Chapter 4
Drug metabolism
4.1 Overview
In the last chapter, we have considered renal elimination of drugs. While some drugs are eliminated via the kidneys unchanged, most of them undergo metabolic transformation at least in part before being excreted.
4.1.1 Types of reactions in drug metabolism
Notes: These categories of metabolic reactions are very broad; for each category, there is a multitude of enzymes. We have already seen examples of drug metabolism by hydrolysis, which is exploited in the design of resorption esters (see slide 3.4.5). As an example of reductive metabolism, we have seen the activation of sulfamidochrysoidine via azoreduction (see slide 1.3.3). As we will become clear in this chapter, oxidative metabolism and conjugation reactions are even more common.
4.1.2 Functional outcomes of drug metabolism
In most cases, drug metabolism results in inactivation and accelerated elimination of drugs from the body. However, other outcomes of metabolism are possible; we will see examples below.
In the list above, outcomes 2. and 3. both yield active products. The difference between them is that, in 2., both the original drug and the metabolite have pharmacological activity. In contrast, a prodrug has no activity before undergoing metabolic conversion.
4.1.3 A hydrolytic metabolite of cocaine can be detected in wastewater
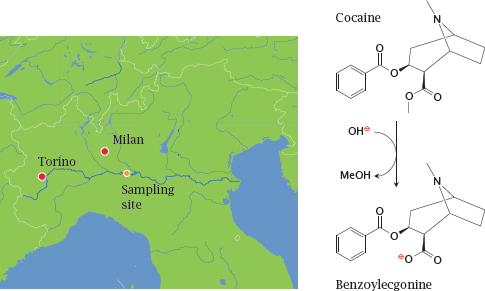
Notes: Cocaine is inactivated by hydrolysis. The metabolite, benzoylecgonine, is excreted with the urine. It is fairly stable and thus can be detected in wastewater.
A study from Italy [15] analyzed water samples from the river Po, which were used to estimate the consumption of cocaine upstream of the sampling site. The samples were taken at a point downstream of Torin and, in part, of Milan, two of Italy’s largest cities. Cocaine consumption in this area was found to be 4-5 times higher than had previously been estimated. Similar findings have since been reported from other European countries.
4.1.4 Metabolism of phenobarbital
Notes: Phenobarbital is a barbituric acid derivative that is sleep-inducing and antiepileptic. The drug molecule itself is quite hydrophobic; this enables it to penetrate the blood brain barrier, and it also slows down renal elimination. Therefore, 75% of the compound is excreted as conjugated metabolites.
Phenobarbital as such is not a good substrate for conjugation reactions. To make it one, a suitable functional group—an aromatic hydroxyl group—is first introduced by a cytochrome P450 enzyme (mostly subtype 3A4; see later). Aromatic or aliphatic hydroxylations are very common as phase I reactions.
In phase II, the hydroxyl group just introduced is conjugated either with glucuronic acid by UDP-glucuronosyltransferases, or with sulfate by sulfotransferases. Both of these metabolites are sufficiently polar and are effectively excreted through the kidneys.
4.1.5 With many drugs, metabolic transformation facilitates excretion
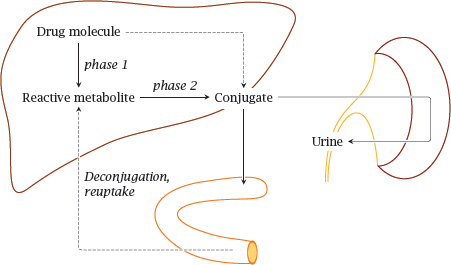
Notes: The metabolism of phenobarbital illustrates a pattern that applies to many other drugs as well. With many drugs, hydroxylation by cytochrome P450 or other phase I reactions precede conjugation with glucuronic acid, glutathione or other functional groups. These conjugations are collectively referred to as phase II reactions. Excretion of conjugated drugs is sometimes referred to as phase III of drug elimination.
The most important organ in drug biotransformation is the liver. The conjugated drugs may be exported into the blood stream and be eliminated in the kidneys, or they may be excreted into the bile and then reach the intestine. In the latter case, they may undergo deconjugation by bacterial enzymes in the large intestine and subsequent reuptake. Reabsorbed drugs will be transported to the liver via the portal vein and may undergo another round of conjugation and biliary excretion; this is called entero-hepatic cycling.
4.1.6 Hydrophobicity does not strongly predict the extent of metabolism
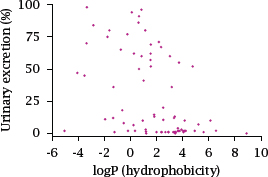
Notes: This scatter plot of a randomly selected sample of 75 drugs (data from [16]) correlates the extent of renal elimination to the estimated logP value, which is the logarithm of the octanol/water partitioning coefficient.
There is a slight trend for hydrophobic drugs to undergo renal elimination to lesser extent; however, only very few drugs are quantitatively eliminated through the kidneys. In most cases, the remainder will undergo metabolic transformation, although some drugs may be excreted into the bile unchanged.
So, the key point of this slide is not in the correlation of the two parameters, but rather in the fact that most drugs undergo metabolic transformation at least in part; contrary to lore, this applies by and large to both hydrophilic and hydrophobic drugs.
4.1.7 Major types of drug-metabolizing enzymes
Phase I
- Cytochrome P450 enzymes
- Diaphorase (NADH:quinone oxidoreductase)
Phase II
- UDP-glucuronosyltransferases
- Sulfotransferases
- Glutathione-S-transferases
- N– and O-acetyltransferases
Notes: Cytochrome P450 enzymes are the most important class of enzymes in phase I metabolism. Other oxidative enzymes in drug metabolism include flavin monooxygenase and monoamine oxidase. Reductive metabolism is carried out by various enzymes; diaphorase, also called quinone reductase, is a major one.
While the number of enzymes involved in the degradation of xenobiotics is large, it is necessarily far smaller than the virtually limitless number of potential substrates. Therefore, many of these enzymes must be able to modify a large number of structurally diverse drugs and xenobiotics. The same also applies to transporters involved in biliary and urinary excretion.
4.2 Cytochrome P450 enzymes
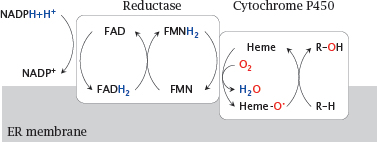
Notes: Cytochrome P450 isoforms are associated with the membrane of the endoplasmic reticulum (mostly) or the mitochondria (some). Membrane association facilitates interfacial processing of lipophilic substrates that often reside in the apolar phase of the membrane.
Cytochrome P450 enzymes cooperate with cytochrome P450 reductase, which acquires two electrons from NADPH and, via two flavin coenzymes,1 passes them on one by one to the heme group in the cytochrome. Thereafter, they are used to reduce one of the oxygen atoms of O2 to water. The second oxygen atom is retained in a highly reactive form, and subsequently carries out the actual attack on the substrate.
4.2.1 Reactions catalyzed by cytochrome P450
Notes: Halfway through the reaction, the active site of cytochrome P450 contains a single oxygen atom in a loosely bound, highly reactive state. This oxygen atom can react with many different functional groups, and in various ways; the particular reaction that occurs with a given substrate depends more on which of its functional groups happens to be the nearest to the oxygen, rather than on which one is the most reactive itself.
The following slides present some examples of drugs that undergo various types of cytochrome P450-mediated oxidative reactions.
4.2.2 The drug oxazepam is also an active metabolite of diazepam
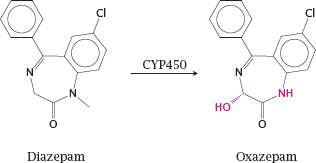
Notes: Diazepam is an agonist of the GABAA receptor in the brain (see slide 6.10.8) that is used as a tranquilizer. Both the hydroxylation and the demethylation of diazepam are performed by cytochrome P450 enzymes. The resulting metabolite, oxazepam, is also used as a drug in its own right. Since it no longer has to undergo phase I metabolism, it is eliminated more rapidly than diazepam, which is advantageous for example when used as a sleeping aid.
4.2.3 Carbamazepine-10,11-epoxide is an active metabolite of carbamazepine
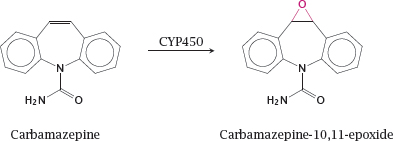
Notes: Carbamazepine blocks sodium channels (slide 6.5.4) that is useful in epilepsy and several other neurological conditions. The epoxide metabolite of carbamazepine significantly contributes to the pharmacological activity, but also to the toxicity, of carbamazepine. Like other epoxides, carbamazepine-10,11-epoxide may wreak havoc by reacting covalently with intracellular nucleophiles.
4.2.4 Fexofenadine is an active metabolite of terfenadine
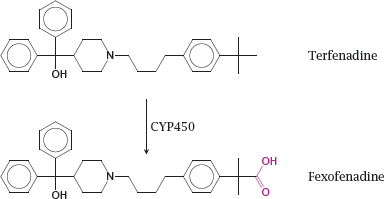
Notes: Fexofenadine is a histamine H1 receptor blocker. It was originally observed as an active metabolite of terfenadine. It has since supplanted the original drug, because it avoids some toxic effects on cardiac excitation that may occur when the parent compound accumulates due to inhibition of cytochrome P450-mediated metabolism.
The metabolism of terfenadine also illustrates that it is possible for one substrate molecule to undergo several rounds of oxidation in one sitting.
4.2.5 Erythromycin bound to the active site of cytochrome P450
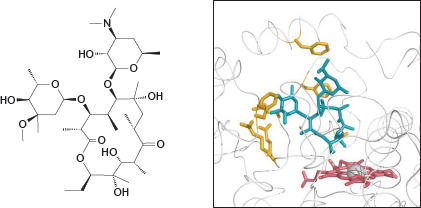
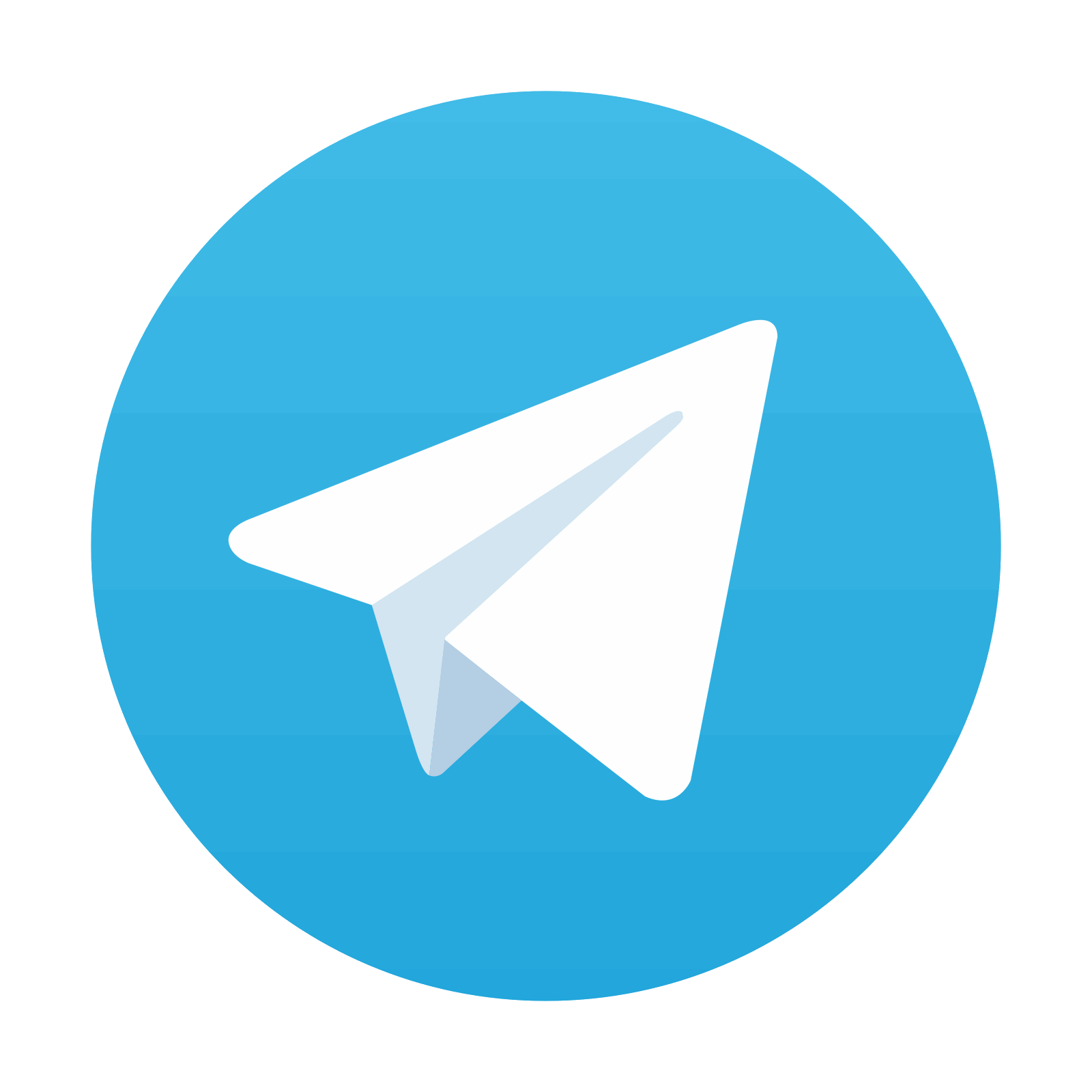