24 Central nervous system infections
Here, we discuss the main routes of central nervous system invasion by microorganisms (see also Ch. 13) and the body’s response, followed by a more detailed discussion of the diseases that result.
Invasion of the central nervous system
Natural barriers act to prevent blood-borne invasion
Blood-borne invasion takes place across:
• the blood–brain barrier to cause encephalitis
• the blood–cerebrospinal fluid (CSF) barrier to cause meningitis (Fig. 24.1).
The blood–brain barrier consists of tightly joined endothelial cells surrounded by glial processes, while the brain–CSF barrier at the choroid plexus consists of endothelium with fenestrations, and tightly joined choroid plexus epithelial cells. Microbes can traverse these barriers by:
• growing across, infecting the cells that comprise the barrier
• being passively transported across in intracellular vacuoles
Examples of each route are seen in viral infections. Poliovirus, for instance, invades the central nervous system (CNS) across the blood–brain barrier. After oral ingestion of virus, a complex stepwise series of events leads to CNS invasion (Fig. 24.2). Poliovirus also invades the meninges after localizing in vascular endothelial cells, and can cross the blood–CSF barrier. Mumps virus behaves in the same way, as do circulating Haemophilus influenzae, meningococci or pneumococci. Once infection has reached the meninges and CSF, the brain substance can in turn be invaded if the infection crosses the pia. In poliomyelitis, for instance, a meningitic phase often precedes encephalitis and paralysis.
Invasion of the CNS via peripheral nerves is a feature of herpes simplex, varicella-zoster and rabies virus infections
Herpes simplex virus (HSV) and varicella-zoster virus (VZV) present in skin or mucosal lesions (see Ch. 26), travel up axons using the normal retrograde transport mechanisms that can move virus particles (as well as foreign molecules such as tetanus toxin) at a rate of about 200 mm/day, to reach the dorsal root ganglia. Rabies virus, introduced into muscle or subcutaneous tissues by the bite of a rabid animal, infects muscle fibres and muscle spindles after the virus binds to the nicotinic acetylcholine receptor. It then enters peripheral nerves and travels to the CNS, to reach glial cells and neurones, where it multiplies.
The body’s response to invasion
CSF cell counts increase in response to infection
The response to invading viruses is reflected by an increase in lymphocytes, mostly T cells, and monocytes in the CSF (Table 24.1). A slight increase in protein also occurs, the CSF remaining clear. This condition is termed ‘aseptic’ meningitis. The response to pyogenic bacteria shows a more spectacular and more rapid increase in polymorphonuclear leukocytes and proteins (Fig. 24.3), so that the CSF becomes visibly turbid. This condition is termed ‘septic’ meningitis. Certain slower growing or less pyogenic microorganisms induce less dramatic changes, such as in tuberculous or listerial meningitis.
The pathologic consequences of CNS infection depend upon the microorganism
Viruses induce perivascular infiltration of lymphocytes and monocytes, sometimes, as in the case of polio, with direct damage to infected cells. (The pathogenesis of viral encephalomyelitis is shown later, in Fig. 24.7.) Associated immune responses not only to viral, but also often to host CNS components, play a part in postvaccinial encephalitis. Infiltrating B cells produce antibody to the invading microorganism, and T cells react with microbial antigens to release cytokines that attract and activate other T cells and macrophages. The pathologic condition evolves over the course of several days and occasionally, when partly controlled by host defences, over the course of years, e.g. subacute sclerosing panencephalitis (SSPE) caused by measles, which has both a virological and immunological pathogenesis. Bacteria cause more rapidly evolving pathologic changes, with local responses to bacterial antigens and toxins playing an important part.
CNS invasion only rarely assists in the transmission of infection
• When dorsal root ganglion neurones are invaded as an essential step in establishing latency (HSV and VZV). This gives a mechanism for reactivation and further episodes of shedding from mucosal or skin lesions.
• In the case of rabies (see below), where CNS invasion in the animal host is necessary for two reasons. First, it enables the virus to spread from the CNS down peripheral nerves to the salivary glands, from which transmission takes place. Second, invasion of the limbic system of the brain causes a change in behaviour of the infected animal so that it becomes less retiring, more aggressive and more likely to bite, thus transmitting the infection. Invasion of the limbic system can be regarded as a fiendish strategy on the part of rabies virus to promote its own transmission and survival.
Meningitis
Bacterial meningitis
Acute bacterial meningitis is a life-threatening infection, needing urgent specific treatment
Bacterial meningitis is more severe, but less common, than viral meningitis and may be caused by a variety of agents (Table 24.2). Prior to the 1990s, Haemophilus influenzae type b (Hib) was responsible for most cases of bacterial meningitis. However, the introduction of the Hib vaccine into childhood immunization regimens has lowered overall Hib incidence in favour of Neisseria meningitidis and Streptococcus pneumoniae, which are now responsible for most bacterial meningitis. These three pathogens have several virulence factors in common (Table 24.3), including possession of a polysaccharide capsule (Table 24.4).
Table 24.2 The important causative agents of non-viral meningitis, their treatment and prevention
Pathogen | Treatmenta | Prevention |
---|---|---|
Neisseria meningitidis | Penicillin (or chloramphenicol) | Rifampicin prophylaxis for close contacts; polysaccharide vaccine (poor protection against group B) |
Haemophilus influenzae | Ampicillinb, ceftriaxone or cefotaxime (or chloramphenicol) | Polysaccharide vaccine against type b (Hib) |
Streptococcus pneumoniae | Penicillinc (or ceftriaxone or chloramphenicol) | Prompt treatment of otitis media and respiratory infections; polyvalent (23 serotypes) polysaccharide vaccine |
Escherichia coli (and other coliforms), group B streptococci | Gentamicin + cefotaxime or ceftriaxone (or chloramphenicol)b | No vaccines available |
Listeria monocytogenes | Penicillin or ampicillin + gentamicin | No vaccines available |
Mycobacterium tuberculosis | Isoniazid and rifampin and pyrazinamide ± streptomycin | BCG vaccination; isoniazid prophylaxis for contacts recommended in USA |
Cryptococcus neoformans | Amphotericin B and flucytosine | No vaccines available |
a Treatment should be initiated immediately and the susceptibility of the infecting isolate confirmed in the laboratory.
b If isolate is shown to be susceptible (10–20% of isolates are resistant because they produce a plasmid coded beta-lactamase).
c In areas of high prevalence of penicillin resistant pneumococci initial treatment with ceftriaxone may be advised until susceptibility of isolate is known. BCG, bacille Calmette–Guérin.
Meningococcal meningitis
Neisseria meningitidis is carried by about 20% of the population, but higher rates are seen in epidemics
Neisseria meningitidis is a Gram-negative diplococcus which closely resembles N. gonorrhoeae in structure (see Ch. 21), but with an additional polysaccharide capsule that is antigenic and by which the serotype of N. meningitidis can be recognized. The bacteria are carried asymptomatically in the population, up to 20% depending on geographic location, and are attached by their pili to the epithelial cells in the nasopharynx. Invasion of the blood and meninges is a rare and poorly understood event. The known virulence factors are summarized in Table 24.3. People possessing specific complement-dependent bacterial antibodies to capsular antigens are protected against invasion. Those with C5–C9 complement deficiencies show increased susceptibility to bacteraemia (as they do to N. gonorrhoeae bacteraemia; see Ch. 21). Young children who have lost the antibodies acquired from their mother, and adolescents who have not previously encountered the infecting serotype, and therefore have no type-specific immunity, are those most often infected.
Person-to-person spread takes place by droplet infection, and is facilitated by other respiratory infections, often viral, that cause increased respiratory secretions. Thus, conditions of overcrowding and confinement such as prisons, military barracks and college dormitories contribute to the frequency of infection in populations. During outbreaks of meningococcal meningitis, which most frequently occur in late winter and early spring, the carrier rate may reach 60–80%. Specific serotypes associated with infection exhibit some geographic variation. However, serotypes B, C and Y tend to predominate in more resource-rich countries, whereas serotypes A and W-135 are more common in less developed regions. Available vaccines target serotypes A, C, Y and W-135 but not B (Table 24.4). The UK was the first country to introduce the meningitis C conjugate vaccine. It has been part of routine childhood immunization since November 1999. The UK Department of Health recommends that all first-year university and college students and others between 20 and 24 years old should be immunized against meningitis C. The US Centers for Disease Control guidance is similar.
Clinical features of meningococcal meningitis include a haemorrhagic skin rash
After an incubation period of 1–3 days, the onset of meningococcal meningitis is sudden with a sore throat, headache, drowsiness and signs of meningitis which include fever, irritability, neck stiffness and photophobia. There is often a haemorrhagic skin rash with petechiae, reflecting the associated septicaemia (Fig. 24.4). In about 35% of patients, this septicaemia is fulminating, with complications due to disseminated intravascular coagulation, endotoxaemia and shock, and renal failure. In the most severe cases there is an acute Addisonian crisis, with bleeding into the brain and adrenal glands referred to as Waterhouse–Friedrichsen syndrome. Mortality from meningococcal meningitis reaches 100% if untreated, but remains around 10% even if treated. In addition, serious sequelae such as permanent hearing loss may occur in some survivors (Table 24.5).
A diagnosis of acute meningitis is usually suspected on clinical examination
Laboratory identification of the bacterial cause of acute meningitis is essential so that appropriate antibiotic therapy can be given and prophylaxis of contacts initiated. Preliminary microscopy results involving white cell counts and Gram staining for bacteria should be available within an hour of receipt of the CSF sample in the laboratory. Results of culture of CSF and blood should follow after 24 h (see Ch. 32). Molecular diagnosis of meningococcal infection can also be carried out, and may be of clinical assistance as early treatment saves lives, but makes culture of viable organisms from specimens more difficult.
Serology is not helpful in the diagnosis because the infection is too acute for an antibody response to be detectable. Bacterial meningitis is a medical emergency and antibiotic therapy (penicillin or ampicillin) must be instigated if the diagnosis is suspected (Table 24.2).
Type b H. influenzae causes meningitis in infants and young children
• Unencapsulated strains are common and are present in the throat of most healthy people.
• The capsulated type b, a common inhabitant of the respiratory tract of infants and young children (where it may cause infection: see Ch. 18), very occasionally invades the blood and reaches the meninges.
Maternal antibody protects the infant up to 3–4 months of age, but as it wanes, there is a ‘window of susceptibility’ until the child produces his/her own antibody. Anticapsular antibodies are good opsonins (see Ch. 14), which allow the bacteria to be phagocytosed and killed, but children do not generally produce them until 2–3 years of age, possibly because these antibodies are T independent. In addition to the capsule, H. influenzae has several other virulence factors, as shown in Table 24.3.
Acute H. influenzae meningitis is commonly complicated by severe neurologic sequelae
The incubation period of H. influenzae meningitis is 5–6 days, and the onset is often more insidious than that of meningococcal or pneumococcal meningitis (Table 24.5). The condition is less frequently fatal, but, as with meningococcal infection, serious sequelae such as hearing loss, delayed language development, and mental retardation and seizures may occur (Table 24.5).
General diagnostic features are the same as for meningococcal meningitis, as explained above. For laboratory diagnosis, see Chapter 32. It is important to note that the organisms may be difficult to see in Gram-stained smears of CSF, particularly if they are present in small numbers.
H. influenzae type b (Hib) vaccine is effective for children from 2 months of age
General features of treatment are referred to above under meningococcal meningitis; details are summarized in Table 24.2. An effective Hib vaccine, suitable for children 2 months of age and upwards, is available. Rifampicin prophylaxis is recommended for close contacts of patients with invasive Hib disease.
Streptococcus pneumoniae is a common cause of bacterial meningitis, particularly in children and the elderly
Strep. pneumoniae was first isolated more than 100 years ago but relatively little is known about its virulence attributes apart from its polysaccharide capsule (Tables 24.3, 24.4), and the pneumococcus remains a major cause of morbidity and mortality. (Pneumococcal respiratory tract infections are reviewed in Ch. 19.)
The clinical features of pneumococcal meningitis are generally worse than with N. meningitidis and H. influenzae and are summarized in Table 24.5. The general diagnostic features are the same as for meningococcal meningitis described above. Details are referred to in Chapter 32.
Treatment and prevention of pneumococcal meningitis are summarized in Table 24.2. Since penicillin-resistant pneumococci have been observed worldwide, attention must be paid to the antibiotic susceptibility of the infecting strain, and empiric chemotherapy usually involves a combination of vancomycin and either cefotaxime or ceftriaxone.
Listeria monocytogenes meningitis
Listeria monocytogenes causes meningitis in immunocompromised adults
Listeria monocytogenes is a Gram-positive coccobacillus and an important cause of meningitis in immunocompromised adults, especially in renal transplant and cancer patients. It also causes intrauterine infections and infections of the newborn, as summarized in Chapter 23. L. monocytogenes is less susceptible than Strep. pneumoniae to penicillin, and the recommended treatment is a combination of penicillin or ampicillin with gentamicin.
Although mortality rates due to neonatal meningitis in resource-rich countries are declining, the problem is still serious
Neonatal meningitis can be caused by a wide range of bacteria, but the most frequent are group B haemolytic streptococci (GBS) and E. coli (Table 24.6; see also Ch. 23). This may occur by routes such as nosocomial infection. However, the infant may also be infected from the mother. For example, with women vaginally colonized by GBS, the infant may swallow maternal secretions such as infected amniotic fluid during delivery.
Table 24.6 Group B streptococci are a major cause of neonatal meningitis
Group B streptococci (Streptococcus agalactiae) are normal inhabitants of the female genital tract and may be acquired by the neonate | ||
---|---|---|
At or soon after birth | In the nursery | |
Early onset disease | Late onset disease | |
Age | < 7 days | 1 week–3 months |
Risk factors | Heavily colonized mother lacking specific antibody | Lack of maternal antibody |
Premature rupture of membranes | Exposure to cross-infection from heavily colonized babies | |
Pre-term delivery | Poor hygiene in nursery | |
Prolonged labour, obstetric complications | ||
Type of disease | Generalized infection including bacteraemia, pneumonia and meningitis | Predominantly meningitis |
Type of group B streptococcus | All serotypes but meningitis mostly due to type III | 90% type III |
Outcome | Approximately 60% fatal; serious sequelae in many survivors | Approximately 20% fatal |
Treatment | Take blood and CSF for culture | Treat on suspicion |
Treat on suspicion | Take blood and CSF for culture | |
Gentamicin and ampicillin or cefotaxime/ceftriaxone | Gentamicin and ampicillin or cefotaxime/ceftriaxone | |
Prevention | Antibiotic treatment does not reliably abolish carriage in mother; not recommended | Good hygiene practices in nursery |
‘Blind’ treatment of sick baby who has risk factors Future: ?? immunize antibody-negative females of child-bearing age | Do not allow mothers to handle other babies |
CSF, cerebrospinal fluid.
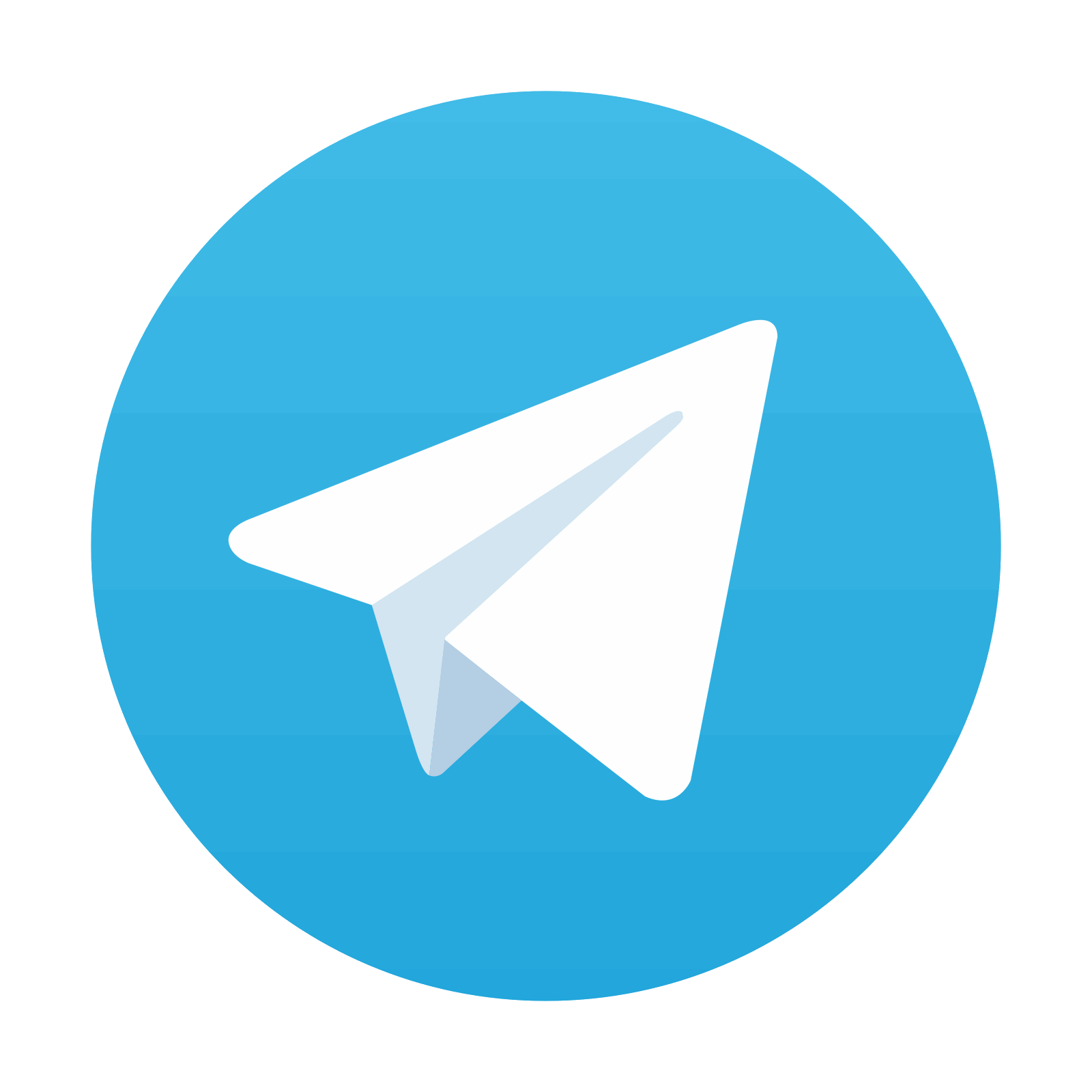
Stay updated, free articles. Join our Telegram channel

Full access? Get Clinical Tree
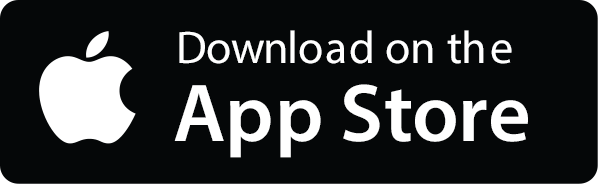
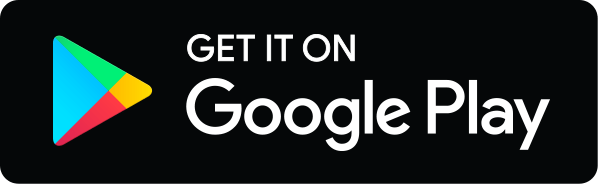