14 High-performance capillary electrophoresis
Applications
• An accurate and precise technique for quantitation of drugs in all types of formulations.
• Particular strength in quality control of peptide drugs.
• Highly selective and is very effective in producing separation of enantiomers.
• Very effective for impurity profiling due to its high resolving power.
• Very effective for the analysis of drugs and their metabolites in biological fluids.
Introduction
Electrophoresis
where v is the ion velocity, μe is the electrophoretic mobility and E is the applied electric field.
where q is the charge on the ion and E is the applied electric field, i.e. the greater the charge on an ion the more rapidly it migrates in a particular electric field.
where η is the viscosity of the medium used for electrophoresis, r is the ion radius and ν is the ion velocity.
When the frictional drag and the electric field experienced by the ion are equal:
substituting this expression into Equation 1:
If the applied electric field is increased beyond the point where the drag and electric field are equal, the ion will begin to migrate. From Equation 2 it can be seen that:
(i) The greater the charge on the ion, the higher its mobility.
(ii) The smaller the ion, the greater its mobility. Linked to this, since Equation 2 applies to a spherical ion, the more closely an ion approximates to a sphere, i.e. the smaller its surface area, the greater its mobility. This effect is consistent with other types of chromatography.
Thus the mobility of an ion can be influenced by its pKa value – the more it is ionised the greater its mobility – and its molecular shape in solution. Since its degree of ionisation may have a bearing on its shape in solution, it can be seen that the behaviour of analytes in solution has the potential to be complex. For many drugs the manipulation of the pH of the electrophoresis medium should have a marked effect on their relative mobilities. Thus one would predict that the electrophoretic separation of the two bases (morphine and codeine), which are of a similar shape and size but have different pKa values, would increase with pH. If we assume that morphine and codeine possess the same mobilities at full charge, then Figure 14.1 indicates how their mobilities vary with pH. As can be seen in Figure 14.1, the biggest numerical difference in mobility is when the pH = pKa of the weaker base, although the ratio of the mobilities goes on increasing with pH, e.g. at pH 8.9 the ion mobility of codeine is ca two times that of morphine.
EOF
The wall of the fused-silica capillary can be viewed as being similar to the surface of silica gel, and at all but very low values the silanol groups on the wall will bear a negative charge. The pKa of the acidic silanol groups ranges from 4.0 to 9.0 and the amount of negative charge on the wall will increase as pH rises. Cations in the running buffer are attracted to the negative charge on the wall, resulting in an increase in positive potential as the wall is approached. The effect of the increased positive potential is that more water molecules are drawn into the region next to the wall (Fig. 14.2). When a potential is applied across the capillary, the cations in solution migrate towards the cathode. The cations in a concentrated layer near to the capillary wall exhibit a relatively high mobility (conductivity) compared to the rest of the running buffer and drag their solvating water molecules with them towards the cathode, creating EOF. The rate of EOF is pH dependent since the negative charge on the silanol groups increases with pH, and between a pH of 3 and 8 the EOF increases about 10 times. The EOF decreases with buffer strength since a larger concentration of anions in the running buffer will reduce the positive potential at the capillary wall and thus reduce the interaction of the water in the buffer with the cations at the wall.
The flow profile obtained from EOF is shown in Figure 14.3 in comparison with the type of laminar flow shown in HPLC. The flat flow profile produces narrower peaks than are obtained in HPLC separations and is a component in the high separation efficiencies obtained in capillary electrophoresis (CE).
Migration in CE
The existence of EOF means that all species, regardless of charge, will move towards the cathode. In free solution, cations move at a rate determined by their ion mobility + the EOF. Neutral compounds move at the same rate as the EOF and anions move at the rate of the EOF – their ion mobility; the rate of EOF towards the cathode exceeds the rate at which anions move towards the anode, by approximately ten times. A typical separation could be viewed as shown in Figure 14.4. The cations in solution migrate most quickly, with the smaller cations reaching the cathode first; the neutral species move at the same rate as the EOF; and the anions migrate most slowly, with the smallest anions reaching the cathode last. The EOF is useful in that it allows the analysis of all species but it adds complexity to the method in that it needs to be carefully balanced against ion mobility. Table 14.1 shows how EOF can be controlled using different variables and illustrates some of the complexity of CE relative to HPLC.
Table 14.1 Variables affecting EOF
Variable | Effects on EOF | Comments |
Buffer pH | EOF increases with pH | Most convenient method for controlling EOF but has to be balanced against effects on the charge on the analyte |
Buffer strength | EOF decreases with increasing buffer strength | (i)Increased ionic strength means increased electric current flow through the capillary, which can cause heating |
(ii)At low ionic strength more sample absorption onto the capillary walls occurs | ||
(iii)Low buffer concentrations reduce sample stacking following injection | ||
Electric field | Increased electric field increases EOF | Lowering the applied electric field may reduce separation efficiency and raising the field strength may cause heating |
Temperature | Increased temperature decreases viscosity and thus increases flow | Easy to control |
Organic modifier | Changes potential at capillary wall – the dielectric constant of the running buffer and the viscosity. Usually decreases EOF | Complex effects – can be useful but best determined experimentally |
Surfactant | Absorbs onto the surface of the capillary wall | (i)Cationic surfactants have a high affinity for the silanol groups and thus block access by the smaller cations in solution, reducing EOF. At high concentration they form a double layer, giving the capillary wall an effective positive charge and causing EOF to reverse flow towards the anode |
(ii)Anionic surfactants reduce the access of the smaller ions in the running buffer to the positive potential at the wall thus increasing the zeta potential and thus EOF | ||
Covalent wall coating | Can raise or lower EOF depending on the coating | (i)Neutral coatings reduce negative charge of the capillary wall thus reducing EOF |
(ii)Ionic coatings will have marked effects on EOF |
Instrumentation
A schematic diagram of a capillary electrophoresis instrument is shown in Figure 4.5. The fundamentals of the system are as follows:
(i) Injection is commonly automated and is usually accomplished by pressuring the vial containing the sample with air.
(ii) Having loaded the sample, the capillary is switched to a vial containing running buffer. The flow rate of the running buffer through the capillary is in the low nanolitres/min range.
(iii) The capillaries are like those used in capillary gas chromatography with a polyamide coating on the outside. The length of the capillaries used is 50–100 cm with an internal diameter of 0.025–0.05 mm. They are generally wound round a cassette holder so that they can simply be pushed into place in the instrument.
(iv) At the detector end, the capillary has a window burnt into it so that it is transparent to the radiation used for detection of the analyte.
(v) The most commonly used detector is a diode array or rapid-scanning UV detector, although fluorometric, conductimetric and mass spectrometric detectors are available.
Control of separation
< div class='tao-gold-member'>
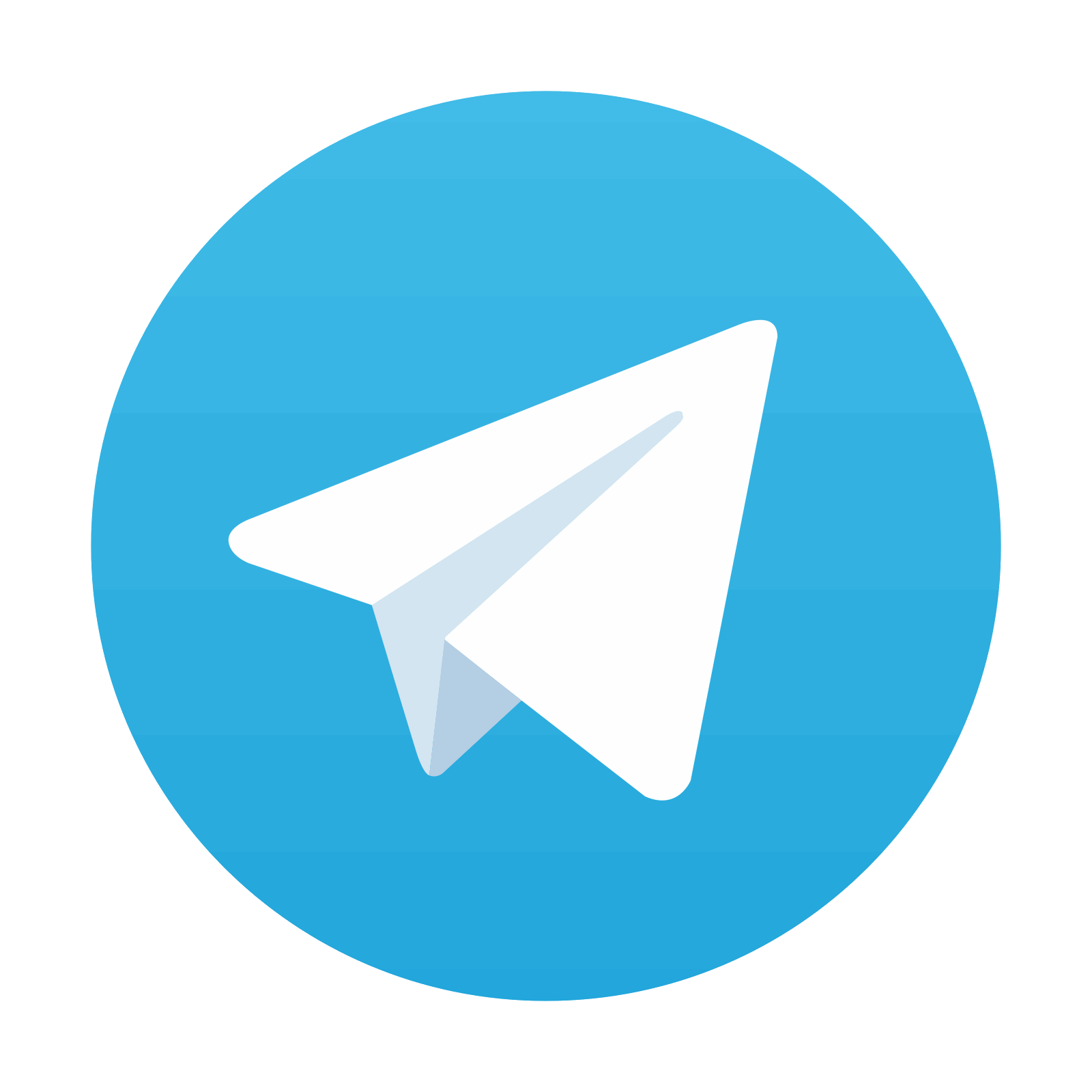
Stay updated, free articles. Join our Telegram channel

Full access? Get Clinical Tree
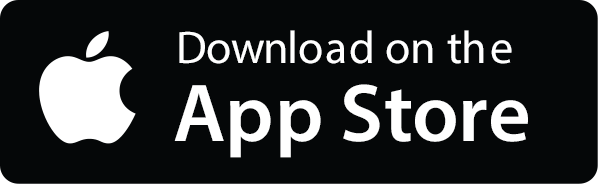
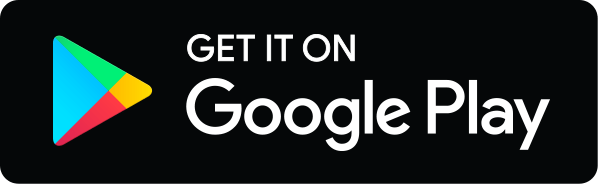