Chapter 7 Gastrointestinal Physiology
I. Structure and Function of the Gastrointestinal Tract
A. Functional anatomy
1. Overview
• The gastrointestinal (GI) tract is essentially a hollow digestive tube that extends from the mouth to the anus.
• Secretions from accessory digestive structures such as the salivary glands, pancreas, and liver empty into this tube and are essential for efficient digestion and absorption.
• The digestive tract can be subdivided into three sections based on embryologic origin and vascular supply (Fig. 7-1).
• The foregut extends from the esophagus to the second part of the duodenum and is supplied by the celiac artery.
• The midgut extends from the second part of the duodenum to the splenic flexure of the colon and is supplied by the superior mesenteric artery.
• The hindgut extends from the splenic flexure of the colon to the anus and is supplied by the inferior mesenteric artery.
2. Layers of the gut wall
• Throughout most of the GI tract, the gut wall is composed of four layers; from inside to outside, these are the mucosa, submucosa, muscularis propria, and serosa (Fig. 7-2).
a. Mucosa
• The mucosa is composed of three distinct layers: the mucosal epithelium, the lamina propria, and the muscularis mucosae.
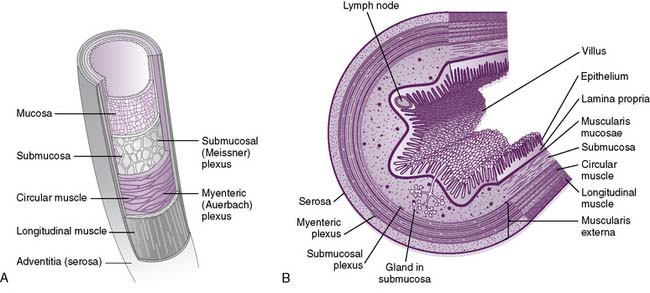
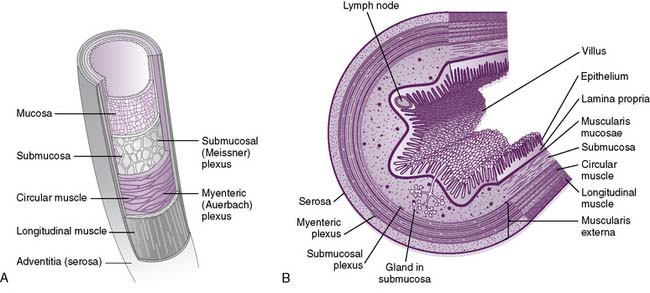
7-2 Two different views of the layers of the gut wall.
(B, From Koeppen BM, Stanton BA: Berne and Levy Physiology, 6th ed. Updated ed. Philadelphia, Mosby, 2010, Fig. 26-2.)
• In contrast, columnar mucosal epithelium is present in the rest of the GI tract, except for the rectum, where it changes back to squamous at the dentate line.
• In the small intestine, there are folds of cells in the mucosa (villi) and projections on individual cells (microvilli), which increase the surface area for absorption (Fig. 7-3).
c. Muscularis propria (externa)
• Thick muscular layer composed of inner circular and outer longitudinal muscle layers that extend the entire length of the intestinal tract
d. Serosa
• Outermost cellular membrane present in most of the intestinal tract that is continuous with the peritoneal lining
3. Neural regulation of the gastrointestinal tract
• Enteric nervous system
b. Submucosal (Meissner) plexus
• Extrinsic regulation: autonomic nervous system (Fig. 7-4)
a. The parasympathetic nervous system (PNS) generally promotes digestion and absorption by stimulating GI secretions and peristalsis while inhibiting sphincter muscle contraction.
b. In contrast, the sympathetic nervous system (SNS) generally inhibits digestion and absorption, stimulates sphincter muscle contraction, and causes vasoconstriction in the splanchnic circulation (Table 7-1).
TABLE 7-1 Effect of the Autonomic Nervous System on the Gastrointestinal Tract
Effect | Parasympathetic | Sympathetic |
---|---|---|
Motility | + | − |
Sphincter tone | − | + |
Secretion | + | − |
Vasoconstriction | No effect | + |
• Anatomy of reflex loops
a. Local reflexes, such as the gastrocolic reflex, involve afferent and efferent arcs that are contained entirely within the enteric nervous system.
b. Vagovagal reflexes, such as receptive relaxation of the stomach in response to swallowing of food, involve afferent fibers from the gut that travel through the vagus nerve to the brainstem and then back to the gut through the vagus nerve.
c. Afferent fibers from the gut may travel to the spinal cord (or sympathetic ganglia) and then back to the gut.
B. Gastrointestinal functions
1. Motility
• Electrical basis for intestinal motility: slow waves
a. Similar to cardiac nodal cells, intestinal smooth muscle cells (SMCs) have a constantly changing resting membrane potential.
b. Rather than constantly generating action potentials, intestinal smooth muscle cells are subject to undulating oscillations in resting membrane potential.
c. These slow waves have a resting membrane potential that varies between approximately −60 and −30 mV (Fig. 7-5).
d. In the absence of spike potentials, the slow waves are unable to elicit smooth muscle contractions, except in the stomach.
• Types of contractions
a. Peristalsis
• Distension of the gut wall by a food bolus triggers reflexive contractions of smooth muscle (mainly the inner circular and outer longitudinal muscle layers) that push the food bolus forward along the intestinal tract.
• This forward propulsion requires smooth muscle contraction just proximal to the food bolus and simultaneous relaxation just distal to the food bolus (Fig. 7-6A).
b. Segmentation
• The primary function of segmentation is to assist digestion by promoting mixing of the intestinal contents (e.g., food, digestive enzymes, bile salts).
• This is achieved by simultaneous contractions both proximal and distal to the food bolus (Fig. 7-6B).
2. Digestion
• Digestion entails the enzymatic hydrolysis of macromolecules (fats, carbohydrates, and proteins) into smaller compounds.
II. Salivation and Mastication
A. Salivation
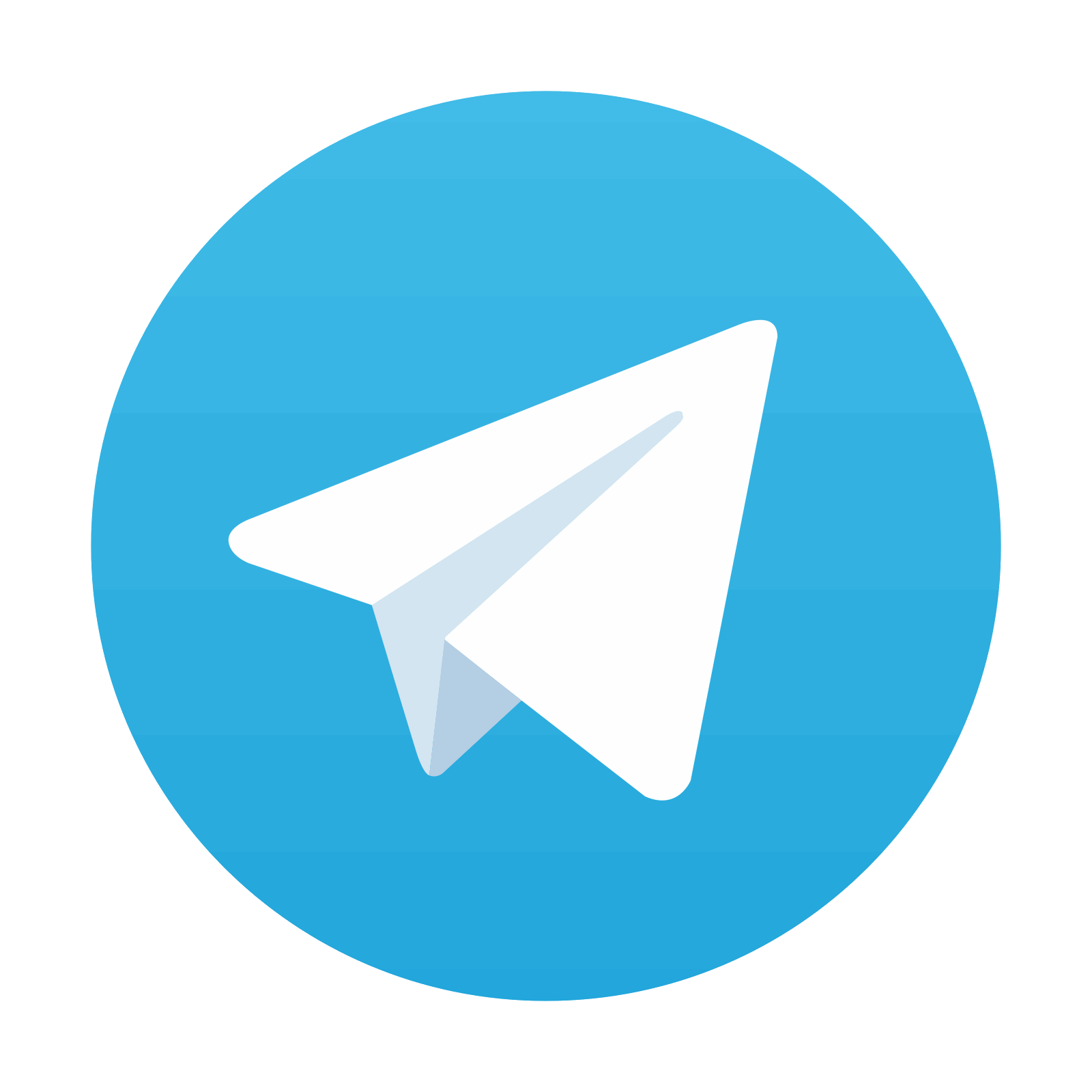
1. Composition and functions of saliva
• Salivation plays several important roles in facilitating digestion in addition to its vital role in maintaining oral health (Table 7-2).
TABLE 7-2 Composition and Function of Saliva
Component | Primary Function |
---|---|
Potassium bicarbonate | Neutralizes bacterial acid, preventing digestion of tooth enamel and dentine (prevents cavities) |
Lingual lipase | Initiates lipid digestion |
Salivary amylase | Initiates carbohydrate digestion |
Mucins | Lubricate food bolus, are primary determinant of viscosity |
Lysozyme | Initiates bacterial lysis |
Immunoglobulins | Offer immune protection |
2. Mechanism of saliva production
• Secretions from the salivary acinus are very similar in tonicity to plasma (i.e., they are isotonic).
• The salivary ducts are relatively impermeable to water entry, and sodium is continually reabsorbed.
3. Types of salivary glands
• Serous glands (e.g., parotid), which are primarily composed of serous cells, secrete a nonviscous saliva containing water, electrolytes, and enzymes.
• Mixed glands (submandibular, sublingual), which are composed of serous and mucous cells (Fig. 7-8), secrete a viscous saliva rich in mucin glycoproteins.
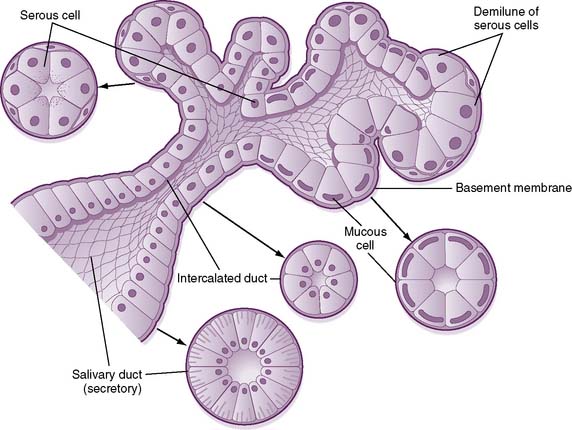
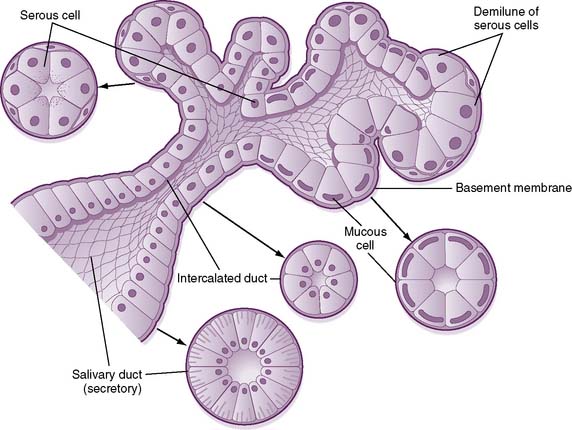
7-8 Structure of a mixed salivary gland, showing both serous and mucous cells.
(From Koeppen BM, Stanton BA: Berne and Levy Physiology, 6th ed. Updated ed. Philadelphia, Mosby, 2010, Fig. 27-1.)
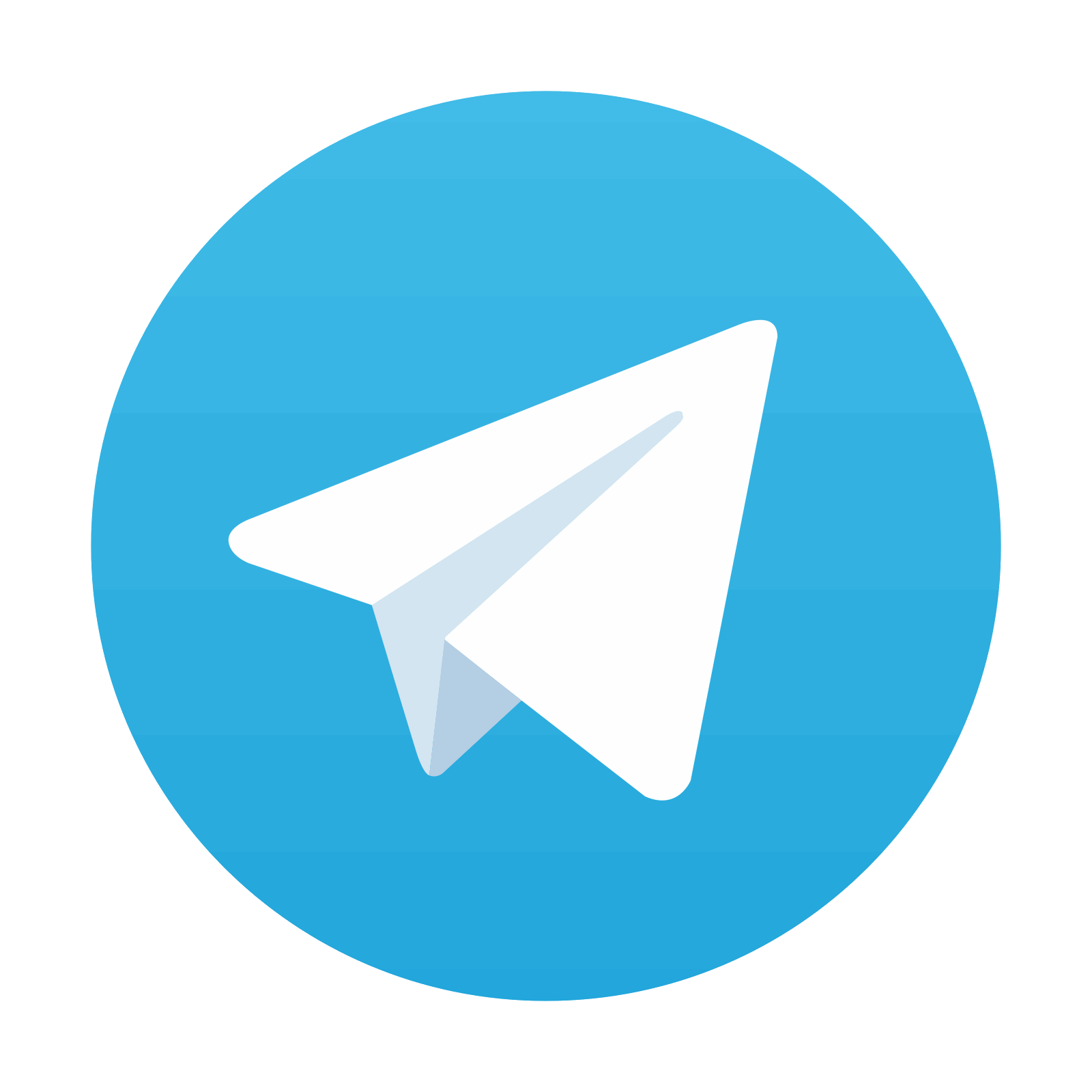
Stay updated, free articles. Join our Telegram channel

Full access? Get Clinical Tree
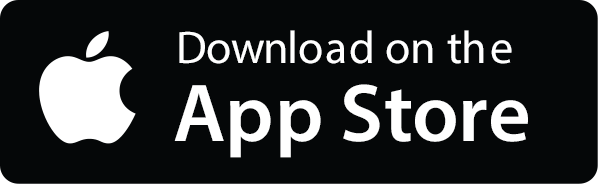
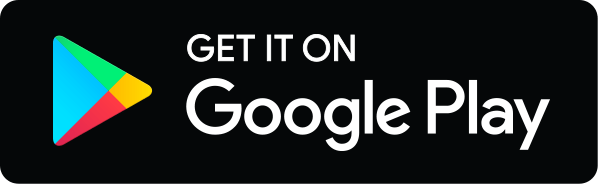