Chapter 21 Diuretic Drugs
Abbreviations | |
---|---|
ATP | Adenosine triphosphate |
ECF | Extracellular fluid |
ENaC | Epithelial Na+ channel |
GFR | Glomerular filtration rate |
GI | Gastrointestinal |
IV | Intravenous |
NCC | Na+/Cl− cotransporter protein |
NHE3 | Na+/H+ exchange (antiport) protein |
NKCC2 | Na+/K+/2Cl− cotransporter protein |
Therapeutic Overview
not a true tolerance but results from activation of compensatory salt-retaining mechanisms. Specifically, contraction of the ECF volume activates the sympathetic nervous system (see Chapter 19), with a resultant increase in release of angiotensin II, aldosterone, and antidiuretic hormone that may lead to a compensatory increase in Na+ reabsorption. Moreover, continued delivery of Na+ to more distal nephron segments induced by loop diuretics,
Therapeutic Overview | |
---|---|
Goal: To increase excretion of salt and water | |
Thiazide Diuretics | K+-sparing Diuretics |
Hypertension | Chronic liver failure |
Congestive heart failure (mild) | Congestive heart failure, when hypokalemia is a problem |
Renal calculi | Carbonic Anhydrase Inhibitors |
Nephrogenic diabetes insipidus | Cystinuria (to alkalinize tubular urine) |
Chronic renal failure (as an adjunct to loop diuretic) | Glaucoma (to decrease intraocular pressure) |
Osteoporosis | Periodic paralysis that affects muscle membrane function |
Loop Diuretics | Acute mountain sickness (to counteract respiratory alkalosis) |
Hypertension, in patients with impaired renal function | Metabolic alkalosis |
Congestive heart failure (moderate to severe) | Osmotic Diuretics |
Acute pulmonary edema | Acute or incipient renal failure |
Chronic or acute renal failure | Reduce intraocular or intracranial pressure (preoperatively) |
Nephrotic syndrome | |
Hyperkalemia | |
Chemical intoxication (to increase urine flow) |
Mechanisms of Action
All diuretics promote natriuresis and diuresis to reduce ECF volume; however, their mechanisms and sites of action differ. The five major types of diuretics are listed in Table 21-1, and their primary sites of action on the nephron are depicted in Figure 21-1. Knowledge of the mechanisms and sites of action of these agents is important in selecting an appropriate drug and anticipating and preventing complications. In addition, because each class of drugs exerts effects at specific targets, a combination of two or more drugs will often result in additive or synergistic effects to affect the reabsorption or excretion of Na+, Cl−, , water, and, to some extent, K+, H+, and organic ions. To understand the mechanisms and consequences of the actions of the diuretics, a basic knowledge of renal physiology is essential.
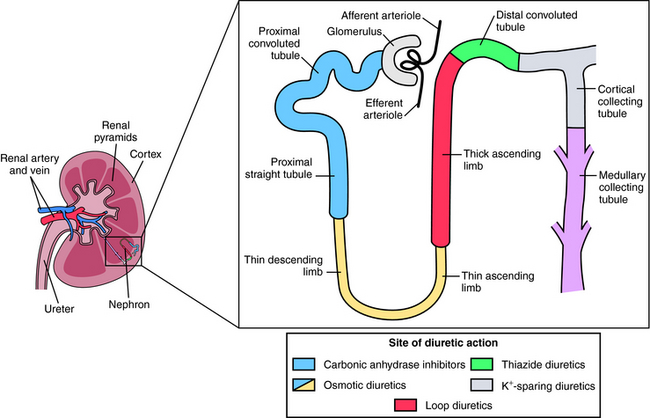
FIGURE 21–1 The nephron, depicting its location, segments, and the sites of action of different classes of diuretics.
Renal Epithelial Transport and Nephron Function
The normal human glomerular filtration rate (GFR) is approximately 180 L/day. Assuming a normal plasma Na+ concentration of 140 mmol/L, that means that 25,200 mmol of Na+ are filtered each day. To maintain Na+ balance, the kidney must reabsorb more than 99% (24,950 mmol) of the filtered load of Na+. This staggering amount of solute and water reabsorption is achieved by the actions of the million nephrons in each human kidney. Renal epithelial cells transport solute and water from the apical cell membrane to the basolateral cell membrane. The polarization of structures that differentiate the apical membrane from the basolateral membrane allows the vectorial transport of solute and water (Fig. 21-2). The basolateral cell membrane expresses the ubiquitous Na+/K+-adenosine triphosphatase (ATPase) (the Na+ pump) that exchanges 3 Na+ ions for 2 K+ ions, resulting in decreased intracellular Na+ providing a chemical gradient and an electronegative cell interior for Na+ entry from the lumen through the apical membrane (a potential difference of approximately 60 mV), which also attracts Na+. The concentration gradient then favors passive efflux of the K+ that entered the cell to the intercellular space. Because the ECF concentration of K+ is low relative to Na+, a recycling of K+ between the cell and interstitial fluid is necessary to maintain the Na+ pump.
The transport of Na+ across the apical cell membrane adjacent to the tubular fluid is achieved by passive diffusion via proteins that form a pore or channel (see Fig. 21-2, A), and two types of carrier-mediated transport, a cotransport (symport) pathway that transports Na+ and another solute species (such as Cl− or amino acids) in the same direction (see Fig. 21-2, B), and a countertransport (antiport) pathway that transports Na+ and another solute species (H+) in the opposite direction (see Fig. 21-2, C). In each case the low intracellular Na+ concentration as a consequence of the action of the Na+/K+-ATPase pump provides the electrochemical gradient for Na+ entry.
This transepithelial transport causes the osmolality of the lateral intercellular spaces to increase as a result of the accumulation of solute, producing an osmotic gradient that permits water to flow by two routes (see Fig. 21-2):
Tubular Reabsorption: Transport by the Proximal Tubule
The GFR of healthy adults ranges from 1.7 to 1.8 mL/min/kg, and approximately two thirds of the water and NaCl filtered at the glomerulus is reabsorbed by the proximal tubule. , glucose, amino acids, and other organic solutes are also reabsorbed. When GFR increases, salt and water excretion increases, but fractional reabsorption in the proximal tubule does not change. This is termed glomerulotubular balance. It moderates but does not entirely eliminate the effects of alterations in the GFR on salt and water excretion.
The transport of Na+, , and Cl− is important for the actions of several diuretics on the proximal tubule. Na+ is reabsorbed primarily with
in the early proximal tubule, whereas Na+ is reabsorbed primarily with Cl− in the late proximal tubule (Fig. 21-3). At the apical cell membrane of the early proximal tubule, Na+ entry is coupled with H+ efflux via the Na+/H+ antiporter (NHE3), which is a protein containing 10 to 12 transmembrane spanning domains and a hydrophilic C-terminal domain and is subject to regulation by a variety of factors, including angiotensin II, which increases its activity. H+ extruded from the cell combines with
to form H2CO3, which rapidly forms CO2 and water in the presence of the enzyme carbonic anhydrase. CO2 rapidly enters the cell via simple diffusion and is rehydrated to form carbonic acid. Because the concentration of cellular H+ is low, the reaction proceeds as follows: CO2 + H2O → H2CO3 → H+ +
. Thus a constant supply of H+ is furnished for countertransport with Na+.
that accumulates is cotransported with Na+ across the basolateral cell membrane into the interstitial fluid and, subsequently, into the blood. Although the cytoplasmic hydration reaction occurs spontaneously, the rate is inadequate to allow reabsorption of the
load filtered (approximately 4000 mEq/day). However, little or none of the filtered
is excreted because of the presence of carbonic anhydrase. The net effect of coupling of the Na+/H+ antiporter to the carbonic anhydrase-mediated hydration and rehydration of CO2 is preservation of
. The final step is its transfer from the interstitial fluid into peritubular capillaries.
In the late proximal tubule (see Fig. 21-3), Na+ is reabsorbed primarily with Cl−. Reabsorption is secondary to activation of both the NHE3, which couples inward Na+ transport with outward H+ transport, and a Cl− base (formate) exchanger that transports Cl− from lumen to cell in exchange for a base. The parallel operation of both exchangers results in net Na+ and Cl− absorption by the late proximal tubule. Passive transport of Na+ and Cl− also occurs between cells through the paracellular pathway.
In summary, the proximal tubule reabsorbs approximately 70% of filtered water, Na+, and Cl−, 85% of filtered , and 50% of filtered K+ (Table 21-2). These percentages are relatively constant, even when filtered quantities increase or decrease. As a result, minor fluctuations in GFR do not influence fluid and electrolyte excretion very much. The driving force for reabsorption of water and electrolytes is the Na+/K+-ATPase. Passive movements of other ions and water are initiated and sustained by active transport of Na+ across basolateral cell membranes. Osmotic equilibrium with plasma is maintained to the end of the proximal tubule. Most of the filtered
is not actually reabsorbed directly from the lumen; rather, it is converted to CO2 and water in the vicinity of the brush border membranes, within which large concentrations of carbonic anhydrase are located. The direction of this reaction is H2CO3 → CO2 + H2O (established by the high concentration of carbonic acid in luminal fluid resulting from the secretion of H+). Carbonic anhydrase in the cytoplasm catalyzes formation of carbonic acid. Cellular H+ is then exchanged for luminal Na+, and
is reabsorbed across the basolateral cell membranes. In this indirect way, filtered
is reabsorbed.
Tubular Reabsorption: Transport by the Loop of Henle
In contrast, the thick ascending limb and its transport functions are an important site of action of the loop (also called high-ceiling) diuretics (Fig. 21-4). Approximately 25% to 35% of filtered Na+ and Cl− is reabsorbed by the loop of Henle. The Na+/K+-ATPase in the basolateral membrane provides the gradient for Na+ and Cl− absorption. Na+ entry across the apical membrane is mediated by an electroneutral transport protein that binds one Na+, one K+, and two Cl− ions and is referred to as the Na+/K+/2Cl− (NKCC2) cotransporter. Although the ascending limb is highly permeable to Na+, K+, and Cl−, it is impermeable to water. Thus the continuous reabsorption of these ions without reabsorption of water dilutes the luminal fluid, thus the name diluting segment. Na+ entry down an electrochemical gradient drives the uphill transport of K+ and Cl−. This system depends on the simultaneous presence of these three ions in the luminal fluid. Once inside the cell, K+ passively reenters the lumen (K+ recycling) via conductive K+ channels in the apical membrane. Cl−, on the other hand, exits the cell via conductive Cl− channels in the basolateral membrane. Depolarization of the basolateral membrane occurs as a consequence of Cl− efflux, creating a lumen-positive (relative to the interstitial fluid) transcellular potential difference of approximately 10 mV. This drives paracellular cation transport, including Na+, Ca++, and Mg++. Inhibition of the NKCC2 cotransporter not only results in excretion of Na+ and Cl− but also in excretion of divalent cations, such as Ca++ and Mg++.
Tubular Reabsorption: Transport by the Distal Convoluted Tubule
In contrast to the proximal tubule and loop of Henle, there is less reabsorption of water and electrolytes in the distal convoluted tubule. It reabsorbs approximately 10% of the filtered load of NaCl. Similar to the thick ascending limb, this segment is impermeable to water, and the continuous reabsorption of NaCl further dilutes tubular fluid. NaCl entry across the apical membrane is mediated by the electroneutral NCC cotransporter sensitive to thiazide diuretics (Fig. 21-5). Unlike the NKCC2 cotransporter of the thick ascending limb, this cotransporter does not require participation of K+. As in other segments, the basolateral Na+/K+-ATPase provides the low intracellular Na+ concentration that facilitates downhill transport of Na+. The distal tubule does not have a pathway for K+ recycling, and therefore the transepithelial voltage is near zero. Therefore the reabsorption of Ca++ and Mg++ is not driven by electrochemical forces. Instead, Ca++ crosses the apical membrane via a Ca++ channel and exits the basolateral membrane via the NCC exchanger. Thus, by inhibiting the NCC cotransporter, thiazide diuretics indirectly affect Ca++ transport through changes in intracellular Na+. Another mechanism of increased Ca++ reabsorption with thiazide diuretics is an increase in the intracellular concentrations of Ca++-binding proteins.
Recently, inactivating mutations have been found in the human gene encoding the NCC transporter in patients with Gitelman’s syndrome, characterized by hypotension, hypokalemia, hypomagnesemia, and hypocalciuria, similar to the effects of thiazides. Pseudohypoaldosteronism type II is an autosomal dominant disease characterized by hypertension, hyperkalemia, and sensitivity to thiazide diuretics. It has been suggested that an activating mutation of the NCC cotransporter is responsible. Recently, two protein kinases have also been linked to the pathogenesis of this syndrome. They are found in the distal nephron and are thought to control the activity of the cotransporters.
Tubular Reabsorption: Transport by the Collecting Tubule
The collecting tubule is the final site of Na+ reabsorption, and approximately 3% of filtered Na+ is reabsorbed by this segment. Although the collecting tubule reabsorbs only a small percentage of the filtered load, two characteristics are important for diuretic action. First, this segment is the site of action of aldosterone, a hormone controlling Na+ reabsorption and K+ secretion (see Chapter 39). Second, virtually all K+ excreted results from its secretion by the collecting tubule. Thus the collecting tubule contributes to the hypokalemia induced by diuretics.
The collecting tubule is composed of two cell types with separate functions. Principal cells are responsible for the transport of Na+, K+, and water, whereas intercalated cells are primarily responsible for the secretion of H+ or . Intercalated cells are of two types, A and B, the former responsible for secretion of H+ via an H+-ATPase (primary active ion pump) in the apical cell membrane, and the latter responsible for secretion of
via a Cl−/
exchanger in the apical membrane. In contrast to more proximal cells, the apical membrane of principal cells does not express cotransport or countertransport systems; rather it expresses separate channels that permit selective conductive transport of Na+ and K+ (Fig. 21-6). Na+ is reabsorbed through a conductive Na+ channel. The low intracellular Na+ as a result of the basolateral Na+/K+-ATPase generates a favorable electrochemical gradient for Na+ entry through epithelial Na+ channels (ENaCs). Because Na+ channels are present only in the apical cell membrane of principal cells, Na+
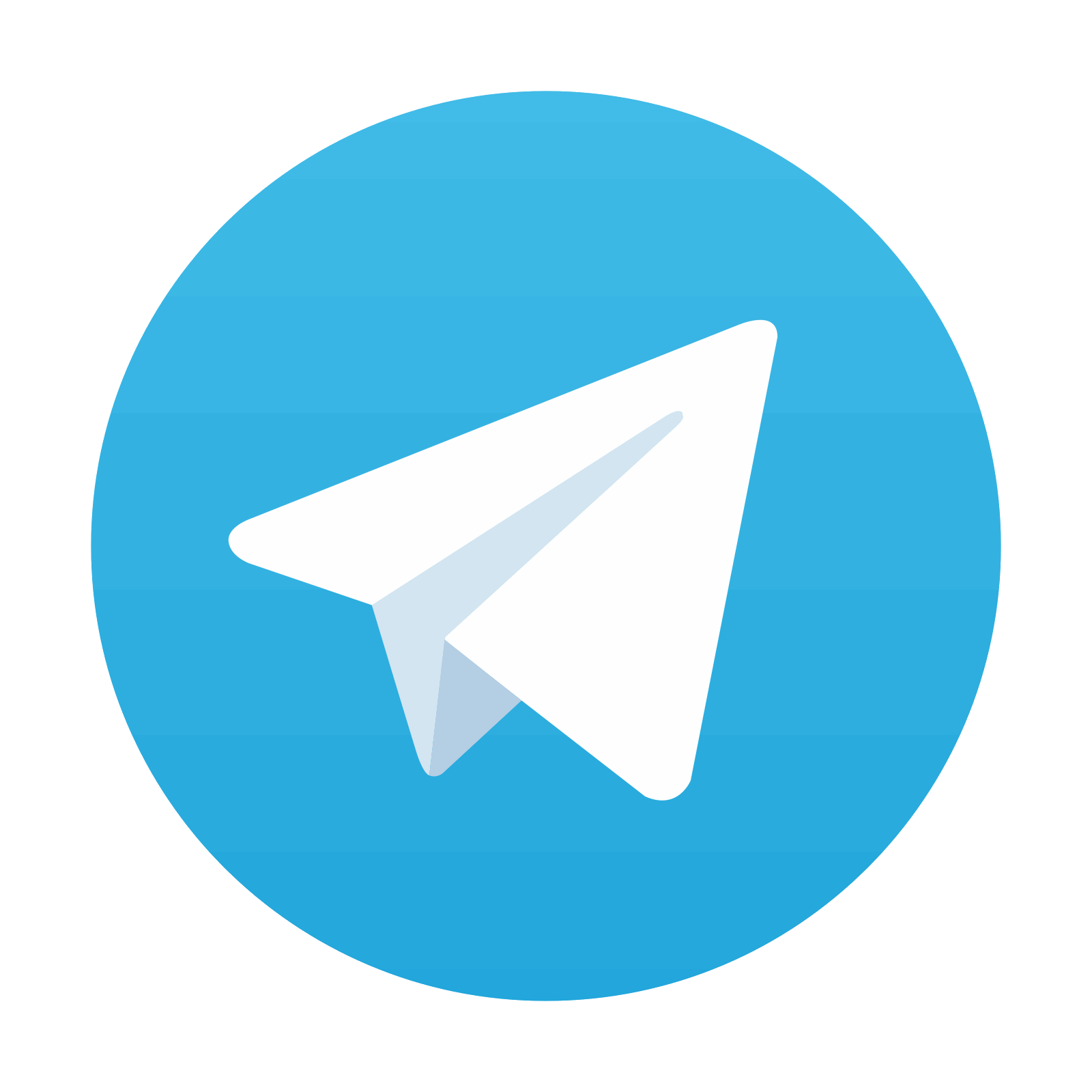
Stay updated, free articles. Join our Telegram channel

Full access? Get Clinical Tree
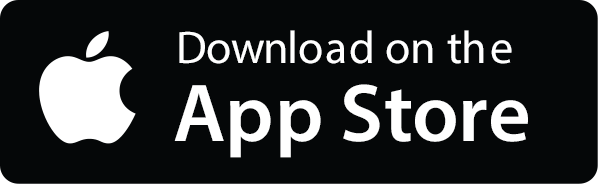
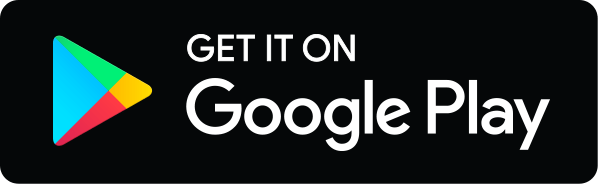
