4 Felipe J.O. Varum1,2 and Abdul W. Basit1 1UCL School of Pharmacy University College London UK 2Tillotts Pharma AG Switzerland The architecture of the gastrointestinal tract is finely arranged in that it provides the necessary machinery involved in the digestion of food and also provides protection to the underlying epithelium from the chemical and bacterial aggressors present in the intestinal lumen. The mechanisms behind the protection of the underlying epithelium are multiple and act in a synergistic manner. Notably, the gastrointestinal mucus layer, covering most of the gut, plays a central role in this process. The dynamic nature of the mucus layer is essential to promote an adequate barrier from the luminal contents, but allowing absorption of nutrients, and also to facilitate propulsion of the products of food digestion through the gut [1]. Considering oral drug delivery, it is essential to bear in mind the inter- and intra-individual variability in gastrointestinal physiology, which is also amplified by gender and age differences [2]. One such variable feature is gastrointestinal transit. The transit of oral dosage forms through the gastrointestinal tract is highly variable, most notably in terms of gastric emptying and colonic transit, and is also affected by the characteristics of the dosage form (single vs multiple units) [3]. Interestingly, the often cited 3–4 hours of transit of formulations through the small intestine can be misleading, as it hides a large intra-individual variation (Figure 4.1). Therefore, the time that a dosage form spends within the gut or at a specific region ultimately affects the oral drug bioavailability of drugs administered through modified release dosage forms, either sustained or delayed release [4]. Figure 4.1 Small intestinal transit time of nondisintegrating pellets in one subject on eight different occasions. The grey area represents the often stated transit time of formulations through the small intestine. Reprinted with permission from [4]. Copyright (2008) Elsevier Ltd. All rights reserved. The gastrointestinal mucus layer provides a platform for adhesion of dosage forms in the gastrointestinal mucosa – a concept referred as mucoadhesion. The mucoadhesion of formulations to a specific region of the gastrointestinal tract has the potential to increase residence time and, ultimately, contribute to normalize and improve oral drug bioavailability [5]. This concept is further explored in great detail in other chapters of this book. The main functions of the gastrointestinal tract are to absorb nutrients from food ingestion and to remove metabolic end products and undigested material. To afford this, a plethora of processes needs to take place in a balanced way, such as digestion, secretion, motility, absorption and excretion. The gastrointestinal tract is commonly divided into the oral cavity, oesophagus, stomach, small intestine and large intestine. The gastrointestinal wall is composed of four different layers: the mucosa, the submucosa, the muscularis and the serosa. The gastrointestinal tract mucosa can be further subdivided into three main layers: the muscularis mucosa, lamina propria and the epithelium. The epithelium of the gastric mucosa is composed of mucus producing cells, parietal cells (responsible for acid secretion), chief cells (secretion of pepsinogen) and enteroendocrine cells (which secrete various hormones and messengers). One type of enteroendocrine cells are the G-cells that secrete gastrin, further stimulating the secretion of acid and other enzymes by the gastric mucosa to help in the digestion process [6]. The epithelial cells of the small and large intestinal mucosa are designated by enterocytes, which are responsible for absorption. However, other cell types are also present, such as goblet (mucus producing) and other enteroendocrine cells. A blanket of mucus covers most of the gastrointestinal tract mucosa, providing lubrication and protective features [7]. Mucus also establishes a first barrier for absorption through the gastrointestinal tract [8,9]. Briefly, absorption can occur at different rates and extents depending on the region. For instance, drug absorption through the oesophageal epithelium is unlikely due to the presence of a stratified squamous epithelium and slow blood flux; any premature drug release could, therefore, be harmful for the oesophageal epithelium [10]. In the stomach, the epithelial surface available for drug absorption is relatively small. A summary of the anatomical and physiological features of the human gastrointestinal tract is presented in Table 4.1. Table 4.1 Summary of the anatomical and physiological features of the human gastrointestinal tract. CFU – colony forming units, SD – standard deviation. Proximal – 66 Distal -197 Proximal -415 Distal -380 Fasted 13 (12) Fed 11 (26) The small intestine possesses more favourable absorption characteristics: a large absorptive area (120 m2) provided not only by the length but also by villi and microvilli; a good blood supply; lymphoid tissue (important in the absorption of lipophilic drugs) [11]. The colon also benefits from this lymphoid tissue, but has a much lower absorptive capacity than the small intestine due to its lower surface area, as presented in Table 4.1. The high viscosity of the colonic contents and the lower availability of fluid may limit the dissolution of drugs, particularly poorly soluble drugs [12]. However, the potential limitations for drug absorption through the colonic mucosa may be offset by some physiological advantages. The lower digestive enzymatic activity relative to the upper gut suggests its potential for peptide and protein drug delivery, and additionally as a site for vaccination [13]. The lower levels of mucosal metabolic enzymes (cytochrome P450) in the colon, relative to the small intestine, may lead to improved drug bioavailability [14]. Additionally, the levels of efflux transporters compared to the small intestine, for example P-glycoprotein, are lower in the colon, which may also contribute to improved oral drug bioavailability of drugs that are substrates for these transporters [15]. Mucus is ubiquitous in the gastrointestinal tract and constitutes a dynamic biophysical barrier between the lumen and the underlying epithelium. Besides the gastrointestinal tract, mucus is also present in several other mucosal surfaces, such as in the vaginal, rectal, nasal and ocular surfaces. Gastrointestinal mucus is mainly produced by goblet cells intercalated between the enterocytes in the small and large intestinal mucosa. Mucus is composed of water (95%), mucin (glycoproteins which provide the gelling and viscoelastic properties), lipids, proteins, sloughed epithelial cells and inorganic salts [16]. Glycoproteins (mucins) have high molecular weights (1–40 × 106 Daltons) and are comprised of a protein core (800 amino acid residues), around 60% of which is attached to oligosaccharide branches (2–22 sugars in length). The side chains are composed of alternating N-acetylglucosamine and galactose residues and have different degrees of branching. In the intermediate positions appear residues of ester sulfates while fucose (a saccharide moiety) and sialic acid are found at terminal ends [7]. The sialic acid and sulfate residues are fully ionized at pH >2.6, which confers a negative charge to the mucin molecule. Sulfation of mucin oligosaccharides occurs predominantly in colonic mucins and has been shown to contribute to an increase in the resistance of mucus to degradation by colonic bacteria [17]. The oligosaccharide chains contain many acid groups, which are able to establish hydrogen bonds and charge interactions [18]. Mucins present the same main structure in different regions of the gastrointestinal tract but genetic and biochemical differences, particularly in the side chains, are relevant and are introduced below. Furthermore, mucins can be involved in intermolecular interactions through disulfide bounds formed in the nonglycosylated regions (Figure 4.2). Figure 4.2 Schematic representation of the mucin macromolecule and intermolecular bounding (disulfide bridges). There are two different types of mucins: secreted mucins and cell bounded mucins. The latter are mainly involved in cell signalling and are not in the focus of this chapter. Human mucins are expressed by eleven genes, namely MUC1–4, MUC5AC, MUC5B, MUC6–8 and MUC11–12. Gel forming mucins are expressed by MUC2, MUC5AC, MUC5B and MUC6 [19]. Expression of these mucins in the gastrointestinal tract varies in different regions. MUC5AC and MUC6 are expressed in the stomach, MUC2 is produced by goblet cells from duodenum to colon and Brunner’s glands in duodenum express MUC6 [20]. Human gastric antral mucus is composed predominantly of neutral mucins whereas mucins in the human caecum, ascending and descending colon were shown to be stained purple/blue, indicating high proportions of acidic mucins [21]. An acidic gradient along the large intestine was previously reported, where glycoproteins were mainly sialated and sulfated [22]. In rat, gastric mucins are predominantly acidic. Interestingly, a similar pattern of gastric and large intestinal mucin glycosylation was observed in the pig mucosa and in the rabbit mucosa [23]. This regional selectivity in glycosylation has also been reported in rats, where differences between the small and large intestine were found, besides differences between rat strains [24]. The neutral mucins, which predominate in the stomach, provide a protective role against acid secretion and enzymatic degradation by pepsin. The presence of sialic acid (sialomucins) and sulfate (sulfomucins) residues, particularly in the large intestine, have been linked with the dense bacterial population, providing protection against mucus degradation [25]. This is particularly relevant since the gradient in acidic mucin distribution along the colon is accompanied by a higher bacterial density in the distal regions. Sulfomucins and sialated mucins have been shown to contribute to the viscoelastic and lubrication properties of mucus [26]. Besides mucin, which is the main component responsible for the structure and functions of mucus, a number of other components are present within the mucus layer. Mucus is composed of water (95%), mucin (glycoproteins which provide the gelling and viscoelastic properties), lipids, proteins, sloughed epithelial cells and inorganic salts [27]. Amongst the main important proteins secreted into the mucus layer are secretory immunoglobulin A (SIgA), lysozyme, lactoferrin and trefoil peptides [28,29]. SIgA is secreted by the epithelial cells into the mucus layer and the lumen, where it can bind to bacteria, antigens and toxins, avoiding their attachment to the epithelial surface and the triggering of an infection/inflammation process. Lysozyme (bound to sialic acid residues) and lactoferrin also contribute to the overall protective functions of the mucus layer through multiple mechanisms [28]. Trefoil peptides are co-secreted along with mucus by mucus-producing cells in the gastrointestinal tract and have been involved in cell migration and cell repair after damage. These molecules are mainly localized in the adherent mucus layer and contribute to the protective properties of the mucus layer by decreasing permeability of hydrogen ions in the stomach and also increasing mucus viscosity [29]. Also lipids and fatty acids can be found noncovalently or covalently bound to mucins [27]. The goblet cells (mucus-producing cells) increase in number distally in the gastrointestinal tract [30]. The goblet cells migrate from the crypts to the villi, a process accompanied by maturation of the mucin-filled vesicles. This migration process usually takes 4–6 days in the human intestinal mucosa. The synthesis of mucin starts with the formation of the peptide core through assembly of the respective aminoacids in the rough endoplasmatic reticulum, followed by the transport through the smooth endoplasmatic reticulum to the Golgi apparatus. Here, the peptide core is highly glycosylated. For instance, sulfation in the Golgi apparatus occurs at higher extension in the goblet cells from the colonic than from the duodenal mucosa [30]. During their movement from the Golgi apparatus to the cell apex, the mucin-containing vesicles coalesce forming bigger vesicles (and the cells become smaller and more columnar), which are accumulated and subsequently discharged into the lumen by a slow and continuous process or by apocrine release after a mechanical or chemical stimulus [31]. Before discharge, mucin granules form a packed structure in the goblet cell (Figure 4.3), delimited by a layer of cytoplasma, designated theca, which is also composed of a cytoskeletal network, which is important in the mucin secretion process. Figure 4.3 Process of maturation of goblet cells during migration to the epithelial surface. Reproduced from Radwin et al., 1990, with permission. (a) Dark stained goblet cells in the colonic crypt; (b) representation of the goblet cell at the base of the crypt; (c) representation of the goblet cell at the top of the crypt, pyramidal shape still present but with lower volume; (d) representation of the goblet cell at the epithelial surface, the apical mucin granules are tightly packed and cell lost volume and shape. Reproduced with permission from [32]. Copyright © 1990, Wiley.
Gastrointestinal Mucosa and Mucus
4.1 Introduction
4.1.1 General Gastrointestinal Physiology
Length (m)
Surface area (m2)
pH
Bacteria levels (CFU/g contents)
Redox potential (mV)
Fasted transit time of a tablet (pooled data, hours)
Fluid volumes (ml)
Total (Range)
Free (SD)
Stomach
0.2
0.1
Fasted 0.8–2.0
102
+ 200
0–2
118 (11–233)
Fasted 45 (18)
Fed 4.0–5.0
Fed 686 (93)
Small intestine
7
120
104–107
Jejunum 0.5–2.0
206 (60–352)
Fasted 105 (72)
Ileum 0.5–2.5
Fed 54 (41)
Ileocaecal junction 0–12
Large intestine
1.5
0.3
6.4–7.0
1011–1012
0–72
187
4.2 The Gastrointestinal Mucus
4.2.1 What is Mucus?
4.2.2 Mucus Composition
4.2.2.1 Glycoproteins (Mucins)
Mucins Expression
Mucins Classification and Distribution Along the Gastrointestinal Tract
4.2.2.2 Nonglycoproteic Components of Mucus
4.2.3 Anatomy of Goblet Cells and Mucin Biosynthesis
4.2.4 Regulation of Mucus Secretion
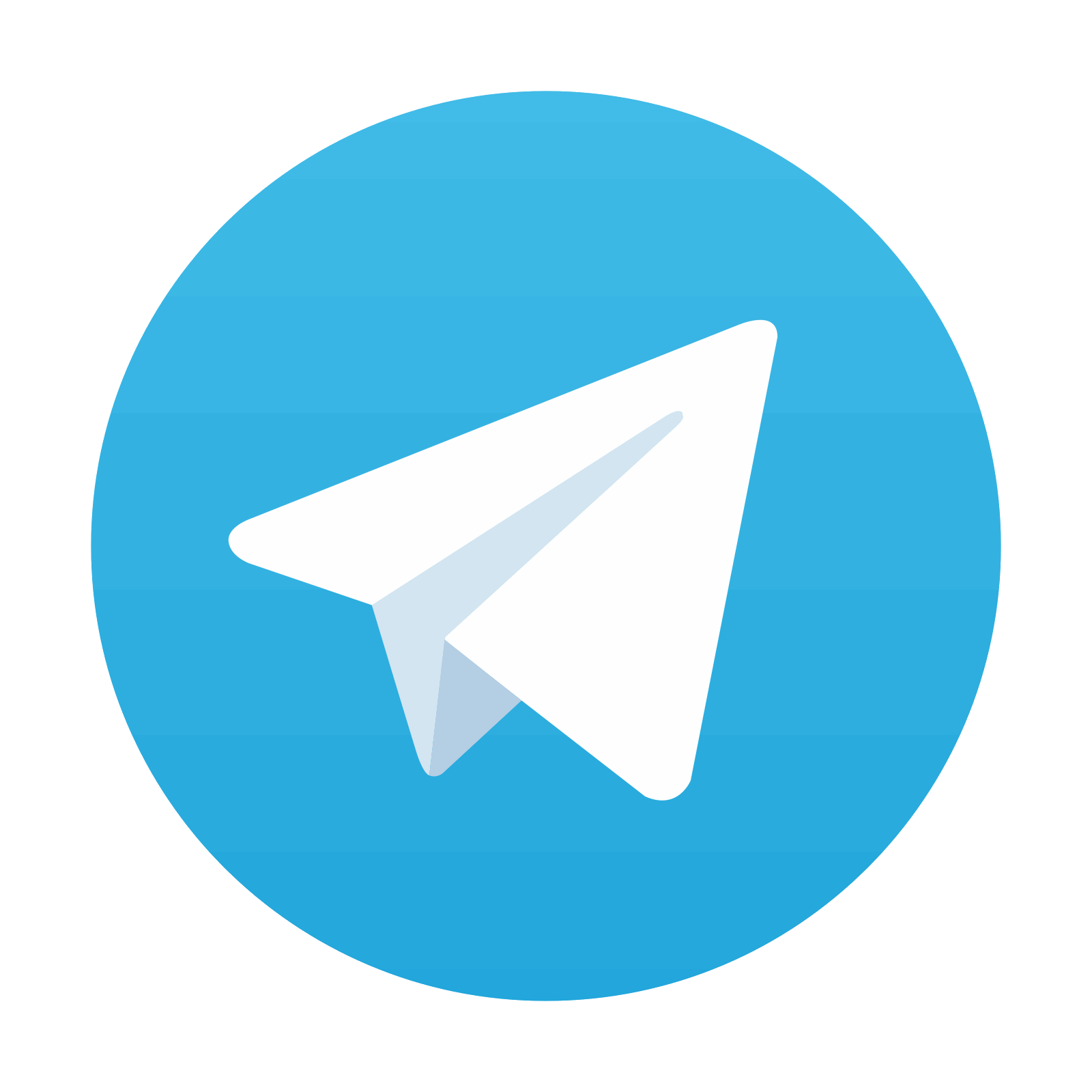
Stay updated, free articles. Join our Telegram channel

Full access? Get Clinical Tree
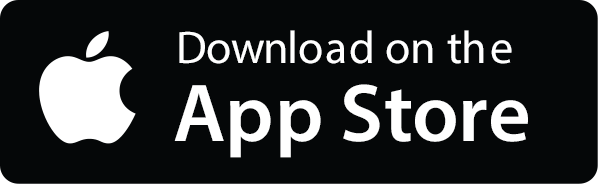
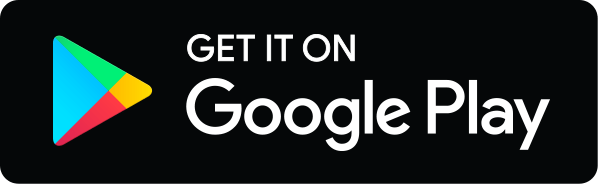