Chapter 5
G protein-coupled receptors
5.1 Overview
As stated earlier (slide 1.2.3), G protein-coupled receptors (GPCRs) are the single most important class of drug targets in the human body. It is therefore appropriate to examine and understand them in some detail.
The human genome contains genes for several hundred GPCRs. For a bit less than half of these, the ligands and physiological functions are not yet known. Once these “orphan” receptors will have become “deorphanized”, it is likely that some of them will become drug targets also.
5.1.1 Drugs that act on G protein-coupled receptors: Some examples
Notes: These few selected examples illustrate the wide-ranging and diverse roles of GPCRs in physiology and pharmacology.
5.1.2 GPCR structure
Notes: All GPCRs (with the exception of rhodopsin) are located in the cytoplasmic membrane. The snake diagram on the left shows the arrangement of the seven transmembrane helices: the N-terminus faces outward, and the C-terminus is located inside the cell. The diagram also indicates the location of several amino acid residues that are conserved within the largest subfamily of GPCRs and play key roles for receptor activation. These include the DRY motif at the cytoplasmic end of helix 3, the proline and tryptophan residues in helix 6, and the short C-terminal helix 8.
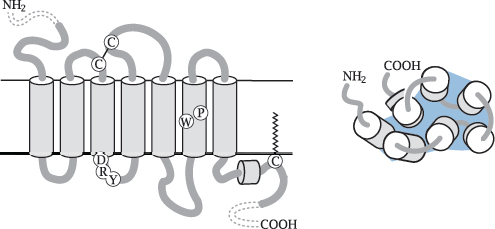
The schematic on the right shows the view from the extracellular side. The helices are arranged in an approximately circular fashion within the membrane plane. With many receptors, the crevice in the middle of the helices contains the ligand binding site, which is accessible from the extracellular side.
While the extracellular N-terminal domain is short in most GPCR molecules, some receptors have larger N-terminal domains that may contain ligand binding sites or other functional features. We will see some examples later.
5.1.3 The G protein cycle
Notes: The G protein cycle comprises the activation and inactivation of a heterotrimeric G protein. The cycle starts when an agonist binds to the extracellular face of a GPCR (1), which changes the conformation of the GPCR molecule so as to also affect its intracellular surface. In this activated conformation, the GPCR binds a G protein (2). The α-subunit of the receptor-bound G protein releases a molecule of GDP, which had been left behind by a previous turn of the cycle, and then dissociates from the βγ-dimer. Both of the dissociated fragments are now free to seek out and bind to their respective effector proteins (4), which have various biochemical activities (see later).
After some time has elapsed, the GTP molecule bound to the Gα-subunit is cleaved by the built-in GTPase activity of that protein (5). This causes the α-subunit to leave its effector and to again associate with a Gβγ dimer (6). The inactive trimer then awaits another round of activation.
5.2 G protein binding and dissociation can be observed with GFP fusions
The experiments described in the following illustrate how the activities of GPCRs and G proteins can be observed within cells. These experimental methods are commonly used today; they were, however, not yet available in the early studies that actually determined how these proteins work.
5.2.1 Autocatalytic formation of the fluorophore in the Aequoria green-fluorescent protein
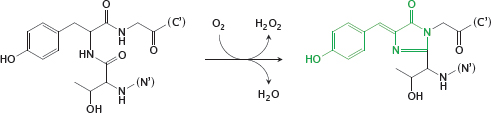
Notes: The great power of the green-fluorescent protein (GFP) is due to its entirely spontaneous formation of a fluorophore—it needs no help from any other protein, and therefore can easily be used in almost any type of cell or cellular compartment. It is typically used through translational fusion with proteins of interest.
While the wild-type GFP is excited with ultraviolet light, several mutants have been created that can be excited with visible light, and which can be combined with one another for fluorescence resonance energy transfer (FRET) experiments. In the mutant cyan-fluorescent protein (CFP), the tyrosine residue that becomes part of the fluorophore is replaced by tryptophan. The yellow-fluorescent protein (YFP) retains the unmodified fluorophore of GFP but contains a mutant tyrosine residue that engages in π-stacking interactions with the latter and changes its absorption and emission spectra.
5.2.2 FRET detection of G protein binding to adenosine receptors
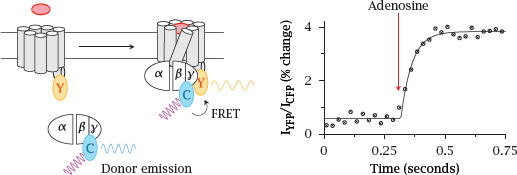
Notes: In this experiment, the yellow-fluorescent protein (Y) was translationally fused to the A2A adenosine receptor, and the cyan-fluorescent protein (C) to the γ-subunit of the cognate G protein (Gs). Addition of adenosine activates the receptor and triggers binding of Gs to the receptor. The ensuing FRET from CFP to YFP is detected through an increase in the ratio of YFP emission to CFP emission.
The observed change in the extent of FRET is fairly small, suggesting that only a small change occurs in the extent of the mutual association of receptor and G protein. Figure prepared from original data in [25].
5.2.3 FRET detection of G protein dissociation
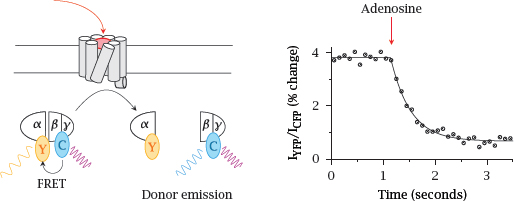
Notes: In this experiment, FRET between CFP and YFP was used to detect the dissociation of the heterotrimeric G protein. In this case, the extent of FRET decreases upon receptor activation. Again, the change in FRET is small.
Note the difference in time scales between this slide and the preceding one: Dissociation of the G protein is noticeably slower than its binding to the activated receptor. Figure prepared from original data in [25].
5.3 G protein effector mechanisms
For each of the three subunits of the heterotrimeric G proteins—α, β and γ—there are several subtypes, which may combine into heterotrimers in various permutations. However, overall, G proteins are less diverse than GPCRs; therefore, multiple types of GPCRs must converge upon the same G proteins and trigger the same intracellular responses.1 We will now look at the major intracellular signaling cascades triggered by different G proteins.
5.3.1 Adenylate cyclase
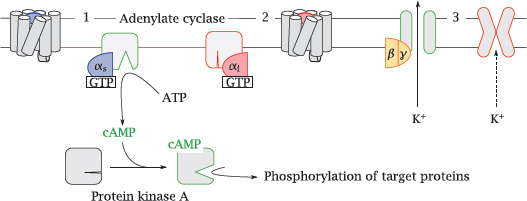
Notes: One important effector protein is adenylate cyclase. This membrane-associated enzyme converts ATP to cyclic AMP (cAMP), which is an allosteric activator of protein kinase A.
Adenylate cyclase is controlled by two different Gα subunits, which are activated by different GPCRs. The stimulatory α-subunit (Gαs, 1) activates the enzyme, whereas the inhibitory α-subunit (Gαi, 2) inhibits it.
An important effector molecule for Gβγ dimers are K+ channels of the Kir type (3). Opening of these channels will cause hyperpolarization of the cell membrane. In excitable cells, this will tend to reduce the level of activity.
5.3.2 The phospholipase C cascade
Notes: Another effector pathway activated downstream of many pharmacologically important GPCRs is the phospholipase C cascade. For example, the contraction of vascular smooth muscle cells downstream of α-adrenergic receptors (slide 2.3.4) or angiotensin receptors (slide 1.2.5) is mediated by this pathway.
In this cascade, the α-subunit of Gq stimulates phospholipase Cβ (1), which cleaves a specific membrane lipid, phosphatidylinositol-bisphosphate (PIP2), into two secondary messengers. The first one is diacylglycerol (DAG), a very hydrophobic molecule that remains associated with the cytoplasmic membrane, where it activates protein kinase C (2). Phosphorylation of target proteins by this kinase causes various downstream effects.
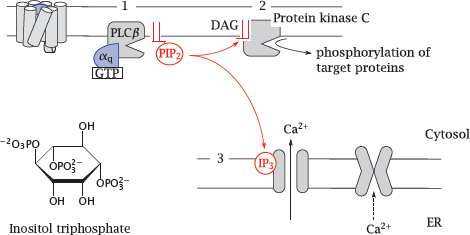
The other messenger released by phospholipase C is inositoltriphosphate (IP3). This molecule is water-soluble and travels through the cytosol to the ER membrane, where it activates a cognate calcium channel, named the IP3 receptor (3). Ca++ ions released from the ER into the cytosol activate calmodulin, which in turn will affect numerous calmodulin-dependent proteins. In smooth muscle, a calmodulin-dependent protein kinase phosphorylates myosin, which triggers contraction.
5.3.3 Summary of G protein effector mechanisms
Class | Effectors and Effects | Some activating GPCRs |
Gαs | Stimulation of adenylate cyclase (various types) | β-adrenergic. 5-HT4, 5-HT6, 5-HT7, D1, D5; ACTH |
Gαi/o | Inhibition of adenylate cyclase; activation of extracellular signal-regulated kinase (ERK) | α2-adrenergic, 5-HT1, D2, D3, D4 |
Gαq/11 | Stimulation of Phospholipase Cβ (various subtypes) | α-adrenergic, 5-HT2, H1, GABAB |
Gα12/13 | Indirect activation of RhoA GTPase and of phospholipase A2 | 5-HT4. AT1. protease-activated receptors |
Abbreviations: 5-HT, 5-hydroxytryptamine (serotonin); D, dopamine; AT, angiotensin; ACTH, adrenocorticotropic hormone; GABA, γ-aminobutyric acid.
Notes: The first three of the G proteins listed here were illustrated in the preceding two slides. The enumeration of activating receptors for each of these cascades is not exhaustive, but only intended to illustrate the convergence of different receptors on the same G proteins.
A single GPCR may interact with multiple G proteins, which may set of different downstream cascades. Occasionally, different receptor agonists may activate various downstream cascades to different extents (see slide 2.5.4).
5.4 Structure and function of GPCRs
Much experimental effort has been devoted to the study of the conformational changes that are at the heart of GPCR function. Many of these studies have been performed on rhodopsin. This molecule differs from other GPCRs in being activated not by ligand binding and dissociation, but instead by photoisomerization of its covalently bound retinal chromophore. However, it is the easiest GPCR molecule to obtain in abundance, and it was the first one to be crystallized, which made it an attractive model. Since it shares extensive homology with many GPCRs that are drug targets, it is also a credible and useful model.
Crystal structures of other GPCRs have now started to appear, and they will indubitably enhance our understanding and the precision of drug design. However, crystal structures are static, and other biophysical methods remain relevant for understanding the molecular movements and interactions.
5.4.1 Substance P and its competitive antagonist CP-96345
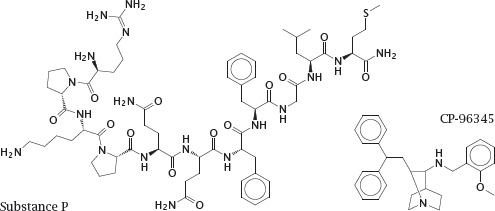
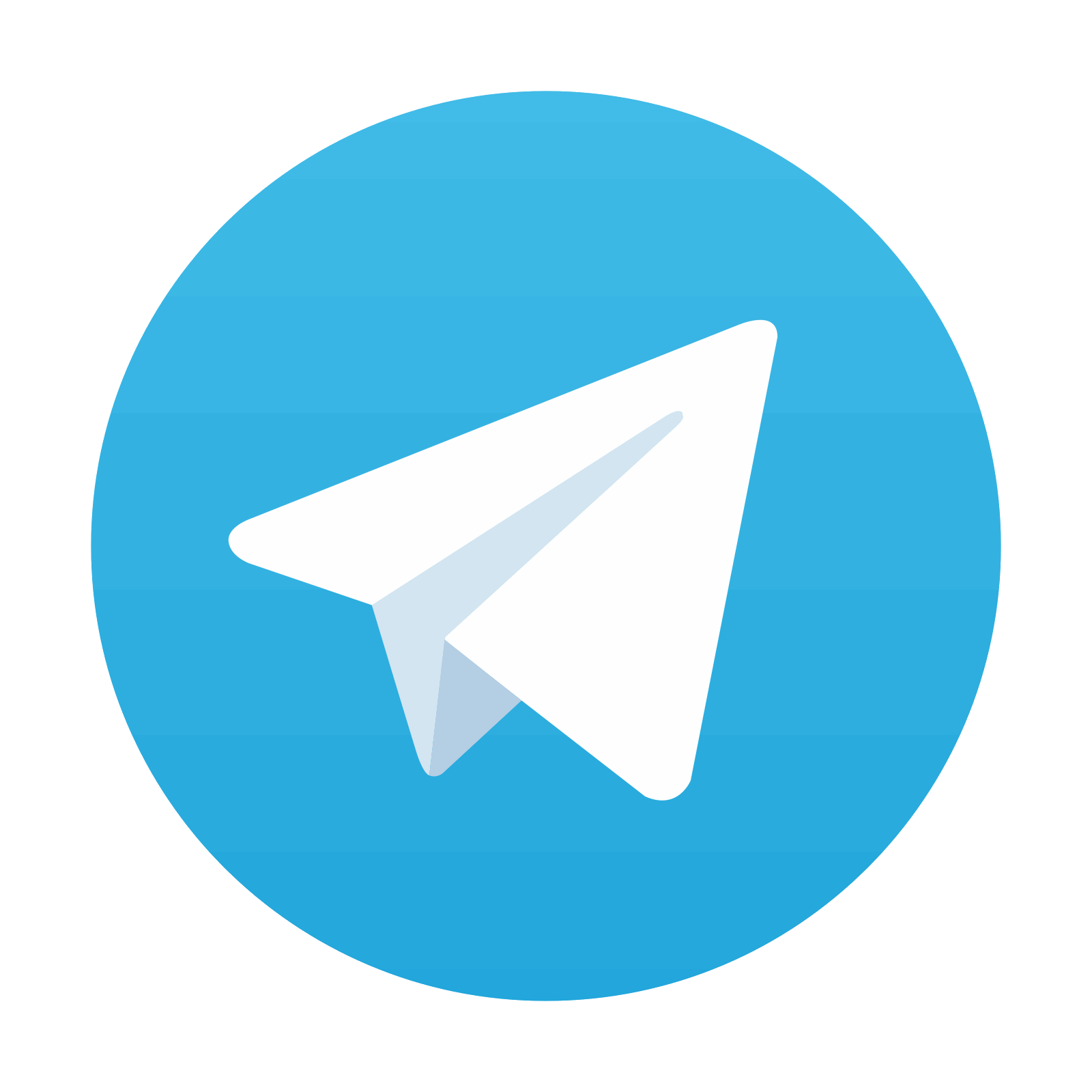