone (i.e. in order of decreasing strength):
Prednisolone is to be eluted from a silica gel column.
List the following systems in order of decreasing rate at which they will elute prednisolone:
2. (i) hexane/isopropanol (90:10); (ii) hexane/dichloromethane (90:10); (iii) dichloromethane/methanol (90:10); (iv) dichloromethane/isopropanol (90:10); (v) dichloromethane/methanol (80:20).
Answers: 1. a. (ii), (iii), (i); b. (iii), (i), (ii); 2. (v), (iii), (iv), (i), (ii).
Structural factors which govern rate of elution of compounds from HPLC columns
Elution of neutral compounds
For a neutral compound it is the balance between its polarity and lipophilicity which will determine the time it takes for it to elute from an HPLC column; the pH of the mobile phase does not play a part. In the case of a reverse-phase column, the more lipophilic a compound is the more it will be retained. For a polar column such as a silica gel column, the more polar a compound is the more it will be retained. Polarity can often be related to the number and hydrogen-bonding strength of the hydroxyl groups present in the molecule; this is illustrated as follows for a series of corticosteroids shown in Figure 12.4. When these compounds are eluted from a reverse-phase column using a mobile phase containing methanol/water (75:25), the expected order of elution would be: prednisolone, betamethasone, betamethasone 17-valerate, betamethasone 21-valerate and betamethasone dipropionate. Prednisolone should elute shortly before betamethasone since it lacks a lipophilic methyl group at position 16 (the fluorine group in betamethasone also contributes to its lipophilicity); the valerates both have large lipophilic ester groups masking one of their hydroxyl groups. The 21-hydroxyl group hydrogen bonds more strongly to the mobile phase, since it is an unhindered primary alcohol. Thus its conversion to an ester has a greater effect on the retention time of the molecule than esterification of the 17-hydroxyl group, which is a tertiary alcohol and is hindered with respect to hydrogen bonding to the mobile phase. Finally, the dipropionate of betamethasone has two lipophilic ester groups masking two hydroxyl groups and this would mean that it would be most strongly retained by a lipophilic stationary phase. Figure 12.5 shows the chromatogram obtained from the mixture of corticosteroids using an ODS column with methanol/water (75:25) as the mobile phase, indicating that the order of elution fits prediction. The lipophilicity of the steroids reflects their pharmaceutical uses since the more lipophilic esters are used in creams and ointments for enhanced penetration through the lipophilic layers of the skin. The order of elution of these steroids would be more or less reversed on a polar silica gel column, although chromatographic behaviour is usually more predictable on reverse-phase columns. Considering the chromatogram shown in Figure 12.5 in more detail, the resolution between the betamethasone 21-valerate and the betamethasone dipropionate is incomplete. Increasing the water content of the mobile phase would result in longer retention times for these two components and better separation; however, increasing the water content would also give very long retention times. In the case of a formulation containing both the 21-valerate and 17,21-dipropionate, another type of column might be chosen to effect separation of these two components within a reasonable length of time, e.g. a silica gel column. If the betamethasone dipropionate were absent from this mixture, a different separation strategy could be adopted to bring the valerate esters closer to betamethasone and prednisolone. It would not be possible to add more methanol to the mobile phase without losing resolution between betamethasone and prednisolone, but, after these two compounds had eluted, if an HPLC system with a binary or ternary gradient system were used, the instrument could be programmed to gradually increase the methanol content in the mobile phase to expedite the elution of the later-running valerates. For example, a suitable solvent programme might be as follows: methanol/water (75:25) for 7 min, then ramping the solvent composition to methanol/water (85:15) up to 17 min. This type of programme would greatly reduce the retention times of the valerates.
Control of elution rate of ionisable compounds by adjustment of pH of mobile phase
This area is not often considered in any detail in books on HPLC; however, pharmacists generally have a good grasp of the concept of pKa and it is worth devoting some space to its effects in relation to HPLC. An additional factor which can be used to control the solvent strength of the mobile phase is pH; pH control is employed mainly in reverse-phase chromatography. However, mobile-phase conditions may be selected in straight-phase chromatography where the ionisation of the analytes is suppressed, and basic compounds are run in a basic mobile phase and acidic compounds are run with an acidic mobile phase. Control of the rate of elution via the pH of the mobile phase is, of course, only applicable to compounds in which the degree of ionisation is dependent on pH, but this covers a majority of commonly used drugs. The pH of the mobile phase can only be set within the range of ca 2–8.5 pH units because of the tendency for extremes of pH to dissolve silica gel and break the bonds between silane-coating agents and the silica gel support. This pH range is gradually being extended with the advent of more stable coatings. The effects of pH on retention time, suprisingly, are as yet not fully understood. The following examples give an approximation of the effect of the pH of the mobile phase on the retention time of drugs on a reverse-phase HPLC column, which provides a starting point for considering the effect of pH on retention time. In fact many drugs are still retained by lipophilic stationary phases to some degree even when they are fully ionised; in this case the drug is probably partitioning into the reverse phase as a lipophilic ion pair. The greatest effects of alteration of pH in the mobile phase are observed within 1 pH unit either side of the pKa value of the drug, i.e. where the partition coefficient of the partially ionised drug varies between 90% and 10% of the partition coefficient of the un-ionised drug (see Ch. 2, p. 31).
The same type of calculation as shown in Calculation example 12.1 can be carried out for basic drugs. Figure 12.6 shows the structures of some local anaesthetic drugs with their pKa values.
The effect of pH on the HPLC retention time of an ionisable acidic drug.
Using the expression introduced in Chapter 2 for the effect of pH on partition coefficient of an acid, it is possible to predict approximately the effect of pH on retention time, since the effect of pH on partition coefficient will reflect its effects on capacity factor and in theory:
Using the observed K′ app at pH 4.2: 9.14 =
Figure 12.7 shows the effect of the pH of the mobile phase on the four local anaesthetics shown in Figure 12.6. The largest effects of pH are on bupivacaine and pentycaine, which are very close in structure; the pH adjustment made in the example is within ± 1 pH unit of their pKa values. The least effect is on procaine, which has a higher pKa (9.0) than the other drugs and is thus already 80% ionised at pH 8.4; for this reason, the lowering of the pH has a less marked effect on its retention time. The effect of pH on prilocaine might initially appear somewhat less than expected but this is because it is closer to to than the other drugs; the decrease in its retention time observed
at the lower pH is, in fact, in line with the decreases observed for bupivacaine and pentycaine. In chromatogram B, the procaine peak has lost some of its integrity due to its proximity to the solvent front; this results in poor trapping of the analyte at the head of the column. The effect of the organic content of the mobile phase on the pKa of analytes is given some additional consideration in Box 12.1.
Box 12.1 Additional consideration of mobile-phase pH
A major factor that is often ignored in preparing mobile phases is the effect of the addition of organic solvent to the buffer. The effect of addition of acetonitrile on the pifa value of acetic acid has been calculated to be as follows:6
Figure 12.8 shows the effect of pH on the retention of a series of acidic non-steroidal anti-inflammatory drugs shown in Figure 12.9.
More advanced consideration of solvent selectivity in reverse-phase chromatography
The simplest selectivity factor that can be varied in reverse-phase chromatography is solvent composition. Lowering the amount of organic solvent in the mobile phase during reverse-phase chromatography increases the retention time. Dolan’s rule of 3 states that a 10% decrease in the organic phase produces a threefold increase in capacity factor, this only really applies to methanol in the case of acetonitrile the increase in capacity factor is approximately 2 for a 10% reduction in organic component. The three common solvents used in reverse-phase chromatography are methanol, acetonitrile and tetrahydrofuran. Another approximate rule is that 40% methanol = 33% acetonitrile = 23% tetrahydrofuran. Apart from eluting power these three solvents also differ in the way that they interact with analytes. Selectivity is important in the impurity profiling of drug substances. Impurities closely related to the drug may elute with very similar retention times. The selection of an optimal solvent system is thus important and in such cases mixtures of three or four solvents may be used. Figure 12.10 shows the effect of using mixtures of three solvents on the separation of hydrocortisone and the closely related steroid cortisone (Fig. 12.11). The cortisone is present in the mixture at 5% of the concentration of hydrocortisone. In chromatogram A, separation was obtained between the two peaks with acetonitrile/water (30:70). In chromatogram B, a mixture of acetonitrile/methanol/water (15:15:70) does not produce a separation, even though the introduction of methanol produces a longer retention time. In chromatogram C, a mixture of acetonitrile/THF/water (15:15:70) causes a reversal in the elution order of hydrocortisone and cortisone. The elution of the smaller, potential impurity, peak for cortisone earlier than the hydrocortisone is more desirable, since even slight tailing of the large peak for the major component can cause closely eluting later-running peaks to be obscured. Such a hit and miss approach for achieving an optimal solvent system for separating out minor impurities could be time consuming. A less hit and miss approach uses log K′ plots to predict retention times. Table 12.1 shows the data obtained for the analysis of two steroidal and two non-steroidal anti-inflammatory (Fig. 12.12) drugs on a reversed phase column in mobile phase consisting of methanol and 0.1% v/v aqueous formic acid. The capacity factors for the four drugs can be calculated and from just two runs log K′ plots can be constructed (Fig. 12.13) based on the assumption that plots of log K′ against percentage of organic solvent is linear where simple reversed phase partitioning is occurring.
As can seen in Figure 12.13, according to the log K′ plots there is an opportunity to produce a method with a short run time since the plots for sulindac and ketoprofen crossover when the percentage of methanol is high. Practical application of this information produced the chromatogram shown in Figure 12.14 where fast separation has been produced with sulindac eluting earlier than ketoprofen.
Software packages such as Drylab® can provide an automated approach to prediction of retention times based on log K′ plots and also model the effects stationary phase particle size, column length and column diameter changes. A popular method for modelling retention times is based on a 2 gradient run. Figure 12.15 shows acebutolol and its impurities which are listed in the EP. Figure 12.16 shows the separation of acebutolol and its impurities using two different solvent gradient methods.
From the retention times obtained in the runs shown in Figure 12.16, Drylab® was asked to predict the mobile phase conditions required for a rapid separation based on a 30 x 2.1 mm column. Figure 12.17 shows the rapid separation of acebutolol from its impurities spiked at the 0.1% w/w reporting level.
Effect of temperature on HPLC
Temperature can also be used to change retention behaviour since capacity factor decreases with an increase in temperature according to the van’t Hoff equation. In addition mass transfer effects are reduced at higher temperatures and thus peak efficiency is better. Returning to the model mixture of cortisone, hydrocortisone, sulindac and ketoprofen, Figure 12.18 shows that increasing temperature both reduces retention times and changes selectivity. Drylab® can be used to model the effects of temperature as well.
Summary of stationary phases used in HPLC
The intention of this book is to focus mainly on applications of techniques to pharmaceutical analysis. Detailed discussions of stationary phases and detectors can be found elsewhere.1, 2, 4, 5 Table 12.2 summarises some of the stationary phases which are used in HPLC. Currently, ODS silica gel or related phases such as octyl silica gel are used for > 80% of all pharmaceutical analyses, as judged from a comprehensive survey of the literature;3 other phases are only used where special selectivity is required, such as for very water-soluble compounds or for bioanalytical separations which may be difficult because the sample matrix produces many interfering peaks. In recent years polymeric phases have become available for certain specialist applications; the surface chemistries of these phases are similar to those of the silica-gel-based phases. Advantages of the polymeric phases are stability to extremes of pH and the lack of secondary interactions of analytes with uncapped silanol groups. Disadvantages include expense and a tendency to swell when in contact with highly lipophilic mobile phases, which can destroy them. Such phases are best used with predominantly aqueous-based mobile phases. In the past 10 years the use of hydrophilic interaction chromatography (HILIC) has increased and in the case of very polar molecules it provides an alternative to ion pair chromatography. However, there a still some doubts whether or not it is sufficiently robust for routine chromatographic methods.
Table 12.2 Some commonly used high-performance liquid chromatography (HPLC) stationary phases
Stationary phase | Applications/comments |
Octadecylsilyl (ODS) silica gel | The most commonly used phase, applicable to most problems in analysis of pharmaceutical formulations. Early phases gave problems with strongly basic compounds because of low purity silica gels and incomplete endcapping of silanol (Si-OH) groups. Amines adsorb strongly onto free silanol groups not covered by the stationary phase. Fully endcapped phases and phases with low metal content are now available, which enable the analysis of strongly basic compounds that formerly tended to produce tailing peaks. ODS silica gel can even be applied to the analysis of peptides, where wide-pore packings are used to improve access of these bulky molecules to the internal surface of the packings |
Octyl silane and butyl silane silica gels | Useful alternatives to ODS phases. The shorter hydrocarbon chains do not tend to lead to shorter retention times of analytes since the carbon loading on the surface of the silica gel may be higher for these phases and retention time is also dependent on how much of the stationary phase is accessible to partitioning by the analyte7 |
Phenyl silane silica gel | Useful for slightly more selective analyses of compounds containing large numbers of aromatic rings, e.g. propranolol and naproxen, where π-π interactions can occur with the phenyl groups on the stationary phase if the analyte is deficient π electrons, e.g. nitro compounds. These interactions are, however, very subtle |
Silica gel | Often used in the past for problematical compounds but, with gradual improvement of reverse phases, increasingly less used. Useful for chromatography of very lipophilic compounds such as in the separation of different classes of lipids and in the analysis of surfactants, which tend to form micelles under the conditions used for reverse-phase chromatography |
Aminopropyl silica gel | A moderately polar phase often used for the analysis of sugars and surfactants. In the case of sugars the chromatographic interactions are due to hydrophilic interactions (see below) |
Cyanopropyl silica gel | A moderately polar phase applicable to the analysis of surfactants. Some of its selectivity is due to dipole–dipole interactions |
Strong cation exchanger (SCX) | Usually based on ion pairing of the analyte with sulfonic acid groups on the surface of the stationary phase. Useful for analysis of very polar compounds such as aminoglycosides and other charged sugar molecules and polar bases such as catecholamines |
Strong anion exchanger (SAX) | Usually based on ion pairing of the analyte with quaternary ammonium groups on the surface of the stationary phase. Useful for the separation of polar compounds with anionic groups such as nucleotides and anionic drug metabolites such as sulphates or glucuronides |
A more advanced consideration of reverse-phase stationary phases
All reverse-phase chromatography columns are not equivalent. There are big differences between stationary phases obtained from different manufacturers. Recently, definitive work has been carried out on the classification of reverse-phase stationary phases.8 Six variables that affected the performance of reverse-phase stationary phases were assessed:
(iii) Shape selectivity αT/O = kT/kO
This is based on the ratio of the capacity factors for triphenylene and o-terphenyl, which have very similar structures but o-terphenyl is less rigid than triphenylene and thus has a more three-dimensional structure (Fig. 12.19). Retention of a molecule via lipophilic interactions depends on the size of molecular surface that interacts with the stationary phase. This means for a surface with large lipophilic groups less of the o-terphenyl molecule will be in contact with the surface, whereas the flat triphenylene molecule can contact a uniform surface more fully. Triphenylene, because of its flatness, is less sensitive to the surface of the stationary phase. Stationary phases with smaller alkyl chains attached to the surface, such as octyl, phenyl or butyl, often do not discriminate between o-terphenyl and triphenylene as strongly as octadecyl phases (mobile phase methanol/water (80:20)).
(iv) Hydrogen bonding capacity αC/P = kC/kP
Hydrogen bonding capacity may be measured as the ratio of the capacity factors for phenol (kP) and caffeine (kC). The smaller the value for αC/P the higher the hydrogen bonding capacity, since phenol can hydrogen bond whereas caffeine cannot. The term measures the level of free silanol (Fig. 12.20), or other groups with hydrogen-bonding capacity, in the phase (mobile phase methanol/water (30:70)).
(v) Total ion exchange capacity aB/P = kB/kP (pH 7.6)
This term gives a measure of the total silanol group activity and is based on the ratio of the capacity factors for benzylamine (kB) and phenol (kP) when analysed using a mobile phase with a pH of 7.6, where most of the uncapped silanol groups (Fig. 12.17) in a silica-gel-based chromatographic phase will be ionised. The benzylamine interacts strongly with negatively charged silanol groups and is thus more strongly retained on phases where there are a high number of silanol groups. The higher the value of αB/P, the greater the ion exchange capacity of the phase.
(vi) The acidic ion exchange capacity aB/P = kB/kP (pH 2.7)
Table 12.3 shows the specification details of some Hypersil columns taken from the manufacturer’s literature along with some Tanaka test measurements.8
< div class='tao-gold-member'>
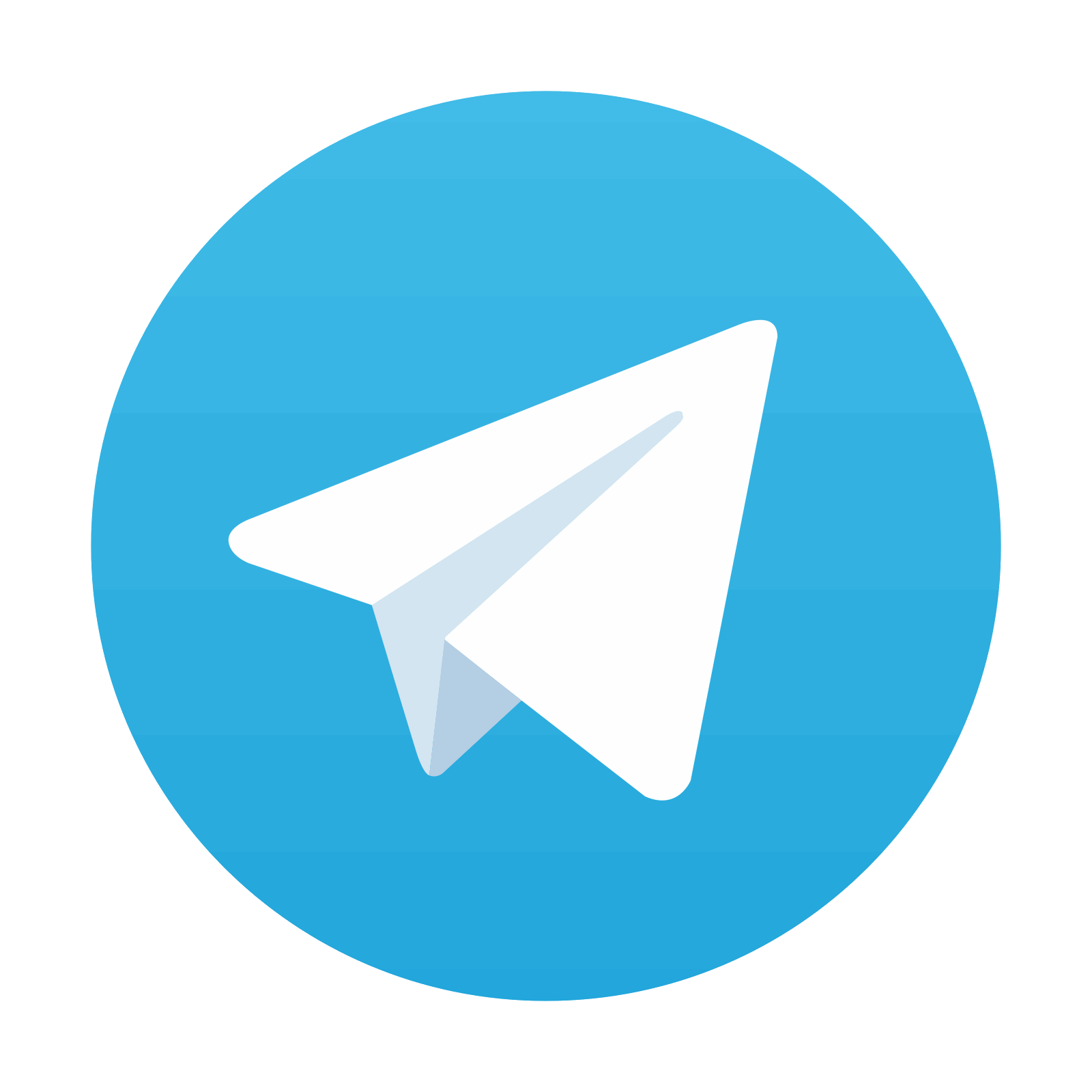
Stay updated, free articles. Join our Telegram channel

Full access? Get Clinical Tree
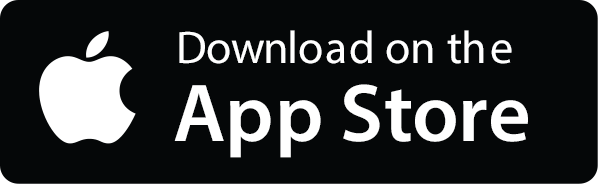
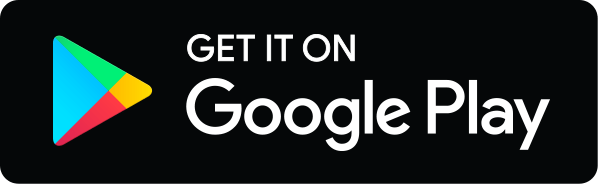