Figure 24–1 Pathophysiology of Parkinson’s disease. The striatum receives input from the entire cerebral cortex and the substantia nigra and sends projections to the thalamus via direct and indirect pathways through the globus pallidus, substantia nigra, and subthalamus. Striatal dopamine D1 receptors excite the direct pathway, whereas D2 receptors inhibit the indirect pathway. In Parkinson’s disease, the degeneration of dopaminergic neurons leads to decreased activity in the direct pathway and increased activity in the indirect pathway. As a result of these changes, thalamic input to the motor area of the cortex is reduced, and the patient exhibits rigidity and bradykinesia. GABA = γ-aminobutyric acid; Glu = glutamate; VA/VL = ventral anterior and ventral lateral nuclei; (+) = excitatory; (–) = inhibitory.
Excitatory cholinergic neurons also participate in the interconnections between structures in the basal ganglia. In Parkinson’s disease, the degeneration of inhibitory dopaminergic neurons leads to a relative excess of cholinergic activity in these pathways. For this reason, patients with Parkinson’s disease can be treated effectively with drugs that inhibit cholinergic activity or with drugs that increase dopamine levels in the basal ganglia.
DRUGS THAT INCREASE DOPAMINE LEVELS
Dopamine levels in the basal ganglia can be increased by various drugs in different ways. Levodopa increases dopamine levels by increasing dopamine synthesis; selegiline by inhibiting dopamine breakdown; and amantadine by increasing dopamine release from neurons. Carbidopa and tolcapone increase the amount of levodopa that enters the brain and thereby enhance dopamine synthesis. The sites of action of these drugs are illustrated in Figure 24–2.
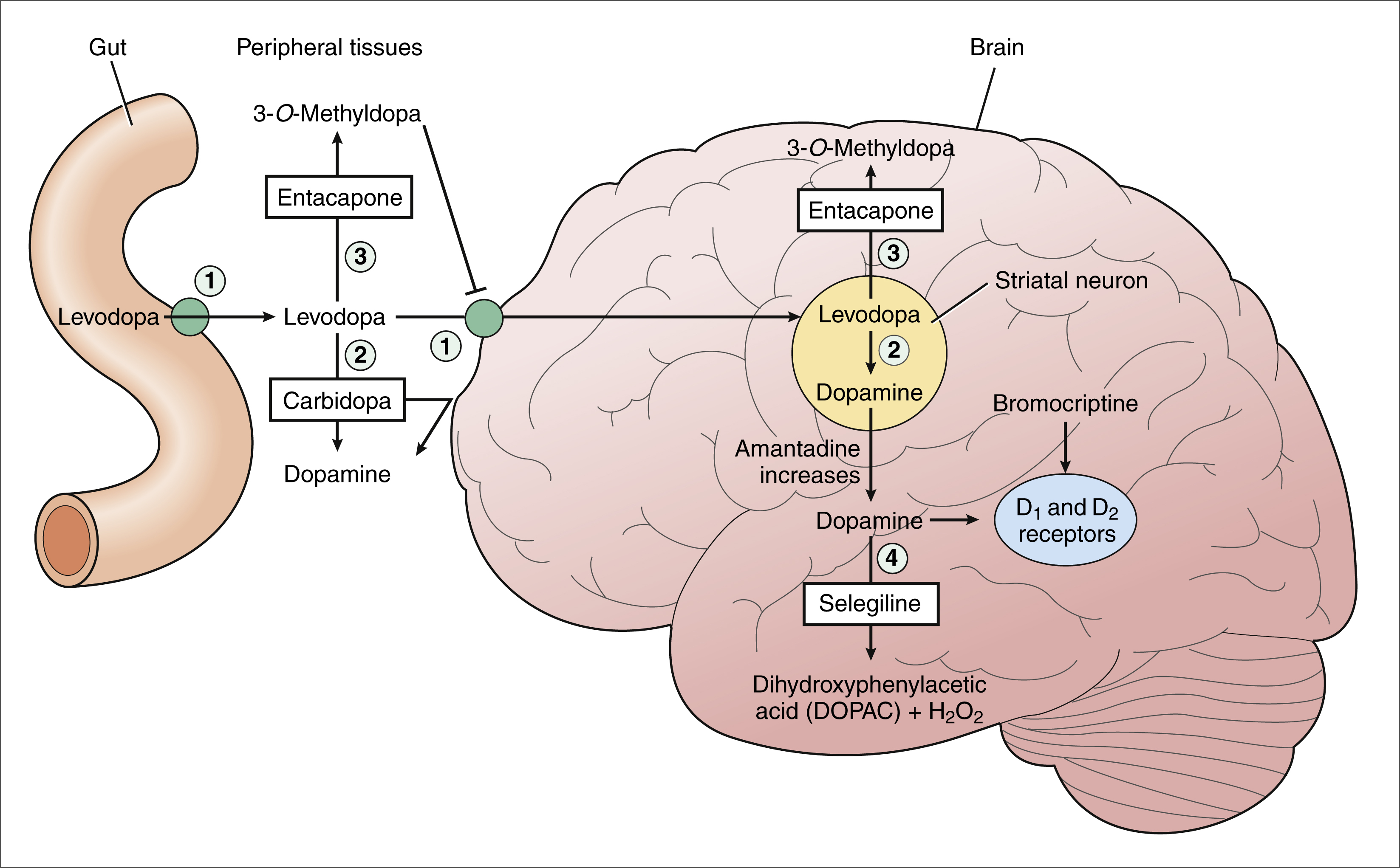
Figure 24–2 Mechanisms of dopaminergic drugs used in the treatment of Parkinson’s disease. Levodopa is transported across the gut wall and the blood-brain barrier and is converted to dopamine in striatal neurons. Carbidopa inhibits the peripheral decarboxylation of levodopa and thereby increases the amount of levodopa that enters the brain. Carbidopa does not cross the blood-brain barrier and does not inhibit dopamine synthesis in the brain. Tolcapone and entacapone inhibit the methylation of levodopa in both peripheral tissues and the brain. Tolcapone and entacapone increase levodopa bioavailability and increase brain uptake of levodopa. Selegiline inhibits the degradation of dopamine to dihydroxyphenylacetic acid (DOPAC) and hydrogen peroxide (H2O2). By this action, selegiline increases dopamine levels in the striatum, and it may reduce the formation of free radicals that are derived from H2O2. Amantadine increases the release of dopamine from striatal neurons. Bromocriptine and other dopamine-receptor agonists activate dopamine receptors in the striatum. Numbered structures and enzymes are as follows: 1 = pump that transports large neutral amino acids; 2 = l-aromatic amino acid decarboxylase (LAAD); 3 = catechol-O-methyltransferase (COMT); 4 = monoamine oxidase type B (MAO-B).
Levodopa
Pharmacokinetics. Levodopa, also called l-dopa or dihydroxyphenylalanine, is the biosynthetic precursor of dopamine. Levodopa increases the concentration of dopamine in the brain and is the main treatment used to alleviate motor dysfunction in patients with Parkinson’s disease. Dopamine itself is not effective in the treatment of Parkinson’s disease when administered systemically, because it does not cross the blood-brain barrier to a significant extent.
Levodopa is absorbed from the proximal duodenum by the same process that absorbs large neutral amino acids (see Fig. 24–2). Dietary amino acids compete with levodopa for transport into the circulation, and amino acids can also reduce the transport of levodopa into the brain. For these reasons, the ingestion of high-protein foods can decrease the effectiveness of levodopa, and a protein-restricted diet may improve the response to levodopa in some patients.
Levodopa is metabolized by two pathways in peripheral tissues. It is converted to dopamine by l-aromatic amino acid decarboxylase (LAAD), and it is metabolized to 3-O-methyldopa (3OMD) by catechol-O-methyltransferase (COMT). A drug that inhibits LAAD (e.g., carbidopa) or inhibits COMT (e.g., tolcapone) is used in combination with levodopa to increase the amount of levodopa that enters brain tissue. LAAD requires vitamin B6 (pyridoxine) as a cofactor. For this reason, vitamin B6 supplements may enhance the peripheral decarboxylation of levodopa and should not be co-administered with levodopa.
Levodopa exhibits a large first-pass effect, and about 95% of an administered dose is metabolized in the gut wall and liver before it reaches the systemic circulation. Additional amounts of levodopa are converted to dopamine and 3OMD before the drug enters the CNS. Therefore, only about 1% of the administered dose of levodopa reaches brain tissue.
MECHANISMS AND PHARMACOLOGIC EFFECTS
In the brain, levodopa is taken up by dopaminergic neurons in the striatum and is converted to dopamine by LAAD. Levodopa thereby increases the amount of dopamine released by these neurons in patients with Parkinson’s disease, and it serves as a form of replacement therapy. Levodopa can counteract all of the signs of parkinsonism, although the degree and duration of its effectiveness usually are not optimal. As the disease progresses and more dopaminergic neurons are lost, the conversion of levodopa to dopamine declines.
About 60% to 70% of nigrostriatal dopaminergic neurons are lost before the clinical symptoms of Parkinson’s disease are first observed, and the degeneration of these neurons continues throughout the course of the disease. Over time, patients begin to experience two types of fluctuation in the effectiveness of levodopa, both of which are probably related to a reduced concentration of dopamine in the striatum. The first type, a “wearing off” effect, occurs toward the end of a dosage interval. The second type, the “on-off” phenomenon, is characterized by severe motor fluctuations that occur randomly.
ADVERSE EFFECTS
When levodopa is used alone, nausea and vomiting occur in about 80% of patients, orthostatic hypotension is reported in 25%, and cardiac arrhythmias occur in 10%. These effects, which are caused by the action of dopamine on β-adrenoceptors in the case of cardiac arrhythmias, are substantially reduced when carbidopa (see below) is administered with levodopa to block the peripheral formation of dopamine.
About 30% of patients who are treated with levodopa on a long-term basis eventually develop involuntary movements, or dyskinesias, as a result of excessive dopamine concentrations in the striatum. Dyskinesias most often occur when levodopa concentrations are highest, in which case they are called peak-dose dyskinesias. The dyskinesias often involve the oral and facial musculature, and patients can appear as if they are chewing on large pieces of food while protruding their lips. Other common dyskinesias involve writhing and flinging movements of the arms and legs. Less commonly, levodopa causes psychotic effects, including hallucinations and distorted thinking, which are probably caused by excessive dopamine concentrations in mesolimbic and mesocortical pathways. Although dyskinesias and psychotic effects could be managed by reducing the levodopa dosage, the therapeutic efficacy of the drug would be reduced as well.
Some patients treated with levodopa complain of sedative effects, agitation, delirium, vivid dreams, or nightmares. Others, however, report a pleasant euphoria after taking the drug.
INTERACTIONS
Levodopa has important interactions with a number of medications. Drugs that delay gastric emptying, such as anticholinergic drugs, can slow levodopa absorption and reduce its peak serum concentration. Drugs that promote gastric emptying (e.g., antacids) can increase levodopa bioavailability. Nonselective monoamine oxidase inhibitors (MAOIs), for example the antidepressant phenelzine, inhibit the breakdown of dopamine and sometimes cause a hypertensive crisis in patients receiving levodopa. Antipsychotic drugs block dopamine receptors and can reduce the effectiveness of levodopa and exacerbate motor dysfunction. Because clozapine is much less likely to do this than other antipsychotic drugs, it is often used to manage psychotic reactions in patients receiving levodopa (see Chapter 22).
INDICATIONS
Levodopa is effective in the management of patients with idiopathic Parkinson’s disease and in the treatment of patients with postencephalitic parkinsonism, a disorder portrayed in the movie Awakenings. It is also used to treat parkinsonian symptoms caused by carbon monoxide poisoning, manganese intoxication, or cerebral arteriosclerosis.
Carbidopa
Carbidopa, a structural analogue of levodopa, inhibits LAAD thereby reducing the conversion of levodopa to dopamine in peripheral tissues and so increasing the amount of levodopa that enters the brain (see Fig. 24–2). Carbidopa is highly ionized at physiological pH, and it does not cross the blood-brain barrier. For this reason, it does not inhibit the formation of dopamine in the CNS.
Carbidopa substantially reduces the gastrointestinal and cardiovascular side effects of levodopa and enables about a 75% reduction in the dosage of levodopa. A levodopa-carbidopa combination is available in immediate-release and sustained-release formulations that contain different ratios of the two drugs. The sustained-release formulations are designed to reduce the “wearing off” effect described above.
Amantadine
Amantadine is an antiviral drug that is used in the prevention and treatment of influenza but also has a beneficial effect on Parkinson’s disease. Amantadine appears to work by increasing the release of dopamine from nigrostriatal neurons, but it may also inhibit the reuptake of dopamine by these neurons. Amantadine is generally better tolerated than levodopa or dopamine agonists, but it is also less effective.
Amantadine is used to treat early or mild cases of Parkinson’s disease and as an adjunct to levodopa. Its adverse effects include sedation, restlessness, vivid dreams, nausea, dry mouth, and hypotension. It can also cause livedo reticularis, which is a reddish-blue mottling of the skin with edema. CNS side effects are more likely to occur in the elderly because of their reduced capacity to excrete the drug by the kidneys.
Selegiline
PHARMACOKINETICS
Selegiline, also known as deprenyl, is a modified phenylethylamine compound. The drug is well absorbed from the gut and is partly metabolized to amphetamine.
MECHANISMS AND PHARMACOLOGIC EFFECTS
Selegiline inhibits monoamine oxidase type B (MAO-B) and thereby prevents the oxidation of dopamine to dihydroxyphenylacetic acid and hydrogen peroxide, as shown in Figure 24–2. By this action, selegiline increases dopamine levels in the basal ganglia and decreases the formation of hydrogen peroxide. In the presence of iron, hydrogen peroxide is converted to hydroxyl and hydroxide free radicals that may participate in the degeneration of nigrostriatal neurons in patients with Parkinson’s disease.
There is evidence that selegiline inhibits the progression of Parkinson’s disease either by inhibiting the formation of free radicals or by inhibiting the formation of an active metabolite of an environmental toxin. However, the ability of selegiline to inhibit disease progression is controversial. It was initially suggested by studies showing that selegiline could prevent a form of parkinsonism that is induced by 1-methyl-4-phenyl-1,2,3,6-tetrahydropyridine (MPTP). MPTP is a toxic byproduct of the synthesis of “designer” street drugs; in the 1980s, people who took what they thought was a meperidine analogue ingested drugs contaminated with MPTP and developed classic signs of parkinsonism. MPTP must be converted to 1-methyl-4-phenylpyridium by MAO-B before it can damage dopaminergic neurons, and studies demonstrated that selegiline blocked this reaction. The results of these studies have led some investigators to postulate that idiopathic Parkinson’s disease is caused by an environmental toxin whose action resembles that of MPTP. Although unfortunate for the drug abusers who were exposed to MPTP, it did lead to an important animal model for parkinsonism and the development of new agents.
ADVERSE EFFECTS AND INTERACTIONS
Adverse effects are listed in Table 24–1. Unlike the nonselective MAOIs used in treating mood disorders, selegiline does not inhibit monoamine oxidase type A (MAO-A), an enzyme that catalyzes the degradation of catecholamines. For this reason, selegiline is much less likely to cause hypertension when it is administered in combination with sympathomimetic amines or when it is taken with foods that contain tyramine. The drug’s selectivity for MAO-B, however, is lost when it is given in higher doses, so the potential for food interaction still exists. Selegiline can cause adverse effects if it is administered with meperidine or with selective serotonin reuptake inhibitors (SSRIs; e.g., fluoxetine) as noted in Chapter 22.
INDICATIONS
Selegiline can be used as a single drug for the treatment of early or mild Parkinson’s disease, and it is used as an adjunct with levodopa-carbidopa for advanced disease. Selegiline reduces the dosage requirement for levodopa, and it may improve motor function in patients who experience “wearing off” and “on-off” difficulties with levodopa.
The most controversial role of selegiline is its use as a neuroprotective agent. Although some clinical studies have indicated that selegiline slows the progression of Parkinson’s disease, others have indicated that it does not.
Recently a second MAO-B inhibitor, rasagiline, was approved as a monotherapy or adjunct medication in the treatment of parkinsonism. It has the same potential adverse effects as selegiline, due to interactions with tyramine, meperidine, and SSRIs.
Tolcapone
Tolcapone is a drug used to enhance the effectiveness of levodopa in the treatment of Parkinson’s disease. It inhibits COMT, the enzyme that metabolizes levodopa to 3OMD in the gut and liver. By this action, tolcapone produces a twofold increase in the oral bioavailability and half-life of levodopa. Because 3OMD competes with levodopa for transport into brain tissue, it may contribute to the “wearing off” and “on-off” effects that occur during long-term levodopa therapy. By inhibiting 3OMD formation, tolcapone may stabilize dopamine levels in the striatum and contribute to a more sustained improvement in motor function.
In clinical studies, tolcapone was found to increase the efficacy of levodopa while reducing the dosage requirement. Tolcapone was well tolerated, and most of the side effects were similar in frequency to those reported with use of a placebo. Although tolcapone caused diarrhea and nausea in some patients, these side effects decreased over time. A few reports were made of rare, but fatal hepatitis following administration of tolcapone.
Dopamine Receptor Agonists
The dopamine-receptor agonists directly activate dopamine receptors in the striatum. Because they do not require a functional dopaminergic neuron to produce their effects, they are sometimes helpful in advanced cases of Parkinson’s disease, in which few dopaminergic neurons remain. The drugs work primarily by activating D2 receptors. As shown in Figure 24–1, activation of these receptors leads to inhibition of the indirect neuronal pathway from the striatum to the thalamus and increases thalamic stimulation of the motor area of the cortex.
Bromocriptine
Bromocriptine is an ergot alkaloid, in the same chemical class as the hallucinogen LSD. Other ergot alkaloids used to treat migraine headaches are discussed in Chapter 29. Bromocriptine is a D2-receptor agonist and a D1-receptor antagonist. Bromocriptine can serve as a useful adjunct to levodopa in patients who have advanced Parkinson’s disease and experience “wearing off” effects and “on-off” motor fluctuations.
Dopamine-receptor agonists produce adverse effects that are similar to those of levodopa. Nausea, which occurs in as many as 50% of patients when they are first treated with a receptor agonist, primarily results from stimulation of dopamine receptors in the vomiting center located in the medulla. Dose-related CNS effects of the receptor agonists include confusion, dyskinesias, sedation, vivid dreams, and hallucinations. Other adverse effects include orthostatic hypotension, dry mouth, and decreased prolactin levels. Nausea and many other adverse effects subside over time. To enable patients to develop tolerance to the effects, treatment should begin with a low dose, and the dosage should be increased gradually.
Pergolide (Permax), is also an ergot alkaloid and direct-acting dopamine agonist, but was withdrawn from the market in 2007 due to increased risk of damage to heart valves.
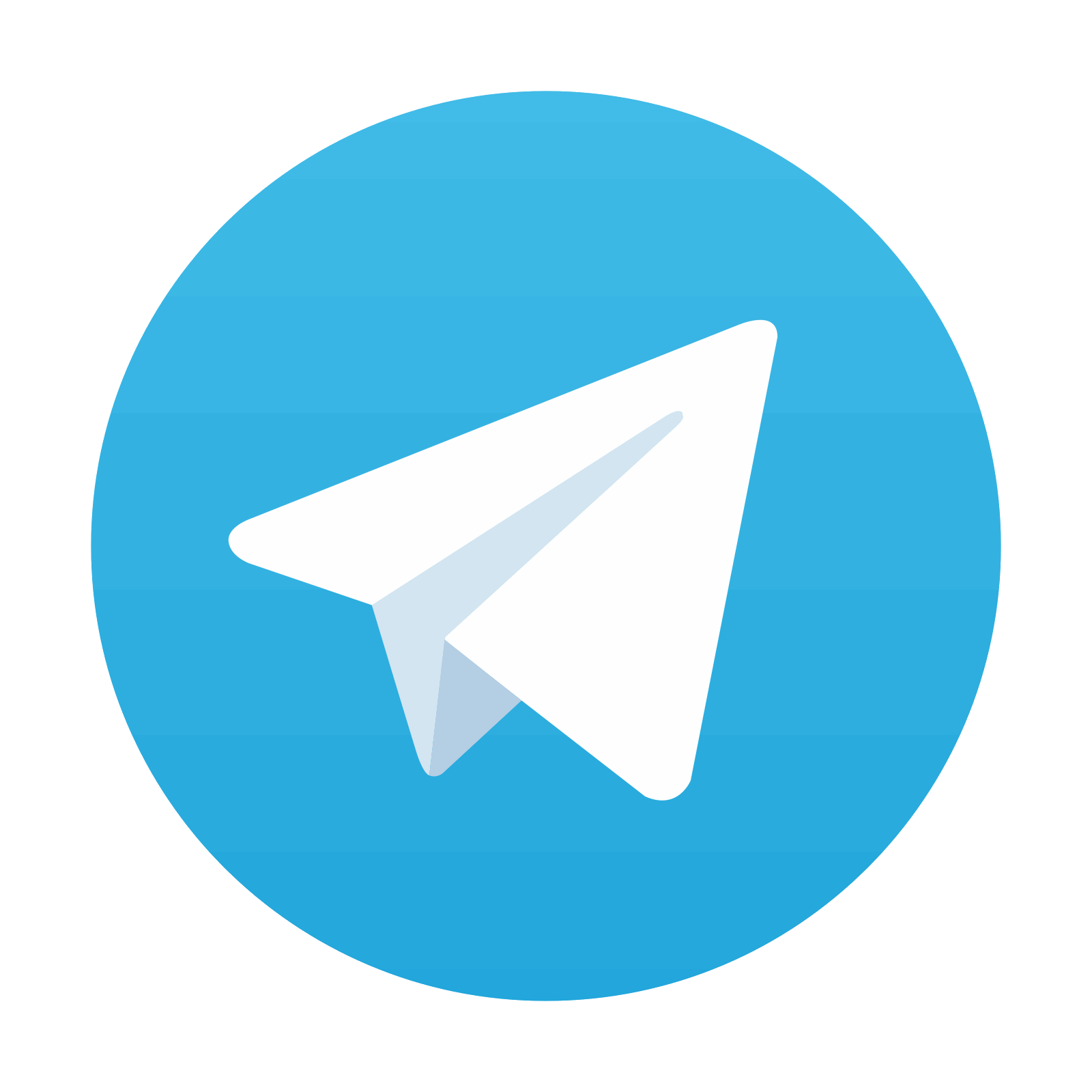
Stay updated, free articles. Join our Telegram channel

Full access? Get Clinical Tree
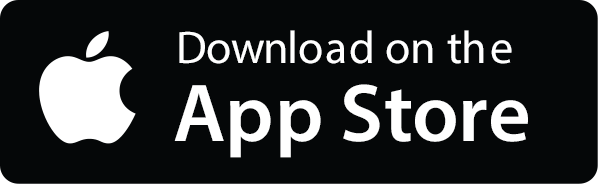
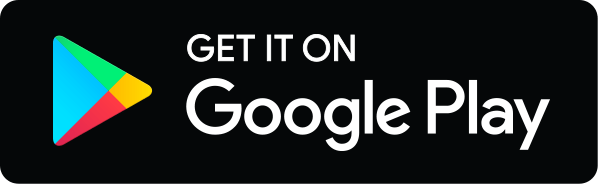