Fluid and Electrolyte Homeostasis and Imbalances
Linda Felver
Key Questions
• How do the compositions of plasma and interstitial fluids differ? How are they similar?
• What are the usual and pathologic routes of fluid exit from the body?
• What capillary-level mechanisms cause edema?
http://evolve.elsevier.com/Copstead/
The fluid of the body flows in arteries, veins, and lymph vessels; it is secreted into specialized compartments as diverse as joints, cerebral ventricles, and the intestinal lumen; it both surrounds and permeates the cells. Body fluid serves as a lubricant and as a solvent for the chemical reactions that we call metabolism; it transports oxygen, nutrients, chemical messengers, and waste products to their destinations; it plays an important role in the regulation of body temperature. Because the fluid within the body is so widespread and serves so many functions, it is not surprising that abnormalities in the volume, concentration, or electrolyte composition of body fluid cause clinical problems.
Disorders of fluid or electrolyte homeostasis occur as a result of many different pathophysiologic conditions. In severe cases, these disorders cause death. Although these disorders develop from many specific causes in different patient populations, these specific causes fall into general categories that arise from the principles of normal fluid and electrolyte homeostasis. This chapter first presents the principles of normal fluid homeostasis and then, building on that foundation, continues with a discussion of fluid imbalances. Similarly, it explains the principles of electrolyte homeostasis before presenting plasma electrolyte imbalances.
Body Fluid Homeostasis
The term body fluid, as used in this chapter, pertains to water within the body and the particles dissolved in it. The body fluid is contained in two major compartments: extracellular (outside the cells) and intracellular (inside the cells). In all age groups except infants, approximately two thirds of body fluid is intracellular.1 The other one third of body fluid is extracellular. Infants have more extracellular fluid than intracellular fluid; this proportion reverses within a few months as the infant grows. The extracellular fluid lies between the cells (interstitial compartment), in the blood vessels (vascular compartment), in dense connective tissue and bone, and in several minor compartments that are collectively termed the transcellular fluids (e.g., synovial, cerebrospinal, and gastrointestinal fluids). The major body fluid compartments are depicted in Figure 24-1.
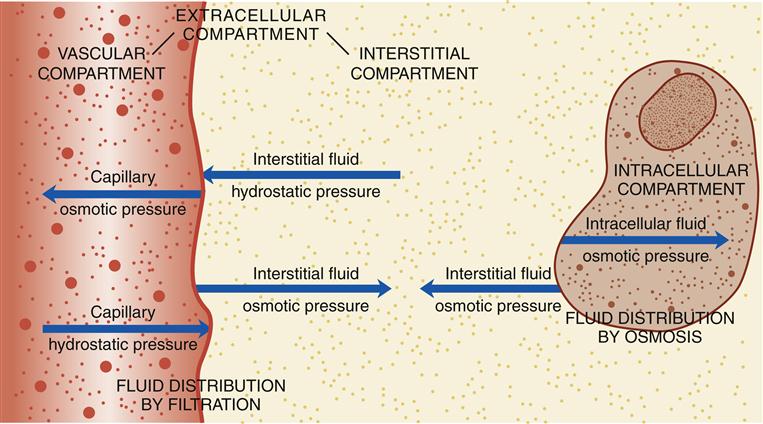
Fluid distribution between the vascular and interstitial compartments is the net result of filtration across permeable capillaries. The distribution of fluid between the interstitial and intracellular compartments occurs by osmosis rather than by filtration.
The fluids in the various body compartments have different compositions, although their total particle concentration is equal. The intracellular fluid is relatively rich in potassium and magnesium ions, inorganic and organic phosphates, and proteins. It is relatively low in sodium and chloride ions. In contrast, the extracellular fluid in the vascular and interstitial compartments is relatively rich in sodium, chloride, and bicarbonate ions and relatively low in potassium, magnesium, and phosphate ions. The vascular portion of the extracellular fluid contains many proteins, whereas the interstitial and transcellular portions of the extracellular fluid contain very few proteins. Most transcellular fluids are secreted by epithelial cells; their composition varies according to their function.
Total body water is the total amount of water in all fluid compartments. The percentage of body weight that is water varies according to a person’s age and proportion of body fat (Figure 24-2). A full-term newborn infant is about 75% water by weight. (Preterm infants have an even higher percentage of water.) This percentage decreases with age. In a standard adult man, body water is about 60% of body weight. The percentage is less (about 50%) in women because they have a greater proportion of body fat than men of the same weight. In obese adults, with a much larger proportion of body fat, less of the body weight is water. With normal aging there is a relative increase in body fat, so that in older men, 50% of the body weight is typically composed of water; in older women, it is even less.
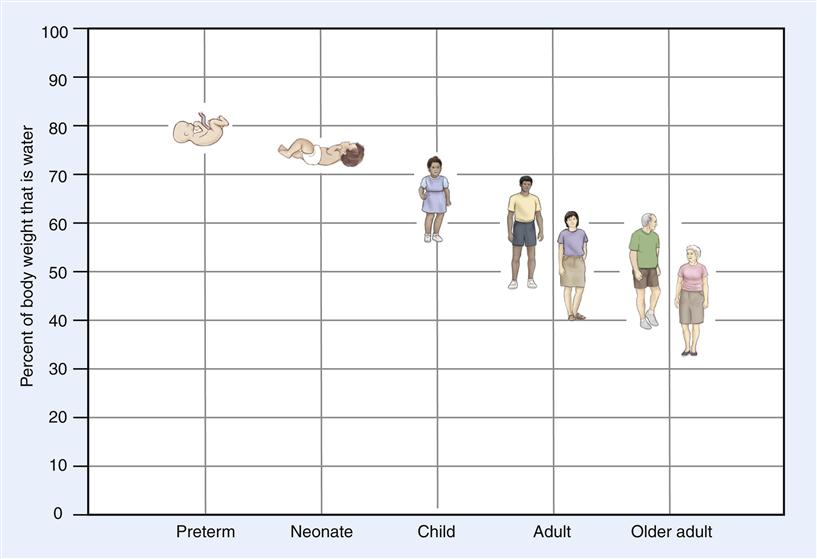
The percentage of body weight that is water is high in infancy and decreases with increasing age.
One liter of water weighs 1 kg (2.2 lb). Thus, a lean, middle-aged, healthy adult man who weighs 70 kg (154 lb) has approximately 42 L of body water. Of this amount, approximately 25 L is intracellular water. The approximately 17 L of extracellular water is distributed as 3 L of plasma water, 8 L of interstitial and lymph water, 5 L of water trapped in dense connective tissue and bone, and 1 L of transcellular water.
Fluid homeostasis is a dynamic process. This process may be viewed as the net result of four subprocesses: fluid intake, fluid absorption, fluid distribution, and fluid excretion. In some individuals who have pathophysiologic conditions, loss of fluid through abnormal routes also occurs. The interplay of these subprocesses is fluid homeostasis (Figure 24-3).
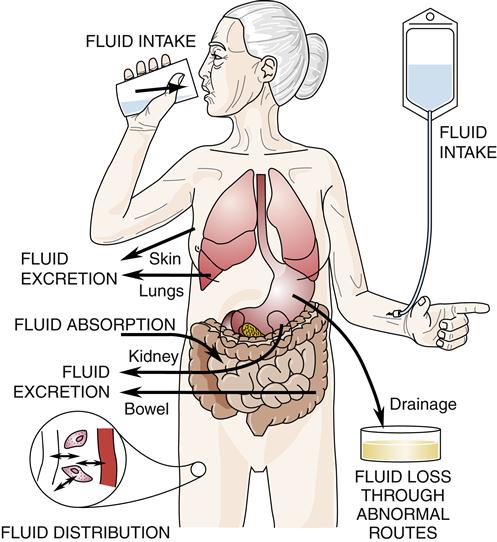
Fluid homeostasis is the interplay of fluid intake and absorption, fluid distribution, fluid excretion, and fluid loss through abnormal routes.
Fluid Intake and Absorption
Fluid intake is entry of fluid into the body by any route. People normally ingest fluids orally, both by drinking and by eating (water contained in food). They also synthesize a small amount of water through cellular metabolism of the foods they eat. Fluid intake by drinking is influenced by habit, social factors, and thirst. Physiologic triggers of thirst include increased osmolality (concentratedness) of extracellular fluid (osmoreceptor-mediated thirst); decreased circulating blood volume (baroreceptor-mediated and angiotensin II–mediated thirst); and dryness of the mucous membranes of the mouth and possibly other visceral signals.2 In older adults, cerebral osmoreceptor-mediated thirst diminishes; thus, older adults who do not have a habit of drinking fluids throughout the day may not have sufficient fluid intake to meet their needs.3
Additional routes of fluid intake that may occur in patients who have various pathophysiologic conditions include intravenous intake; intake tubes into the gastrointestinal tract, other body cavities, subcutaneous tissue, or bone marrow; rectal intake (such as tap water enema); and, occasionally, intake through the lungs (such as near-drowning). Health care professionals often control fluid intake by many of these routes.
Unless fluid intake occurs intravenously, the fluid must be absorbed before it reaches the vascular compartment. Fluid absorption from the gastrointestinal tract partially depends on osmotic forces generated by absorption of electrolytes and other particles.
Fluid Distribution
Much of the fluid that reaches the vascular compartment then distributes into other fluid compartments. Fluid distribution between the vascular and interstitial compartments is the net result of filtration across permeable capillaries. At the capillary level, two forces tend to move fluid from the capillaries into the interstitial compartment: capillary hydrostatic pressure (the outward push of vascular fluid against the capillary walls) and interstitial fluid osmotic pressure (the inward-pulling force of particles in the interstitial fluid). Concurrently, two forces tend to move fluid from the interstitial compartment into the capillaries: capillary osmotic pressure (the inward-pulling force of particles in vascular fluid) and interstitial fluid hydrostatic pressure (the outward push of interstitial fluid against the outside of the capillary walls).1
The distribution of fluid between the vascular and interstitial compartments is analogous to two groups of people pushing on opposite sides of a swinging door—the strongest “push” will determine in which direction the door will swing. Thus, at any one point along a capillary, the direction and amount of fluid flow between the vascular and interstitial compartments are determined by the net result of opposing forces. These forces are illustrated in Figure 24-1.
In contrast, the distribution of fluid between the interstitial and intracellular compartments occurs by osmosis, rather than by filtration. Cell membranes are semipermeable membranes. This means that they are permeable to water but not to electrolytes, many of which require specialized transport mechanisms to cross a cell membrane. Thus, water can move freely through a cell membrane, but electrolytes and other particles cannot. When there is a difference in particle concentration (osmolality) inside and outside cells because the particles cannot move freely, the water crosses the membrane rapidly to equalize the osmolality. Osmosis occurs until the intracellular osmotic pressure and the interstitial fluid osmotic pressure become equal, as in Figure 24-1.
The direction of movement of water by osmosis is determined by the particle concentrations on the two sides of the semipermeable cell membrane. If, on the one hand, the particle concentration (osmolality) of the interstitial fluid becomes higher than the particle concentration inside cells, water will move by osmosis from the cells to the interstitial fluid to equalize the osmolality in the two compartments. If, on the other hand, the osmolality of the interstitial fluid becomes lower than the osmolality of the intracellular fluid, then water will move from the interstitial compartment to the intracellular compartment to equalize the osmolality. In this way, changes in the osmolality of the interstitial and intracellular compartments control the distribution of water between them.1
Distribution of fluid between the intracellular and transcellular compartments is controlled by processes within the epithelial cells that secrete these fluids.
Fluid Excretion
The fourth component of fluid homeostasis is fluid excretion. Fluid excretion normally occurs through the urinary tract, bowels, lungs, and skin. Fluid is excreted through the skin as visible sweat (which may or may not occur) and as insensible perspiration (which always occurs). Another obligatory route of excretion of water is through the lungs as a person exhales. Fecal excretion of fluid occurs with normal bowel function and increases dramatically in a person who has diarrhea. In most circumstances, the largest volume of fluid is excreted in the urine.
The amount of fluid excreted in the urine is controlled primarily by the hormones antidiuretic hormone (ADH), aldosterone, and natriuretic peptides (e.g., A-type natriuretic peptide [ANP]), and to a lesser degree by minor hormones such as renal prostaglandins and by the renal sympathetic nerves. ADH is synthesized by cells in the supraoptic and paraventricular nuclei of the hypothalamus. The axons of these cells extend down the median eminence of the pituitary stalk. The release of ADH thus occurs from the posterior pituitary gland. Factors that increase release of ADH into the blood include increased osmolality (concentratedness) of the extracellular fluid, decreased circulating fluid volume, pain, nausea, and physiologic and psychological stressors. The hormone circulates to the distal tubules and collecting ducts in the kidneys where, consistent with its name, ADH causes reabsorption of water that dilutes the blood and other body fluids. Reabsorption of water decreases the urine volume and makes the urine concentrated, thus decreasing fluid excretion. Factors that decrease ADH release (such as decreased osmolality of the extracellular fluid and ethanol intake) allow a large, dilute urine volume.
Aldosterone is another hormone that influences urine volume. Aldosterone is synthesized and secreted by cells in the adrenal cortex. The major stimuli for its release are angiotensin II (from the renin-angiotensin system, which is activated by decreased circulating blood volume) and an increased concentration of potassium ions in the plasma.4 Aldosterone causes the renal tubules to reabsorb sodium and water (saline), which expands the extracellular fluid volume. This renal action decreases fluid excretion, although by a different mechanism than ADH. When more aldosterone is secreted, the urine volume is smaller; decreased secretion of aldosterone causes a larger urine volume.
A comparison of ADH and aldosterone is useful to remember their actions. ADH is the tap water hormone. It causes the kidneys to reabsorb water. Renal reabsorption of water caused by ADH makes a smaller volume of more concentrated urine and dilutes body fluids. Aldosterone is the salt water hormone. It causes the kidneys to reabsorb sodium and water. Renal reabsorption of sodium and water caused by aldosterone makes a smaller volume of urine and expands extracellular fluid volume.
ANP normally is stored in granules in the cardiac atrial cells and released when the atria are stretched. B-type natriuretic peptide (BNP) is synthesized and released from ventricular cells when ventricular diastolic pressure increases abnormally, as in heart failure. ANP and BNP cause natriuresis (sodium excretion in the urine), which is accompanied by water excretion. Thus, these natriuretic peptides (NPs) promote fluid excretion in the urine. When the vascular volume increases, the heart is stretched, and more NPs are released to cause renal excretion of the excess fluid.5 When the vascular volume is decreased, the heart is less stretched; therefore, fewer NPs are released and the kidneys excrete less fluid. NPs oppose the action of aldosterone, but they are not as strong as aldosterone.
The urine volume that an individual produces also is highly dependent on having adequate blood pressure to perfuse the kidneys and on the glomerular filtration rate. Thus, renal excretion of fluid is the end result of several factors, including hormones that respond to different stimuli and have different actions on the renal tubules.
Fluid Loss Through Abnormal Routes
People who have pathophysiologic conditions often experience loss of fluid through abnormal routes. Examples of these routes are emesis; tubes in the gastrointestinal tract or other body cavities; hemorrhage; drainage from fistulas, wounds, or open areas of skin; and paracentesis. Fluid lost through abnormal routes may be a significant factor in disturbing fluid homeostasis.
If the body’s physiologic mechanisms are functioning well, the processes of fluid homeostasis maintain normal body fluid status. If fluid intake is large, fluid excretion increases by the mechanisms described previously that increase urine volume (large volume of dilute urine). If fluid intake is diminished or if fluid is lost through abnormal routes, fluid excretion decreases (small volume of concentrated urine), and thirst may cause an increase in fluid intake.
If pathophysiologic processes interfere with normal fluid homeostasis or if the normal processes become overwhelmed, then fluid imbalances result. For example, a person who has a pathophysiologic process that prevents the kidneys from excreting much fluid may accumulate too much fluid unless the fluid intake is reduced. The opposite problem will occur in a person whose fluid intake is too small to replace a large amount of fluid excreted or lost through abnormal routes.
Fluid Imbalances
If fluid homeostasis is disturbed by pathophysiologic processes or other factors (such as medications), fluid imbalances may occur. Fluid imbalances fall into two major categories: imbalances of extracellular fluid volume (saline imbalances) and imbalances of body fluid concentration (water imbalances).
Extracellular Fluid Volume
In some circumstances, individuals have too much or too little extracellular fluid. These disorders are called extracellular fluid volume (ECV) imbalances because they involve a change in the amount (volume) of the extracellular fluid. These disorders also are termed saline imbalances because they are disorders of isotonic salt water. (Isotonic saline is salt water in the same concentration as the normal plasma concentration.) In an ECV imbalance, the concentration of the extracellular fluid may be normal; there is simply too much or too little of it. Some individuals have an ECV imbalance and an imbalance of body fluid concentration at the same time. In this case, both the volume and serum sodium concentration of the extracellular fluid are abnormal. This section discusses only the isotonic volume imbalances; the concentration imbalances are discussed separately because they may occur separately.
Volume Deficit
ECV deficit is caused by removal of a sodium-containing fluid from the body. It is a decrease in saline (isotonic salt water) in the same concentration as the normal extracellular fluid, which is why the condition sometimes is termed saline deficit. In an uncomplicated ECV deficit, the serum sodium concentration is normal. The concentration of the extracellular fluid is normal; the amount of the extracellular fluid is abnormally decreased (Figure 24-4).
Etiology
Specific causes of ECV deficit are listed in Box 24-1. All causes of ECV deficit involve removal of a sodium-containing fluid from the extracellular compartment. The sodium-containing fluid usually is removed from the body; however, it may be sequestered in a “third space” in the body that is outside the extracellular compartment. For example, ascites (fluid in the peritoneal cavity) that develops rapidly may deplete the ECV. Another example is fluid that accumulates rapidly in the bowel during an acute intestinal obstruction. Although the fluid in these examples remains in the body, it no longer is part of the extracellular fluid, and signs and symptoms of ECV deficit occur.
Clinical manifestations
Signs and symptoms of ECV deficit are the result of decreased fluid volume in the vascular and interstitial areas. These clinical manifestations include sudden weight loss, postural blood pressure decrease with concurrent increased heart rate, flat neck veins (or veins collapsing with inspiration) when a patient is supine, prolonged small-vein filling time, prolonged capillary refill time, lightheadedness, dizziness, syncope, and oliguria. If the kidneys are responding normally, the small volume of urine will be concentrated (and thus quite yellow). An ECV deficit that develops slowly also may be manifested by decreased skin turgor (skin tenting when it is pinched up over the sternum), dryness of oral mucous membranes between cheek and gum, hard stools, soft sunken eyeballs, longitudinal furrows in the tongue, and absence of tears and sweat. An infant who develops ECV deficit has a sunken fontanel; neck veins are not reliably assessed in infants.
Sudden weight loss is a sensitive measure of ECV deficit. One liter of saline weighs 1 kg; therefore, a person who loses 1 kg in 24 hours has excreted 1 L of fluid or lost it through an abnormal route. It is not possible to lose a kilogram of fat overnight; a sudden weight loss of this magnitude results only from fluid loss, if the body weight is measured accurately. An ECV deficit may occur without a weight loss if fluid is sequestered in a third space somewhere in the body, as with ascites or intestinal obstruction.
A postural blood pressure decrease with concurrent increased heart rate that is measured when a previously supine person stands, or sits with legs dependent, is a good indicator of fluid volume depletion in the vascular compartment. Severe ECV deficit may lead to hypovolemic shock, which can be fatal if not treated effectively with fluid replacement.
Volume Excess
ECV excess is essentially the opposite of an ECV deficit. It is the condition in which the amount of extracellular fluid is abnormally increased. Both the vascular and the interstitial areas have too much isotonic fluid (Figure 24-5). In an uncomplicated ECV excess, the concentration of the extracellular fluid is normal, but an excessive amount of that fluid is present.
Etiology
ECV excess is caused by addition or retention of saline (salt water in the same concentration as normal plasma). For this reason, it sometimes is termed saline excess. As mentioned previously, the hormone aldosterone causes the kidneys to retain saline. ECV excess, therefore, may be caused by conditions that involve excessive aldosterone secretion. For example, increased aldosterone secretion is a compensatory mechanism that commonly accompanies chronic heart failure and eventually leads to ECV excess.6 Additional causes of ECV excess are presented in Box 24-2.
Clinical manifestations
Signs and symptoms of ECV excess are sudden weight gain, edema, and manifestations of circulatory overload: bounding pulse, neck vein distention in a person in the upright position, crackles in the dependent portions of the lungs, dyspnea, orthopnea, and even the frothy sputum of pulmonary edema. An infant who develops ECV excess has a bulging fontanel; assessment of neck veins is not effective in infants.
Sudden weight gain is a sensitive measure of ECV excess. It is impossible to gain a kilogram of fat overnight; such a sudden weight gain is an accumulation of saline. People who eat salty food in a restaurant weigh more the next day because the water they drank combined with the salt in the food to make isotonic saline. The isotonic saline expands the extracellular fluid, causing a mild saline excess until it is excreted by the kidneys. This is the reason that low-sodium diets are prescribed for people who have pathophysiologic processes that cause saline excess (e.g., compensated heart failure).
Body Fluid Concentration
In contrast to the ECV disorders just discussed, imbalances of body fluid concentration are disorders of the concentration rather than of the amount of extracellular fluid. Body fluid concentration disorders also are called water imbalances. The serum sodium concentration reflects the osmolality (concentratedness) of the blood. Imbalances of body fluid concentration are recognized by abnormal serum sodium concentration. The normal serum sodium concentration is 135 to 145 mEq/L (may vary slightly with different laboratories). Many individuals develop imbalances of both ECV and serum sodium concentration at the same time. Isolated imbalances of serum sodium concentration may also occur. This section discusses the concentration imbalances separately.
Hyponatremia
Natrium is the Latin word for sodium. A serum sodium concentration below the lower limit of normal indicates hyponatremia. When hyponatremia is present, the extracellular fluid contains relatively too much water for the amount of sodium ions present; it is more dilute than normal.
Etiology
Hyponatremia is caused by factors that produce a relative excess of water in proportion to salt in the extracellular fluid. Because the serum sodium concentration reflects the osmolality of the blood, the reduced serum sodium concentration of hyponatremia indicates that the extracellular fluid has a reduced osmolality; it is too dilute. Hyponatremia also is called hypotonic syndrome, hypoosmolality, and water intoxication. All of these terms reflect the abnormally dilute concentration of the extracellular fluid that results when the normal proportion of salt to water in the extracellular fluid is disrupted by gaining more water than salt or losing relatively more salt than water.
A gain of relatively more water than salt will cause hyponatremia. As mentioned previously, the hormone ADH causes the kidneys to retain water (not sodium and water) in the body. This hormone is part of the system that normally regulates the osmolality of extracellular fluid. However, circumstances that cause prolonged or excessive release of ADH cause the kidneys to retain too much water, which effectively dilutes the blood; hyponatremia is the result. ADH secretion is excessive in the syndrome of inappropriate secretion of ADH (SIADH). ADH also may be produced ectopically. For example, small cell (oat cell) carcinoma is a type of lung tumor that frequently synthesizes and releases ADH. This ectopic production of ADH from a tumor is not subject to the feedback inhibition of normal ADH release, so inappropriate amounts of ADH are released. With continually high levels of ADH being produced by the tumor, the kidneys retain excessive amounts of water—a gain of water relative to salt. Pain, nausea, and other physical and psychological stressors also increase ADH release, which can be significant in hospitalized patients, especially in the postoperative period. Recent research links the proinflammatory cytokine interleukin-6 (IL-6) to increased ADH release during inflammatory conditions.7 Although it is difficult to drink enough water to cause hyponatremia, water intake that exceeds renal excretory capacity is seen in some situations. For example, the hyponatremia of beer potomania arises when people habitually drink 14 or more cans of beer daily with very little food intake.8 Factors that cause hyponatremia by gain of water relative to salt are presented in Box 24-3.
Hyponatremia also may be caused by a loss of relatively more salt than water. If salt is removed from the body while water remains, then the extracellular fluid once again will become too dilute; hyponatremia results. Factors that cause hyponatremia by loss of salt relative to water also are presented in Box 24-3. Although Box 24-3 separates causes of hyponatremia into two categories, some types of hyponatremia are due to simultaneous gain of water and loss of salt. For example, hyponatremia in marathon runners occurs from loss of salt through heavy sweating and gain of water from excessive water intake plus inappropriate renal water reabsorption caused by increased ADH secretion.9
Clinical manifestations
Clinical manifestations of hyponatremia are nonspecific manifestations of central nervous system dysfunction.10 They vary from malaise, anorexia, nausea, vomiting, and headache to confusion, lethargy, seizures, and coma. Profound hyponatremia causes fatal cerebral herniation. The signs and symptoms are caused by swelling of neurons and glial cells as a result of the decreased osmolality of extracellular fluid. When the extracellular fluid becomes too dilute, the intracellular fluid initially is more concentrated. Therefore, water moves into cells by osmosis (Figure 24-6). The severity of the signs and symptoms depends on how rapidly hyponatremia develops as well as on the absolute value of the serum sodium concentration. A rapid decrease in osmolality produces more severe manifestations than a slow decline, other factors being equal.
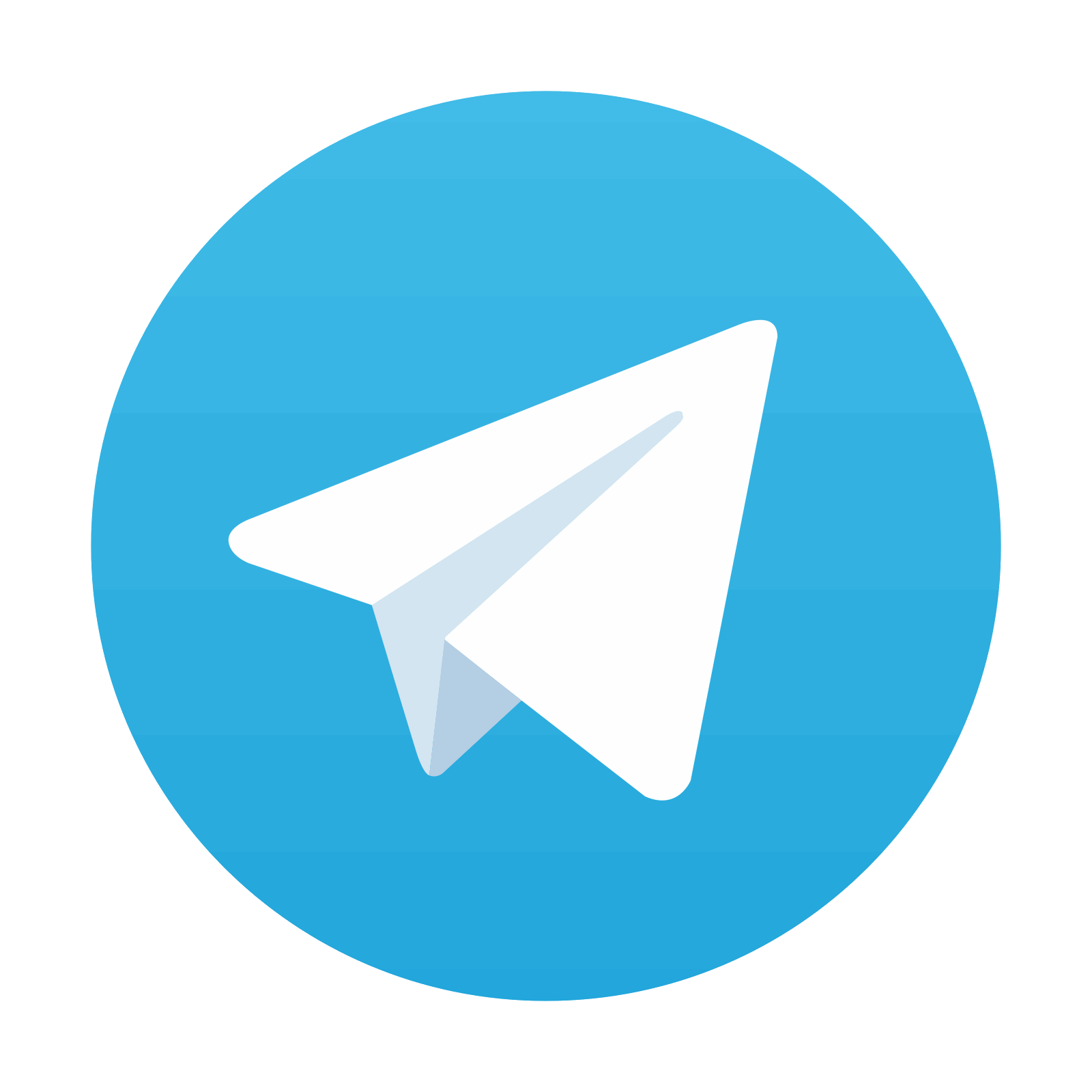
Stay updated, free articles. Join our Telegram channel

Full access? Get Clinical Tree
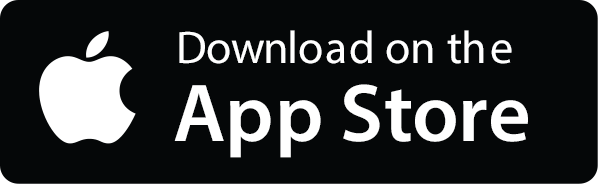
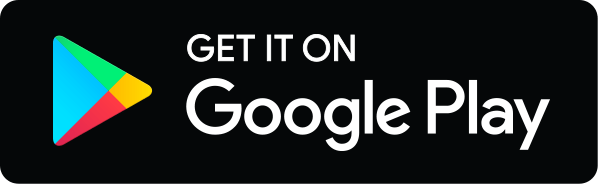