3 Michelle Armstrong, Shonagh Walker, Jenifer Mains and Clive G. Wilson Strathclyde Institute of Pharmacy and Biomedical Sciences University of Strathclyde UK Drug delivery into the nasal cavity has an established role in local delivery of over-the-counter medicines used to treat allergic rhinitis and blocked sinuses. More recently, nasal drug delivery (NDD) has been proven as a useful gateway in hormone replacement therapy using small peptides including salmon calcitonin and DDAVP, which are able to traverse the nasal mucosa and avoid the first pass effect. The potential application of nasal drug delivery in emergency medical care and the prospect of direct nose to brain delivery has stimulated interest from all sections of pharmaceutical sciences. The key advantages are easy accessibility, fast onset of action and the prospect of good to moderate bioavailability of drugs, which are extensively metabolised following oral administration. A place for nasal drug delivery in the emergency treatment of a patient was dramatically illustrated by Dr Rob Curran, who constructed a scenario of a child in a schoolroom, who is in seizure and full tonic-clonic contractions [1]. Imagine that this patient has become cyanotic and establishing an intravenous (IV) line is difficult. Would a highly concentrated drug given as a simple nasal spray save the child’s life? Benzodiazepines for termination of seizures administered on encounter by paramedics result in a reduction in mortality from 15.7% on admission to accident and emergency departments compared to 4.5–7.7% when treated in the field. Intranasal (IN) fentanyl is as effective as morphine in young children presenting with acute fracture. Intranasal naloxone has become standard police issue in New Mexico for encounters with individuals overdosing on heroin. Another issue for the emergency medical team is needle stick injury (NSI), a particular hazard for the less experienced members of the team. There is a documented lack of risk awareness and reporting behaviour surrounding NSI which has existed through generations of medical students and which now impacts on provision of health insurance for professionals [2]. Salzer’s study reveals that 26% of medical students who had reported NSIs were not aware of the patient’s HIV status. The issue of blood-borne disease and NSI is uniform worldwide: Foster and colleagues report that 1% of Jamaican blood donors are seropositive for hepatitis B virus and hepatitis C (HCV) is 0.4%, yielding a risk of infection of 1.8% for HCV in health workers. In 2010, there was no effective anti-HCV immunisation available [3]. The move to ‘needleless’ systems decreases the significant risk of handling blood-contaminated administration sets but progress towards alternatives has been slow. Collopy and colleagues [4], reviewing the progress since Curran’s 2007 article, comment that in part this might be due to the compounding of formulations for nasal administration as ‘off-label’, since there is a scarcity of drugs considered by the US Food and Drug Administration (FDA) for approval for IN administration and, in emergency medicine, many drugs are used within this ‘off-label’ envelope (Table 3.1). Table 3.1 Drugs administered intranasally for unlicensed indications. 1. Prolonged febrile seizures in children 2. Sedation of pre-school dental patients [20–32] There will, of course, be situations in which the nasal turbinates are blocked with congealed blood, or where disease and/or substance misuse may have radically altered the absorbing membrane. Nevertheless, a consideration of the benefits of nasal formulations is more urgent than it was twenty years ago, before the awareness of needle-borne dangers. Another factor to consider is patient acceptability, which can be much higher with nasal formulations than with IV, due in part to patients presenting an aversion to needles [34]. Nasal drug delivery has also shown to be more acceptable than oral formulations in young children [11–14]. Nasal administration of drugs can be carried out by the patient, patient’s carer or primary health care practitioner in an outpatient setting, with minimal training. These formulations could, therefore, be administered rapidly in a pre-hosptial setting, without the presence of emergency health professionals. Drug delivery into the nasal cavity is a useful gateway in the treatment of many topical and systemic ailments; and in addition it holds the prospect of direct nose to brain delivery. In order to safely and effectively dose the nasal cavity, a drug formulation must be effectively administered to, but also retained in, the nose. Whilst older dosage forms (such as nasal bougies) have fallen from favour over the years, nasal sprays and nasal drops remain the staple dosage forms for drug delivery into the nasal cavity. Nasal sprays are the most commonly used delivery system, with a host of different formulations available to treat topical diseases including allergy and nasal congestion (Table 3.2). For the treatment of allergy, corticosteroid preparations are available in the United Kingdom, including Beconase® (beclometasone dipropionate), Flixonase® (fluticasone propionate) and Nasonex® (mometasone furoate). Comparable efficacy of nasal spray formulations has been demonstrated previously [35], with a preference for a particular formulation likely to be related to the odour and the taste associated after administration of the formulation [36]. Table 3.2 Drugs administered intranasally for systemic and local delivery. Nasal administration is also used to deliver a range of drugs systemically. The avoidance of the acidic environment of the stomach, the thin membranes allowing rapid drug absorption and avoidance of first pass metabolism are significant benefits over oral delivery. One of the earliest drugs delivered nasally for systemic effect was nicotine. Comparing a nasal spray at a dose of 1 mg per actuation with an intravenous infusion, the systemic bioavailability of nicotine following nasal administration was estimated to reach levels of 65–70% [37]. Although in a direct comparison of systemic concentrations of nicotine in smokers and patients treated with nasally administered nicotine, reduced nicotine systemic levels were demonstrated with the nasal formulation. Comparable levels were shown when directly comparing transdermal and nasal nicotine administration. A high level of individual variability was present following nasal nicotine administration, thought to be due to improper use of the nasal spray, loss of formulation due to sneezing, loss in the nasopharynx and interpatient variability in absorption [38]. This variability in uptake was not significantly affected by the location of spray deposition [37]. In addition to nicotine, the nasal cavity has also been used in the treatment of migraine through the administration of sumatriptan in a nasal spray formulation (Imigran). In a direct comparison of sumatriptan administered via the oral route and via a nasal spray, no significant difference in bioavailability was seen between the two routes of administration, and the maximum rate of sumatriptan absorption was higher following nasal delivery [39]. With the rapid expansion of the biotech industry over recent years, alongside the delivery of small drug molecules, interest has turned to exploiting the nasal cavity for systemic protein and peptide delivery. Desmospray® (Desmopressin acetate) has been licensed for multiple indications, including the treatment of diabetes insipidus. In comparison to larger nasal drop delivery of desmopressin, the spray formulation has shown to reside more anteriorly and was also cleared more slowly. As a result of this, the spray formulation was able to achieve higher levels systemically, with the volume of the spray influencing the rate of clearance from the nasal cavity [40]. Salmon calcitonin (Miacalcin®) is a peptide compound administered intranasally to reduce the risk of vertebral fractures in patients with established post-menopausal osteoporosis. Questions have been raised about the ability of larger drug molecules to penetrate through the nasal mucosa unaided, due to their molecular size. A clinical study has demonstrated low serum levels of calcitonin following nasal administration of a 200 U, with CMax reaching 4.8 pg/ml ten minutes after administration. When the penetration enhancer sodium taurodihydrofusidate was administered alongside calcitonin at a concentration of 0.5%, nasal administration of a 205 U dose of calcitonin improved dramatically with CMax levels of 70.7 pg/ml achieved ten minutes following administration [41]. In addition to these compounds, more protein and peptide drugs are achieving approval for administration via the nasal cavity, including nafarelin acetate (Synarel®) and buserelin acetate (Suprecur®, Suprefact®) for the treatment of endometriosis and prostatic carcinoma. A key issue for nasal delivery of peptides is the poor permeability coupled with the susceptibility towards metabolic breakdown. Cross described the generation of leu-enkephalinamide derivatives by addition of a non-native amino acid containing a lipophilic side group to allow chain elongation to produce C8 and C12 acetylated derivatives as L- and D- isomers. These had higher permeability in CaCO2 culture lines. Substituting the D-isomer for the L-isomer reduced the rate of metabolism in plasma with t1/2 of 8.9–120 minutes [42]. Cell-penetrating peptides, which are highly cationic, appear to assist the translocation of co-administered peptides without the involvement of receptors. Khafagy and colleagues describe the properties of L-penetratin, which significantly increased the absorption of GLP-1 through nasal and intestinal mucosa [43], and have advanced the concept to insulin and other molecules, generating an analogue, termed ‘shuffle (R,K fix) 2’ [44]. These molecules have to form reversibly bound complexes at a specified binding ratio. Simpler formulation constructs have also proved useful. Matsuyama and colleagues have examined the influence of filler excipients in a salmon calcitonin dry powder system [45]. From work in rat intranasal formulations it was noted that less wettable powders, including ethyl cellulose, provided the highest bioavailability. This system was applied to laboratory studies of human parathyroid and insulin with increased bioavailabilities – around 30%. Draize tests in rabbits suggested that these formulations had a low irritancy potential. The nose is a complex organ positioned on the face between the eyes. It is the gateway to the respiratory system and extends posteriorly to the nasopharynx leading to the trachea and oesophagus. The nasal vestibule, the most anterior part of the nasal cavity, is adjacent to the atrium and opens to the face through external openings known as nares or nostrils. The primary functions of the nasal cavity are olfaction and breathing but it is also involved in the filtration of airborne particles and the humidifying and heating of inspired air. The human nasal cavity is an irregularly shaped space (Figure 3.1). In its entirety, it consists of dual chambers approximately 5 cm high and 7.5 cm long that are subdivided into two halves by a cartilaginous wall called the median septum. Septal cartilage forms the anterior section of the septum and posteriorly it consists of the vomer and perpendicular plate of the ethmoid bone. The nasal cavity fills the void between the mouth and the base of the skull. It is supported above and laterally by the ethmoid bones, and ethmoid maxillary and inferior conchae bones, respectively. The nasal cavity floor, which forms the roof of the mouth, consists of hard palatine bone, the anterior two-thirds, and soft palate, the posterior third of the nasal cavity. The hard palatine bone is made up of bony palate and is responsible for holding the roots of the teeth. The soft palate, or velum palatinum, is a fibromuscular flap of tissue that extends to the nasopharynx and aids in blocking the pharyngeal isthmus during swallowing to prevent food lodging at the rear of the nose and blocking the airway. Figure 3.1 Representation of the nasal cavity including the vestibule, turbinates and epithelial regions. The most anterior part of the nose, situated just inside the nostril, is the nasal vestibule, which represents a total surface area of approximately 0.6 cm2 [46], although there is a difference in the area of the left and right nasal vestibule, with the left being significantly bigger [47]. The area of the vestibule is also known to decrease with age [47]. The covering of the vestibule consists of an external layer of skin that is lined with vibrissae (nasal hairs) and sebaceous and sweat glands. The vibrissae point inferiorly and externally and are involved in the trapping and filtering of inhaled particles. The nasal vestibule possesses characteristics that allow high resistance against harmful environmental substances but this high resistance makes the absorption of drugs in the region very difficult [48]. Posterior to the vestibular region is a small area called the atrium. The largest part of the nasal cavity is the respiratory region, which is divided into three thin projections known as conchae. The conchae, or turbinates, are thin, bony plates covered by a spongy mucosa and are located just behind the vestibule. There are three turbinates on each side of the vestibule: the inferior, middle and superior turbinate. Each turbinate serves to increase the surface area of the cavities and conditions the inhaled air before it reaches the lungs. Between the turbinates are spaces, known as meatus, which are flues through which flows inspired air. The spaces are extremely tight but swell bodies located in the septum and turbinates can adjust the width. The narrow spacing results in the airstream being near to the moist mucus surface, which lines the air space, at all times. The airflow through the meatus is turbulent and the turbinates cause directional change of the air, which encourages inertial impaction of any particles present. The heating and humidification of the air is facilitated by the rich vasculature through the arteriovenous anastomoses in the turbinates. The anterior serous glands, seromucous glands and goblet cells produce an abundance of fluid that aids humidification of the air. Inspired ambient air of between −20 °C and 55 °C can be brought to within 10 degrees of the body temperature, and saturation can be achieved to within 97–98%. The middle meatus, located between the middle and inferior turbinates, is the location for the majority of the airflow from the nose to the pharynx; however, up to 20% of the air is channelled vertically upwards by the internal ostium towards the olfactory region, where it is then arched down towards to nasopharynx. The primary function of the nose is considered to be olfaction, although the region involved in the sense of smelling is relatively small. The olfactory region is located at the uppermost area of the nasal cavity, above the superior turbinate, and extends down the septum and lateral wall. It is lined mostly with a mucous membrane, but a small area is lined by neuroepithelium. Of the whole central nervous system (CNS), the neuroepithelium is the only part that is exposed to the external environment [49]. Dendritic fibres project from the neuroepithelium into the nasal cavity. The dendrites are covered by a thin layer of moisture, produced by the glands of Bowman, which acts as a solvent and dissolves microscopic particles from odorous substances in the air. These then chemically stimulate the olfactory nerve cells and generate a receptor potential in the cell, which results in a nerve impulse being initiated in the olfactory nerves of the brain [50]. More than half of the epithelial surface of the nasopharyngeal mucosa is covered by stratified squamous epithelium. Islets of transitional epithelium separate alternating patches of squamous and ciliated cells in the lateral walls and roof of the nasopharynx. This alternating pattern is also present in the narrow region between the oropharynx and nasopharynx. A mucosal membrane lines the lower part of the pharynx, which is covered by stratified squamous epithelium. Columnar cells line the posterior two-thirds of the nasal cavity. Apical tight junctions connect neighbouring cells, which can be either ciliated or nonciliated. All columnar cells possess approximately 300 microvilli, which are evenly distributed over the entire surface. Each cell is covered by approximately 300 microvilli. Microvilli are short, motile, hair like, cytoplasmic projections that increase the overall surface area of the nose and encourage exchange processes, including drug delivery, across the epithelial layer. The microvilli, along with cilia, inhibit drying of the surface by promoting the transport of water and other substances between the cells and nasal secretions. Ciliated cells typically contain around 100 cilia each, which measure 0.3 μm wide by 5 μm long. Cilia are located in the posterior two-thirds of the nose from the inferior turbinate, with epithelium covering the posterior part of the nasal cavity. The paranasal sinuses are densely covered by cilia. It has been stated that the distribution of ciliated columnar cells relates to the route of nasal airflow and that the linear velocity of inspired air is inversely proportional to the concentration of ciliated cells [51]. The entire respiratory region is coated with goblet cells. These are unicellular mucous glands, which are responsible for supplying the surface with viscous mucus. An adult respires about 105 litres of air per day, which must be heated and humidified before it enters the lungs. The humidification provided by the nasal mucosa raises the relative humidity (RH) to 95% before the air reaches the nasopharynx, aided by turbulent, mixing airflows. Through heat exchange in the sinuses, the nasal mucosa will attempt to maintain an air temperature of 31–37 °C. Approximately 10% of the heat exchange occurs in the external nares but most of the heat exchange occurs in a countercurrent heat exchange between the splenopalatine artery and airflow over the turbinates. Inspiration of air follows the generation of a negative pressure at an outflow boundary, that is the pharynx, and the pattern of deposition results from unequal airflows through the turbinates. The characteristics of the generated flume of liquid or powder are important, such as the initial inertial angle of application and cone angle [52]. The nasal structures provide resistance to entry, which contributes about half of the airway’s resistance. The four nasal limiting segments, the external and internal nasal valves, the septal valve and the inferior turbinates, contribute this resistance. A narrow or extra-wide nasal passage will affect the proportion of turbulent and laminar airflow. The inferior turbinates go through a cycle of vasodilatation and vasoconstriction, with a period of around four hours. This is known as the nasal cycle and alternates between one nostril and the other [53]. Old studies suggest that the amplitude of the nasal cycle is exaggerated in infection [54]; Eccles concluded that periodic congestion and decongestion of the sinusoids contributed to a mechanism of generation of plasma exudates that is an important component of respiratory defence. Soane and colleagues used a double isotope scintigraphy technique to assess the rate of mucociliary clearance at the morning peak of the nasal cycle in a small group of individuals [55]. The measured clearance times for the patent versus the obstructed nostril were statistically significant, even in the small number of subjects in this study (n = 5). The ratio was approximately 2.5 : 1. Nasal patency can be detected by sonography. Tahimiler and colleagues have used a remote evaluation system to monitor twenty individuals whilst working in call centres [56]. Nasal acoustic measurements suggest that the cyclic changes are much faster with periods between 20 and 150 minutes. Nasal congestion is often associated with sleep-related disorders particularly in snoring. Young and colleagues examined more than 4000 questionnaires looking at the history of nasal congestion and sleep problems [57]. Patients who had congestion due to allergy were 1.8 times more likely to have moderate issues with apnoea and hypopnea suggesting sleep-disordered breathing. The mucous membranes constantly secrete mucus, which is a watery fluid rich in sialylated proteins. The glands secreting the fluids are of a mixed type comprising serous and mucous types. The system can be stimulated by cold dry air.
Drug Delivery Across the Nasal Mucosa
3.1 Introduction
Active compound
Licensed indication
Unlicensed indication
Aspirin
Mild to moderate pain, pyrexia
Nasal polyps [5]
Atropine sulfate
IV or IO dosing for treatment of organophosphorus poisoning
IN dosing for treatment of organophosphorus poisoning [6]
Salmon calcitonin
Osteoporosis in post-menopausal women
Osteoporotic vertebral fracture [7]
Epinephrine
IV or IO dosing for cardiac resuscitation, bronchial asthma, acute allergic reaction
IN dosing for cardiac resuscitation [8]
Fentanyl citrate
Breakthrough pain relief for cancer patients
Post-operative pain [9]
Glucagon
IM dosing for treatment of hypoglycaemia
IN dosing for treatment of hypoglycaemia [10–14]
Ibuprofen
Pain, inflammation, flu, colds and fever
Polyposis in children with cystic fibrosis [15]
Lorazepam
Anxiety
Seizures in children [16–18]
Meclizine dihydrochloride
Oral dosing for treatment of motion sickness, vertigo, nausea and vomiting
IN dosing for treatment of motion sickness, vertigo, nausea and vomiting [19]
Midazolam
For use as a sedative, anxiety treatment or anaesthesia medication
Naloxone hydrochloride
IV dosing for opioid overdose
IN dosing for opioid overdose [33]
3.2 Drug Delivery via the Nasal Mucosa
3.2.1 Drugs Administered for Local Action
Product name
Active compound
Form
Manufacturer
Indication
Source of data
Beconase
Beclometasone dipropionate
Spray
GSK
Seasonal allergic rhinitis
Manufacturer’s site
Flixonase
Fluticasone Propionate
Spray
GSK
Rhinitis
Manufacturer’s site
Nasacort
Triamcinolone acetonide
Spray
Sanofi-Aventis
Rhinitis
PDR
Nasonex
Mometasone furoate monohydrate
Spray
Schering-Plough
Rhinitis
PDR
Otrivine
Xylometazoline
Spray/Drops
Novartis
Rhinitis/sinusitis
EMC
Rinatec
Ipatropium bromide
Spray
Boehrinher Ingelheim
Rhinitis
PDR
Rhinocort
Budesonide
Spray
AstraZeneca
Allergic rhinitis/nasal polyps
PDR
Rhinolast
Budesonide
Spray
Meda
Rhinitis
EMC
Rynacrom
Sodium cromoglicate
Spray
Sanofi-Aventis
Rhinitis
EMC
Bactoban
Mupirocin calcium
Ointment
GSK
Elimination nasal staphylococci
EMC
Naseptin
Chlorhexidine hydrochloride and neomycin sulphate
Cream
Alliance
Elimination nasal staphylococci
EMC
Imigran
Sumatriptan
Spray
GSK
Migraine
EMC
Zomig
Zolmitriptan
Spray
AstraZeneca
Migraine
PDR
Instanyl
Fentanyl Citrate
Spray
Nycomed
Breakthrough pain
EMC
Miacalcic
Calcitonin
Spray
Novartis
Osteoporosis
PDR/EMC
Suprecur
Burserelin acetate
Spray
Sanofi-Aventis
Endometriosis/pituitary desensitisation
EMC
Suprefact
Burserelin acetate
Spray
Sanofi-Aventis
Prostatic carcinoma
EMC
Synarel
Nafarelin
Spray
Pharmacia
Endometriosis/ovarian stimulation
EMC
Desmospray
Desmopressin
Spray
Ferring
MS/Vasopressinsensitive cranial diabetes insipdus/renal concentration capacity
EMC
Nicorette
Nicotine
Spray
Pharmacia
Nicotine withdrawal
EMC
Flumist
Influenza virus vaccine
Spray
Medimmune vaccines
Influenza vaccine
PDR
* PDR = Physician’s Desk Reference, EMC = Electronic Medicines Compendium.
3.2.2 Drugs Administered for Systemic Effect
3.2.3 Peptide and Protein Delivery
3.3 Anatomy and Physiology of the Nasal Cavity
3.3.1 Structure and Function of the Nasal Cavity
3.3.2 Nasal Epithelia
3.3.3 Airflow
3.3.4 Nasal Secretions
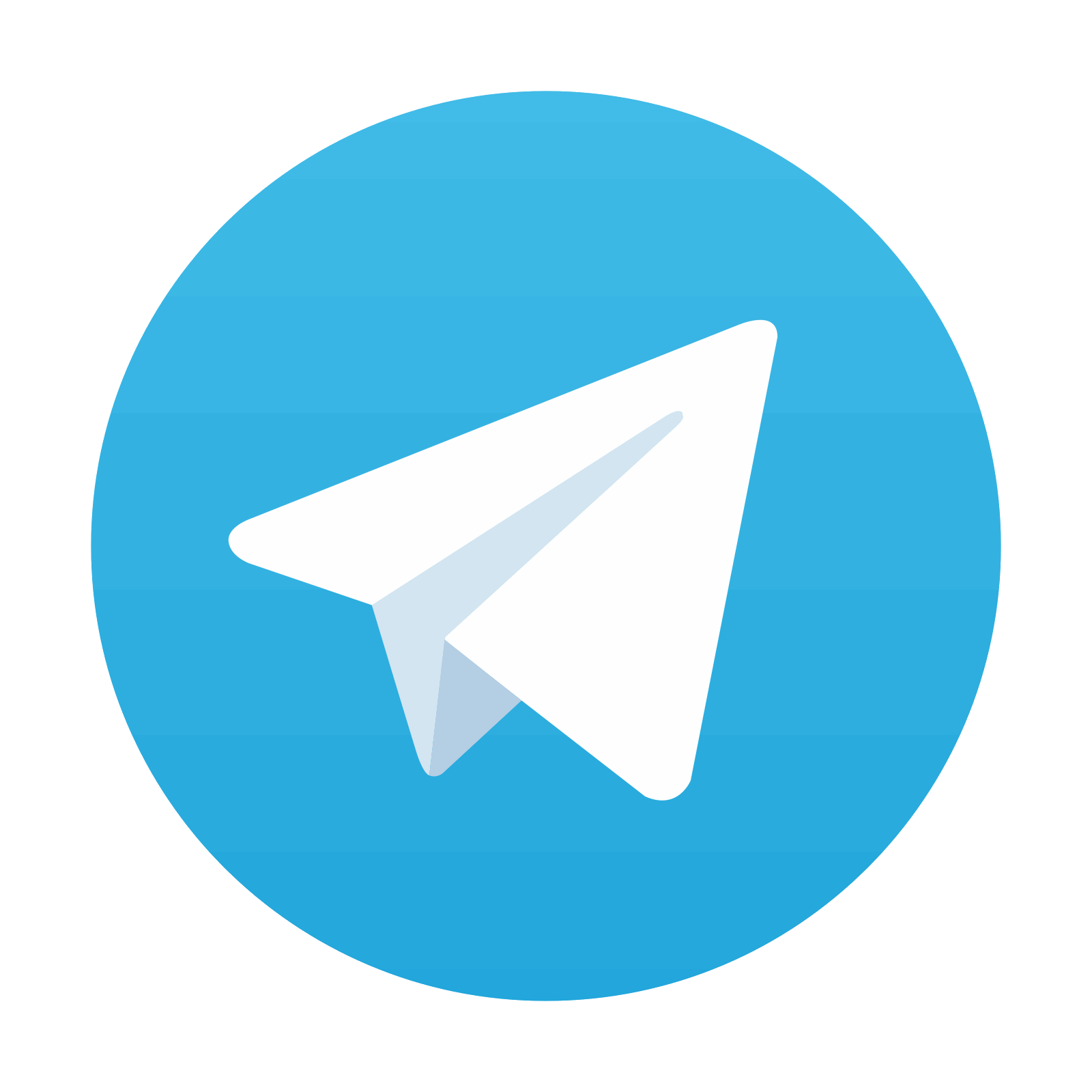
Stay updated, free articles. Join our Telegram channel

Full access? Get Clinical Tree
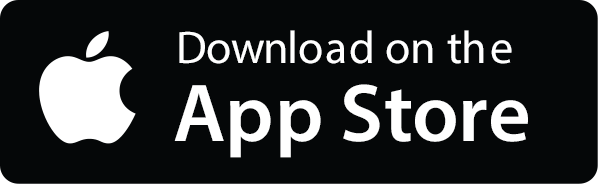
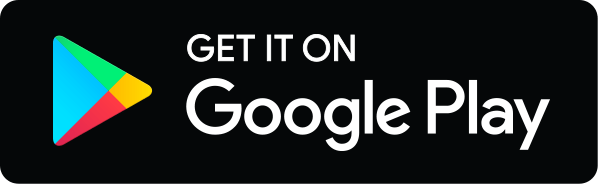