Carbohydrates are the largest source of dietary calories for most of the world’s population. The major carbohydrates in the U.S. diet are starch, lactose, and sucrose. The starches amylose and amylopectin are polysaccharides composed of hundreds to millions of glucosyl units linked together through α-1,4- and α-1,6-glycosidic bonds (Fig. 21.1). Lactose is a disaccharide composed of glucose and galactose, linked together through a β-1,4-glycosidic bond. Sucrose is a disaccharide composed of glucose and fructose, linked through an α-1,2-glycosidic bond. The digestive processes convert all of these dietary carbohydrates to their constituent monosaccharides by hydrolyzing glycosidic bonds between the sugars.
FIGURE 21.1 The structures of common dietary carbohydrates. For disaccharides and higher, the sugars are linked through glycosidic bonds between the anomeric carbon of one sugar and a hydroxyl group on another sugar. The glycosidic bond may be either α or β, depending on its position above or below the plane of the sugar containing the anomeric carbon. (See Chapter 5, Section II.A, to review terms used in the description of sugars.) The starch amylose is a polysaccharide of glucose residues linked with α-1,4-glycosidic bonds. Amylopectin is amylose with the addition of α-1,6-glycosidic branch points. Dietary sugars may be monosaccharides (single sugar residues), disaccharides (two sugar residues), oligosaccharides (several sugar residues), or polysaccharides (hundreds of sugar residues). For clarity, the hydrogen atoms are not shown in the figure.
The digestion of starch begins in the mouth (Fig. 21.2). The salivary gland releases α-amylase, which converts starch to smaller polysaccharides called α-dextrins. Salivary α-amylase is inactivated by the acidity of the stomach (hydrochloric acid [HCl]). Pancreatic α-amylase and bicarbonate are secreted by the exocrine pancreas into the lumen of the small intestine, where bicarbonate neutralizes the gastric secretions. Pancreatic α-amylase continues the digestion of α-dextrins, converting them to disaccharides (maltose), trisaccharides (maltotriose), and oligosaccharides called limit dextrins. Limit dextrins usually contain four to nine glucosyl residues and an isomaltose branch (two glucosyl residues attached through an α-1,6-glycosidic bond).
FIGURE 21.2 Overview of carbohydrate digestion. Digestion of the carbohydrates occurs first, followed by absorption of monosaccharides. Subsequent metabolic reactions occur after the sugars are absorbed.
The digestion of the disaccharides lactose and sucrose, as well as further digestion of maltose, maltotriose, and limit dextrins, occurs through disaccharidases attached to the membrane surface of the brush border (microvilli) of intestinal epithelial cells. Glucoamylase hydrolyzes the α-1,4-bonds of dextrins. The sucrase–isomaltase complex hydrolyzes sucrose, most of maltose, and almost all of the isomaltose formed by glucoamylase from limit dextrins. Lactase-glycosylceramidase (β-glycosidase) hydrolyzes the β-glycosidic bonds in lactose and glycolipids. A fourth disaccharidase complex, trehalase, hydrolyzes the bond (an α-1,1-glycosidic bond) between two glucosyl units in the sugar trehalose. The monosaccharides produced by these hydrolases (glucose, fructose, and galactose) are then transported into the intestinal epithelial cells.
Dietary fiber, composed principally of polysaccharides, cannot be digested by human enzymes in the intestinal tract. In the colon, dietary fiber and other nondigested carbohydrates may be converted to gases (H2, CO2, and methane) and short-chain fatty acids (principally acetic acid, propionic acid, and butyric acid) by bacteria in the colon.
Glucose, galactose, and fructose formed by the digestive enzymes are transported into the absorptive epithelial cells of the small intestine by protein-mediated Na+-dependent active transport and facilitative diffusion. Monosaccharides are transported from these cells into the blood and circulate to the liver and peripheral tissues, where they are taken up by facilitative transporters. Facilitative transport of glucose across epithelial cells and other cell membranes is mediated by a family of tissue-specific glucose transport proteins (GLUT I to GLUT V). The type of transporter found in each cell reflects the role of glucose metabolism in that cell.
THE WAITING ROOM 
Denise V. is a 20-year-old exchange student from Nigeria who has noted gastrointestinal bloating, abdominal cramps, and intermittent diarrhea ever since arriving in the United States 6 months ago. A careful history shows that these symptoms occur most commonly about 45 minutes to 1 hour after eating breakfast but may occur after other meals as well. Dairy products, which were not a part of Denise V.’s diet in Nigeria, were identified as the probable offending agent because her gastrointestinal symptoms disappeared when milk and milk products were eliminated from her diet.
Deborah S.’s fasting and postprandial blood glucose levels are frequently above the normal range in spite of good compliance with insulin therapy. Her physician has referred her to a dietician skilled in training diabetic patients in the successful application of an appropriate American Diabetes Association diet. As part of the program, Deborah S. is asked to incorporate foods containing fiber into her diet, such as whole grains (e.g., wheat, oats, corn), legumes (e.g., peas, beans, lentils), tubers (e.g., potatoes, peanuts), and fruits.
Nina M. is a 13-month-old baby girl, the second child born to unrelated parents. Her mother had a healthy, full-term pregnancy, and Nina M.’s birth weight was normal. She did not respond well to breastfeeding and was changed entirely to a formula based on cows’ milk at 6 weeks. Between 9 and 18 weeks of age, she was admitted to the hospital twice with a history of screaming after feeding but was discharged after observation without a specific diagnosis. Elimination of cows’ milk from her diet did not relieve her symptoms; Nina M.’s mother reported that when Nina M. turned 1 year old she introduced some fruit juice into her diet the screaming bouts were worse, particularly after drinking juice. She also noticed that Nina M. frequently had gas and a distended abdomen. She was still thriving (weight > 97th percentile), with no abnormal findings on physical examination. A stool sample was taken.
I. Dietary Carbohydrates
Carbohydrates are the largest source of calories in the average American diet and usually constitute 40% to 45% of our caloric intake. The plant starches amylopectin and amylose, which are present in grains, tubers, and vegetables, constitute approximately 50% to 60% of the carbohydrate calories consumed. These starches are polysaccharides, containing 10,000 to 1 million glucosyl units. In amylose, the glucosyl residues form a straight chain linked via α-1,4-glycosidic bonds; in amylopectin, the α-1,4-chains contain branches connected via α-1,6-glycosidic bonds (see Fig. 21.1). The other major sugar found in fruits and vegetables is sucrose, a disaccharide of glucose and fructose (see Fig. 21.1). Sucrose and small amounts of the monosaccharides glucose and fructose are the major natural sweeteners found in fruit, honey, and vegetables. Dietary fiber, the part of the diet that cannot be digested by human enzymes of the intestinal tract, is also composed principally of plant polysaccharides and a polymer called lignin.
Most foods derived from animals, such as meat or fish, contain very little carbohydrate except for small amounts of glycogen (which has a structure similar to amylopectin) and glycolipids. The major dietary carbohydrate of animal origin is lactose, a disaccharide composed of glucose and galactose that is found exclusively in milk and milk products (see Fig. 21.1). Sweeteners, in the form of sucrose and high-fructose corn syrup (starch, partially hydrolyzed and isomerized to fructose), also appear in the diet as additives to processed foods. On average, a person in the United States consumes 65 lb of added sucrose and 40 lb of high-fructose corn syrup solids per year.
Although all cells require glucose for metabolic functions, neither glucose nor other sugars are specifically required in the diet. Glucose can be synthesized from many amino acids found in dietary protein. Fructose, galactose, xylulose, and all the other sugars required for metabolic processes in the human can be synthesized from glucose.
II. Digestion of Dietary Carbohydrates
In the digestive tract, dietary polysaccharides and disaccharides are converted to monosaccharides by glycosidases, enzymes that hydrolyze the glycosidic bonds between the sugars. All of these enzymes exhibit some specificity for the sugar, the glycosidic bond (α or β), and the number of saccharide units in the chain. The monosaccharides formed by glycosidases are transported across the intestinal mucosal cells into the interstitial fluid and subsequently enter the bloodstream. Undigested carbohydrates enter the colon, where bacteria may ferment them.
A. Salivary and Pancreatic α-Amylase
The digestion of starch (amylopectin and amylose) begins in the mouth, where chewing mixes the food with saliva. The salivary glands secrete approximately 1 L of liquid per day into the mouth, containing salivary α-amylase and other components. α-Amylase is an endoglycosidase, which means that it hydrolyzes internal α-1,4-bonds between glucosyl residues at random intervals in the polysaccharide chains (Fig. 21.3). The shortened polysaccharide chains that are formed are called α-dextrins. Salivary α-amylase is largely inactivated by the acidity of the stomach contents, which contain HCl secreted by the parietal cells.
FIGURE 21.3 Action of salivary and pancreatic α-amylases.
The acidic gastric juice enters the duodenum, the upper part of the small intestine, where digestion continues. Secretions from the exocrine pancreas (~1.5 L/day) flow down the pancreatic duct and also enter the duodenum. These secretions contain bicarbonate (HCO3−), which neutralizes the acidic pH of stomach contents, and digestive enzymes, including pancreatic α-amylase.
Pancreatic α-amylase continues to hydrolyze the starches and glycogen, forming the disaccharide maltose, the trisaccharide maltotriose, and oligosaccharides. These oligosaccharides, called limit dextrins, are usually four to nine glucosyl units long and contain one or more α-1,6-branches. The two glucosyl residues that contain the α-1,6-glycosidic bond eventually become the disaccharide isomaltose.
α-Amylase has no activity toward sugar-containing polymers other than glucose linked by α-1,4-bonds. α-Amylase displays no activity toward the α-1,6-bond at branch points and has little activity for the α-1,4-bond at the nonreducing end of a chain.
B. Disaccharidases of the Intestinal Brush-Border Membrane
The dietary disaccharides lactose and sucrose, as well as the products of starch digestion, are converted to monosaccharides by glycosidases attached to the membrane in the brush border of absorptive cells. The different glycosidase activities are found in four glycoproteins: glucoamylase, the sucrase–isomaltase complex, the smaller glycoprotein trehalase, and lactase-glucosylceramidase (Table 21.1). These glycosidases are collectively called the small intestinal disaccharidases, although glucoamylase is really an oligosaccharidase.
TABLE 21.1 The Different Forms of the Brush-Border Glycosidases
COMPLEX | CATALYTIC SITES | PRINCIPAL ACTIVITIES |
β-Glucoamylase | α-Glucosidase | Split α-1,4-glycosidic bonds between glucosyl units, beginning sequentially with the residue at the tail end (nonreducing end) of the chain. This is an exoglycosidase. Substrates include amylose, amylopectin, glycogen, and maltose. |
β-Glucosidase | Same as above, but with slightly different specificities and affinities for the substrates | |
Sucrase–isomaltase | Sucrase–maltase | Splits sucrose, maltose, and maltotriose |
Isomaltase–maltase | Splits α-1,6-bonds in several limit dextrins, as well as the α-1,4-bonds in maltose and maltotriose | |
β-Glycosidase | Glucosyl–ceramidase | Splits β-glycosidic bonds between glucose or galactose and hydrophobic residues, such as the glycolipids glucosylceramide and galactosylceramide. Also known as phlorizin hydrolase for its activity on an artificial substrate |
Lactase | Splits the β-1,4-bond between glucose and galactose. To a lesser extent also splits the β-1,4-bond between some cellulose disaccharides | |
Trehalase | Trehalase | Splits bond in trehalose, which is two glucosyl units linked α-1,1 through their anomeric carbons |
1. Glucoamylase
Glucoamylase and the sucrase–isomaltase complex have similar structures and exhibit a great deal of sequence homogeneity. A membrane-spanning domain near the N-terminus attaches the protein to the luminal membrane. The long polypeptide chain forms two globular domains, each with a catalytic site. In glucoamylase, the two catalytic sites have similar activities, with only small differences in substrate specificity. The protein is heavily glycosylated, with oligosaccharides that protect it from digestive proteases.
Glucoamylase is an exoglycosidase that is specific for the α-1,4-bonds between glucosyl residues (Fig. 21.4A). It begins at the nonreducing end of a polysaccharide or limit dextrin, and it sequentially hydrolyzes the bonds to release glucose monosaccharides. It will digest a limit dextrin down to isomaltose, the glucosyl disaccharide with an α-1,6-branch, that is subsequently hydrolyzed principally by the isomaltase activity in the sucrase–isomaltase complex.
FIGURE 21.4 A. Glucoamylase activity. Glucoamylase is an α-1,4-exoglycosidase that initiates cleavage at the nonreducing end of the sugar. Thus, for maltotriose, the bond labeled 1 is hydrolyzed first, which then allows the bond at position 2 to be the next one hydrolyzed. B. Isomaltase activity. Arrows indicate the α-1,6-bonds that are cleaved. C. Trehalose. This disaccharide contains two glucose moieties linked by an unusual bond that joins their anomeric carbons. It is cleaved by trehalase.
2. Sucrase–Isomaltase Complex
The structure of the sucrase–isomaltase complex is similar to that of glucoamylase, and these two proteins have a high degree of sequence homology. However, after the single polypeptide chain of sucrase–isomaltase is inserted through the membrane and the protein protrudes into the intestinal lumen, an intestinal protease clips it into two separate subunits that remain attached to each other, forming a functional heterodimer. Each subunit has a catalytic site that differs in substrate specificity from the other through noncovalent interactions. The sucrase–maltase site accounts for approximately 100% of the intestine’s ability to hydrolyze sucrose in addition to maltase activity; the isomaltase–maltase site accounts for almost all of the intestine’s ability to hydrolyze α-1,6-bonds (see Fig. 21.4B), in addition to maltase activity. Together, these sites account for approximately 80% of the maltase activity of the small intestine. The remainder of the maltase activity is found in the glucoamylase complex.
3. Trehalase
Trehalase is only half as long as the other disaccharidases and has only one catalytic site. It hydrolyzes the glycosidic bond in trehalose, a disaccharide composed of two glucosyl units linked by an α-bond between their anomeric carbons (see Fig. 21.4C). Trehalose, which is found in insects, algae, mushrooms, and other fungi, is not currently a major dietary component in the United States. However, unwitting consumption of trehalose can cause nausea, vomiting, and other symptoms of severe gastrointestinal distress if consumed by an individual deficient in the enzyme. Trehalase deficiency was discovered when a woman became very sick after eating mushrooms and was initially thought to have α-amanitin poisoning.
4. α-Glycosidase Complex (Lactase-Glucosylceramidase)
The β-glycosidase complex is another large glycoprotein found in the brush border that has two catalytic sites extending in the lumen of the intestine. However, its primary structure is very different from that of the other enzymes, and it is attached to the membrane through its carboxyl end by a phosphatidylglycan anchor (see Fig. 10.5). The lactase catalytic site hydrolyzes the β-bond connecting glucose and galactose in lactose (a β-galactosidase activity; Fig. 21.5). The major activity of the other catalytic site in humans is the β-bond between glucose or galactose and ceramide in glycolipids (this catalytic site is sometimes called phlorizin hydrolase, named for its ability to hydrolyze an artificial substrate).
FIGURE 21.5 Lactase activity. Lactase is a β-galactosidase. It cleaves the β-galactoside lactose, the major sugar in milk, forming galactose and glucose.
5. Location within the Intestine
The production of maltose, maltotriose, and limit dextrins by pancreatic α-amylase occurs in the duodenum, the most proximal portion of the small intestine. Sucrase–isomaltase activity is highest in the jejunum, where the enzymes can hydrolyze sucrose and the products of starch digestion. β-Glycosidase activity is also highest in the jejunum. Glucoamylase activity increases progressively along the length of the small intestine, and its activity is highest in the ileum. Thus, it presents a final opportunity for digestion of starch oligomers that have escaped amylase and disaccharidase activities at the more proximal regions of the intestine.
C. Metabolism of Sugars by Colonic Bacteria
Not all of the starch ingested as part of foods is normally digested in the small intestine (Fig. 21.6). Starches that are high in amylose, or are less well-hydrated (e.g., starch in dried beans), are resistant to digestion and enter the colon. Dietary fiber and undigested sugars also enter the colon. Here, colonic bacteria rapidly metabolize the saccharides, forming gases, short-chain fatty acids, and lactate. The major short-chain fatty acids formed are acetic acid (two carbons), propionic acid (three carbons), and butyric acid (four carbons). The short-chain fatty acids are absorbed by the colonic mucosal cells and can provide a substantial source of energy for these cells. The major gases formed are hydrogen gas (H2), carbon dioxide (CO2), and methane (CH4). These gases are released through the colon, resulting in flatulence, or through the breath. Incomplete products of digestion in the intestines increase the retention of water in the colon, resulting in diarrhea.
FIGURE 21.6 Some indigestible carbohydrates. These compounds are components of dietary fiber.
D. Lactose Intolerance
Lactose intolerance refers to a condition of pain, nausea, and flatulence after the ingestion of foods containing lactose, most notably dairy products. Although lactose intolerance is often caused by low levels of lactase, it also can be caused by intestinal injury (defined in the following text).
1. Nonpersistent and Persistent Lactase
Lactase activity increases in the human from about 6 to 8 weeks of gestation, and it rises during the late gestational period (21 to 32 weeks) through full term. It remains high for about 1 month after birth and then begins to decline. For most of the world’s population, lactase activity decreases to adult levels at approximately 5 to 7 years of age. Adult levels are <10% of those present in infants. These populations have adult hypolactasia (formerly called adult lactase deficiency) and exhibit the lactase nonpersistence phenotype. In people who are derived mainly from Western Northern Europeans, and milk-dependent Nomadic tribes of Saharan Africa, the levels of lactase remain at, or only slightly below, infant levels throughout adulthood (lactase persistence phenotype). Thus, adult hypolactasia is the normal condition for most of the world’s population (Table 21.2).
TABLE 21.2 Prevalence of Late-Onset Lactase Deficiency
GROUP | PREVALENCE (%) |
U.S. Population | |
Asians | 100 |
American Indians (Oklahoma) | 95 |
Black Americans | 81 |
Mexican Americans | 56 |
White Americans | 24 |
Other Populations | |
Ibo, Yoruba (Nigeria) | 89 |
Italians | 71 |
Aborigines (Australia) | 67 |
Greeks | 53 |
Danes | 3 |
Dutch | 0 |
Data from Biller HA, Grand RS. Lactose intolerance. Annu Rev Med. 1990;41:141–148.
In contrast, congenital lactase deficiency is a severe autosomal-recessive inherited disease in which lactase activity is significantly reduced, or totally absent. The disorder presents as soon as the newborn is fed breast milk or lactose-containing formula, resulting in watery diarrhea, weight loss, and dehydration. Treatment consists of removal of lactose from the diet, which allows for normal growth and development to occur.
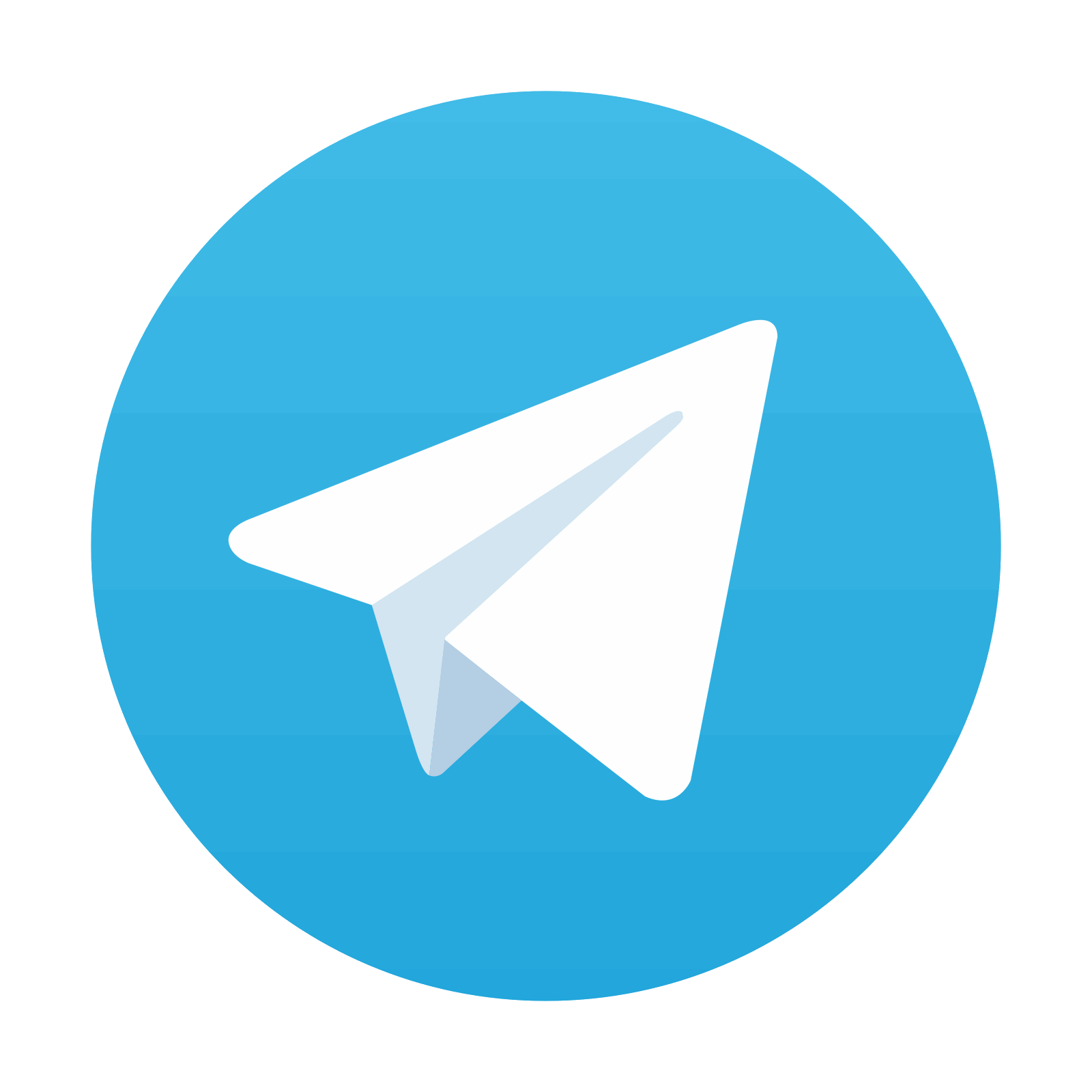
Stay updated, free articles. Join our Telegram channel

Full access? Get Clinical Tree
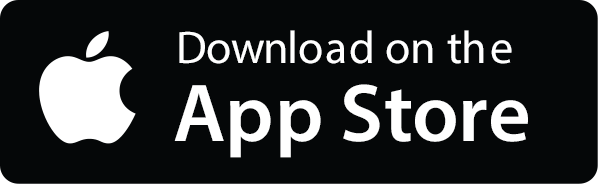
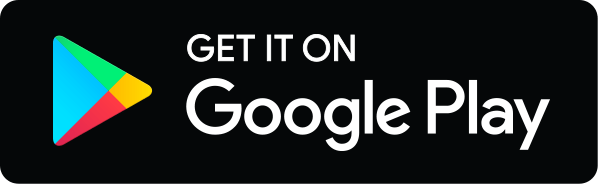