Cell Injury, Aging, and Death
Jacquelyn L. Banasik
Key Questions
• What are the usual cellular responses to reversible injury?
• How are reversible and irreversible cellular injuries differentiated?
• How do necrosis and apoptosis differ?
• To what kind of injuries are cells susceptible?
• What are the usual physiologic changes of aging and how are these differentiated from disease?
Disease and injury are increasingly being understood as cellular and genetic phenomena. Although pathophysiologic processes are often presented in terms of systemic effects and manifestations, ultimately it is the cells that make up the systems that are affected. Even complex multisystem disorders such as cancer ultimately are the result of alterations in cell function. As the mysterious mechanisms of diseases are understood on the cellular and molecular levels, more specific methods of diagnosis, treatment, and prevention can be developed. This chapter presents the general characteristics of cellular injury, adaptation, aging, and death that underlie the discussions of systemic pathophysiologic processes presented in later chapters of this text.
Cells are confronted by many challenges to their integrity and survival and have efficient mechanisms for coping with an altered cellular environment. Cells respond to environmental changes or injury in three general ways: (1) when the change is mild or short-lived, the cell may withstand the assault and completely return to normal. This is called a reversible cell injury. (2) The cell may adapt to a persistent but sublethal injury by changing its structure or function. Generally, adaptation also is reversible. (3) Cell death may occur if the injury is too severe or prolonged. Cell death is irreversible and may occur by two different processes termed necrosis and apoptosis. Necrosis is cell death caused by external injury, whereas apoptosis is triggered by intracellular signaling cascades that result in cell suicide. Necrosis is considered to be a pathologic process associated with significant tissue damage, whereas apoptosis may be a normal physiologic process in some instances and pathologic in others.
Reversible Cell Injury
Regardless of the cause, reversible injuries and the early stages of irreversible injuries often result in cellular swelling and the accumulation of excess substances within the cell. These changes reflect the cell’s inability to perform normal metabolic functions owing to insufficient cellular energy in the form of adenosine triphosphate (ATP) or dysfunction of associated metabolic enzymes. Once the acute stress or injury has been removed, by definition of a reversible injury, the cell returns to its preinjury state.
Hydropic Swelling
Cellular swelling attributable to accumulation of water, or hydropic swelling, is the first manifestation of most forms of reversible cell injury.1 Hydropic swelling results from malfunction of the sodium-potassium (Na+-K+) pumps that normally maintain ionic equilibrium of the cell. Failure of the Na+-K+ pump results in accumulation of sodium ions within the cell, creating an osmotic gradient for water entry. Because Na+-K+ pump function is dependent on the presence of cellular ATP, any injury that results in insufficient energy production also will result in hydropic swelling (Figure 4-1). Hydropic swelling is characterized by a large, pale cytoplasm, dilated endoplasmic reticulum, and swollen mitochondria. With severe hydropic swelling, the endoplasmic reticulum may rupture and form large water-filled vacuoles. Generalized swelling in the cells of a particular organ will cause the organ to increase in size and weight. Organ enlargement is indicated by the suffix -megaly (e.g., splenomegaly denotes an enlarged spleen, hepatomegaly denotes an enlarged liver).
Intracellular Accumulations
Excess accumulations of substances in cells may result in cellular injury because the substances are toxic or provoke an immune response, or merely because they occupy space needed for cellular functions. In some cases, accumulations do not in themselves appear to be injurious but rather are indicators of cell injury. Intracellular accumulations may be categorized as (1) excessive amounts of normal intracellular substances such as fat, (2) accumulation of abnormal substances produced by the cell because of faulty metabolism or synthesis, and (3) accumulation of pigments and particles that the cell is unable to degrade (Figure 4-2).
Normal intracellular substances that tend to accumulate in injured cells include lipids, carbohydrates, glycogen, and proteins. Faulty metabolism of these substances within the cell results in excessive intracellular storage. In some cases, the enzymes required for breaking down a particular substance are absent or abnormal as a result of a genetic defect. In other cases, altered metabolism may be due to excessive intake, toxins, or other disease processes.
A common site of intracellular lipid accumulation is the liver, where many fats are normally stored, metabolized, and synthesized. Fatty liver is often associated with excessive intake of alcohol.2 Mechanisms whereby alcohol causes fatty liver remain unclear, but it is thought to result from direct toxic effects as well as the preferential metabolism of alcohol instead of lipid (see Chapter 38 for a discussion of fatty liver). Lipids may also contribute to atherosclerotic diseases and accumulate in blood vessels, kidney, heart, and other organs. Fat-filled cells tend to compress cellular components to one side and cause the tissue to appear yellowish and greasy (Figure 4-3). In several genetic disorders, the enzymes needed to metabolize lipids are impaired; these include Tay-Sachs disease and Gaucher disease, in which lipids accumulate in neurologic tissue.
Glycosaminoglycans (mucopolysaccharides) are large carbohydrate complexes that normally compose the extracellular matrix of connective tissues. Connective tissue cells secrete most of the glycosaminoglycan into the extracellular space, but a small portion remains inside the cell and is normally degraded by lysosomal enzymes. The mucopolysaccharidoses are a group of genetic diseases in which the enzymatic degradation of these molecules is impaired and they collect within the cell. Mental disabilities and connective tissue disorders are common findings.
Like other disorders of accumulation, excessive glycogen storage can be the result of inborn errors of metabolism, but a common cause is diabetes mellitus.1 Diabetes mellitus is associated with impaired cellular uptake of glucose, which results in high serum and urine glucose levels. Cells of the renal tubules reabsorb the excess filtered glucose and store it intracellularly as glycogen. The renal tubule cells also are a common site for abnormal accumulations of proteins. Normally, very little protein escapes the bloodstream into the urine. However, with certain disorders, renal glomerular capillaries become leaky and allow proteins to pass through them. Renal tubule cells recapture some of the escaped proteins through endocytosis, resulting in abnormal accumulation.
Cellular stress may lead to accumulation and aggregation of denatured proteins. The abnormally folded intracellular proteins may cause serious cell dysfunction and death if they are allowed to persist in the cell. A family of stress proteins (also called chaperone or heat-shock proteins) is responsible for binding and refolding aberrant proteins back into their correct three-dimensional forms (Figure 4-4). If the chaperones are unsuccessful in correcting the defect, the abnormal proteins form complexes with another protein called ubiquitin. Ubiquitin targets the abnormal proteins to enter a proteosome complex, where they are digested into fragments that are less injurious to cells (see Figure 4-4). In some cases, the accumulated substances are not metabolized by normal intracellular enzymes. In diabetes, for instance, high serum glucose levels result in excessive glucose uptake by neuronal cells because they do not require insulin for glucose uptake.3 (Diabetes mellitus is discussed in Chapter 41.)
Finally, a variety of pigments and inorganic particles may be present in cells. Some pigment accumulations are normal, such as the accumulation of melanin in tanned skin, whereas others signify pathophysiologic processes. Pigments may be produced by the body (endogenous) or may be introduced from outside sources (exogenous). In addition to melanin, the iron-containing substances hemosiderin and bilirubin are endogenous pigments that, when present in excessive amounts, indicate disease processes. Hemosiderin and bilirubin are derived from hemoglobin. Excessive amounts may indicate abnormal breakdown of hemoglobin-containing red blood cells (RBCs), prolonged administration of iron, and the presence of hepatobiliary disorders. Inorganic particles that may accumulate include calcium, tar, and mineral dusts such as coal, silica, iron, lead, and silver. Mineral dusts generally are inhaled and accumulate in lung tissue (Figure 4-5). Inhaled dusts cause chronic inflammatory reactions in the lung, which generally result in destruction of pulmonary alveoli and capillaries and the formation of scar tissue. Over many years, the lung may become stiff and difficult to expand because of extensive scarring (see Chapter 23).
Deposits of calcium salts occur in conditions of altered calcium intake, excretion, or metabolism. Impaired renal excretion of phosphate may result in the formation of calcium phosphate salts that are deposited in the tissues of the eye, heart, and blood vessels. Calcification of the heart valves may cause obstruction to blood flow through the heart or interfere with valve closing. Calcification of blood vessels may result in narrowing of vessels and insufficient blood flow to distal tissues. Dead and dying tissues often become calcified (filled with calcium salts) and appear as dense areas on x-ray films. For example, lung damage resulting from tuberculosis often is apparent as calcified areas, called tubercles.
With the exception of inorganic particles, the intracellular accumulations generally are reversible if the causative factors are removed.
Cellular Adaptation
The cellular response to persistent, sublethal stress reflects the cell’s efforts to adapt. Cellular stress may be due to an increased functional demand or a reversible cellular injury. Although the term adaptation implies a change for the better, in some instances an adaptive change may not be beneficial. The common adaptive responses are atrophy (decreased cell size), hypertrophy (increased cell size), hyperplasia (increased cell number), metaplasia (conversion of one cell type to another), and dysplasia (disorderly growth) (Figure 4-6). Each of these changes is potentially reversible when the cellular stress is relieved.
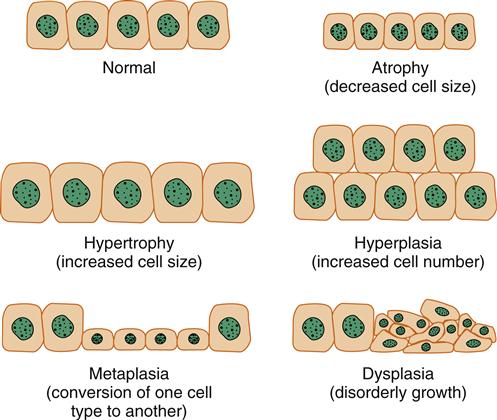
Atrophy
Atrophy occurs when cells shrink and reduce their differentiated functions in response to a variety of normal and injurious factors. The general causes of atrophy may be summarized as (1) disuse, (2) denervation, (3) ischemia, (4) nutrient starvation, (5) interruption of endocrine signals, (6) and persistent cell injury. Apparently, atrophy represents an effort by the cell to minimize its energy and nutrient consumption by decreasing the number of intracellular organelles and other structures.
A common form of atrophy is the result of a reduction in functional demand, sometimes called disuse atrophy. For example, immobilization by bed rest or casting of an extremity results in shrinkage of skeletal muscle cells. On resumption of activity, the tissue resumes its normal size. Denervation of skeletal muscle results in a similar decrease in muscle size caused by loss of nervous stimulation. Inadequate blood supply to a tissue is known as ischemia. If the blood supply is totally interrupted, the cells will die, but chronic sublethal ischemia usually results in cell atrophy. The heart, brain, kidneys, and lower leg are common sites of ischemia. Atrophic changes in the lower leg attributable to ischemia include thin skin, muscle wasting, and hair loss. Atrophy also is a consequence of chronic nutrient starvation, whether the result of poor intake, absorption, or distribution to the tissues. Many glandular tissues throughout the body depend on growth-stimulating (trophic) signals to maintain size and function. For example, the adrenal cortex, thyroid, and gonads are maintained by trophic hormones from the pituitary gland and will atrophy in their absence. Atrophy that results from persistent cell injury is most commonly related to chronic inflammation and infection.
The biochemical pathways that result in cellular atrophy are imperfectly known; however, two pathways for protein degradation have been implicated. The first is the previously mentioned ubiquitin-proteosome system, which degrades targeted proteins into small fragments (see Figure 4-4). The second involves the lysosomes that may fuse with intracellular structures leading to hydrolytic degradation of the components. Certain substances apparently are resistant to degradation and remain in the lysosomal vesicles of atrophied cells. For example, lipofuscin is an age-related pigment that accumulates in residual vesicles in atrophied cells, giving them a yellow-brown appearance.
Hypertrophy
Hypertrophy is an increase in cell mass accompanied by an augmented functional capacity. Cells hypertrophy in response to increased physiologic or pathophysiologic demands. Cellular enlargement results primarily from a net increase in cellular protein content.4 Like the other adaptive responses, hypertrophy subsides when the increased demand is removed; however, the cell may not entirely return to normal because of persistent changes in connective tissue structures. Organ enlargement may be a result of both an increase in cell size (hypertrophy) and an increase in cell number (hyperplasia). For example, an increase in skeletal muscle mass and strength in response to repeated exercise is primarily the result of hypertrophy of individual muscle cells, although some increase in cell number is also possible because muscle stem cells (satellite cells) are able to divide. Physiologic hypertrophy occurs in response to a variety of trophic hormones in sex organs—the breast and uterus, for example. Certain pathophysiologic conditions may place undue stress on some tissues, causing them to hypertrophy. Liver enlargement in response to bodily toxins and cardiac muscle enlargement in response to high blood pressure (Figure 4-7) represent hyperplastic and hypertrophic adaptations to pathologic conditions. Hypertrophic adaptation is particularly important for cells, such as differentiated muscle cells, that are unable to undergo mitotic division.
Hyperplasia
Cells that are capable of mitotic division generally increase their functional capacity by increasing the number of cells (hyperplasia) as well as by hypertrophy. Hyperplasia usually results from increased physiologic demands or hormonal stimulation. Persistent cell injury also may lead to hyperplasia. Examples of demand-induced hyperplasia include an increase in RBC number in response to high altitude and liver enlargement in response to drug detoxification. Trophic hormones induce hyperplasia in their target tissues. Estrogen, for example, leads to an increase in the number of endometrial and uterine stromal cells. Dysregulation of hormones or growth factors can result in pathologic hyperplasia, such as that which occurs in thyroid or prostate enlargement.
Chronic irritation of epithelial cells often results in hyperplasia. Calluses and corns, for example, result from chronic frictional injury to the skin. The epithelium of the bladder commonly becomes hyperplastic in response to the chronic inflammation of cystitis.
Metaplasia
Metaplasia is the replacement of one differentiated cell type with another. This most often occurs as an adaptation to persistent injury, with the replacement cell type better able to tolerate the injurious stimulation.1 Metaplasia is fully reversible when the injurious stimulus is removed. Metaplasia often involves the replacement of glandular epithelium with squamous epithelium. Chronic irritation of the bronchial mucosa by cigarette smoke, for example, leads to the conversion of ciliated columnar epithelium to stratified squamous epithelium. Metaplastic cells generally remain well differentiated and of the same tissue type, although cancerous transformations can occur. Some cancers of the lung, cervix, stomach, and bladder appear to derive from areas of metaplastic epithelium.
Dysplasia
Dysplasia refers to the disorganized appearance of cells because of abnormal variations in size, shape, and arrangement. Dysplasia occurs most frequently in hyperplastic squamous epithelium, but it may also be seen in the mucosa of the intestine. Dysplasia probably represents an adaptive effort gone astray. Dysplastic cells have significant potential to transform into cancerous cells and are usually regarded as preneoplastic lesions. (See Chapter 7 for a discussion of cancer.) Dysplasia that is severe and involves the entire thickness of the epithelium is called carcinoma in situ. Mild forms of dysplasia may be reversible if the inciting cause is removed.
Irreversible Cell Injury
Pathologic cellular death occurs when an injury is too severe or prolonged to allow cellular adaptation or repair. Two different processes may contribute to cell death in response to injury: necrosis and apoptosis. Necrosis usually occurs as a consequence of ischemia or toxic injury and is characterized by cell rupture, spilling of contents into the extracellular fluid, and inflammation. Apoptosis (from a Greek word meaning falling off, as in leaves from a tree) occurs in response to injury that does not directly kill the cell but triggers intracellular cascades that activate a cellular suicide response. Apoptotic cells generally do not rupture and are ingested by neighboring cells with minimal disruption of the tissue and without inflammation. Apoptosis is not always a pathologic process and occurs as a necessity of development and tissue remodeling.
Necrosis
Necrotic cells demonstrate typical morphologic changes, including a shrunken (pyknotic) nucleus that is subsequently degraded (karyolysis), a swollen cell volume, dispersed ribosomes, and disrupted plasma and organelle membranes (Figure 4-8). The disruption of the permeability barrier of the plasma membrane appears to be a critical event in the death of the cell.5
Localized injury or death of tissue is generally reflected in the entire system as the body attempts to remove dead cells and works to compensate for loss of tissue function. Several manifestations indicate that the system is responding to cellular injury and death. A general inflammatory response is often present, with general malaise, fever, increased heart rate, increased white blood cell (WBC) count, and loss of appetite. With the death of necrotic cells, intracellular contents are released and often find their way into the bloodstream. The presence of specific cellular enzymes in the blood is used as an indicator of the location and extent of cellular death. For example, an elevated serum amylase level indicates pancreatic damage, and an elevated creatine kinase (MB isoenzyme) or cardiac troponin level indicates myocardial damage. The location of pain caused by tissue destruction may also aid in the diagnosis of cellular death.
Four different types of tissue necrosis have been described: coagulative, liquefactive, fat, and caseous (Figure 4-9). They differ primarily in the type of tissue affected. Coagulative necrosis is the most common. Manifestations of coagulative necrosis are the same, regardless of the cause of cell death. In general, the steps leading to coagulative necrosis may be summarized as follows: (1) ischemic cellular injury, leading to (2) loss of the plasma membrane’s ability to maintain electrochemical gradients, which results in (3) an influx of calcium ions and mitochondrial dysfunction, and (4) degradation of plasma membranes and nuclear structures (Figure 4-10). The area of coagulative necrosis is composed of denatured proteins and is relatively solid. The coagulated area is then slowly dissolved by proteolytic enzymes and the general tissue architecture is preserved for a relatively long time (weeks). This is in contrast to liquefactive necrosis.
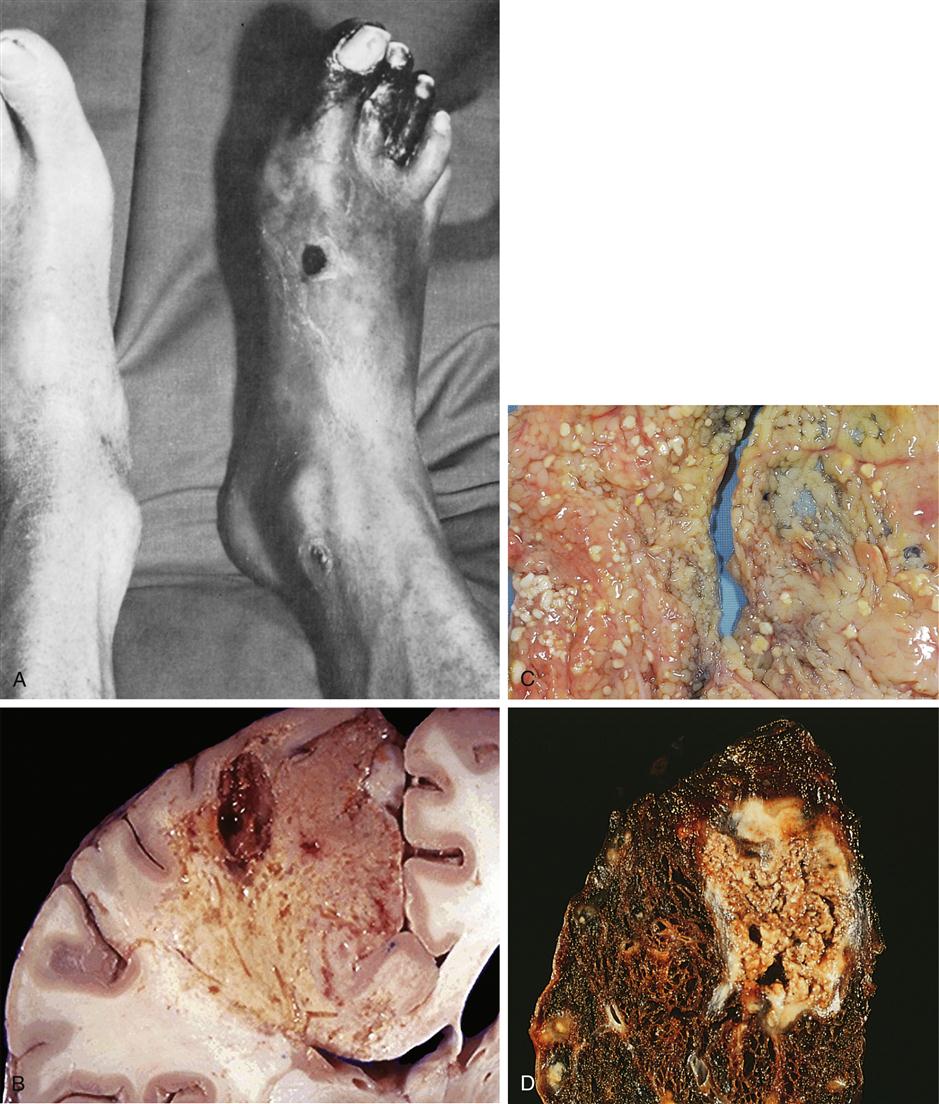
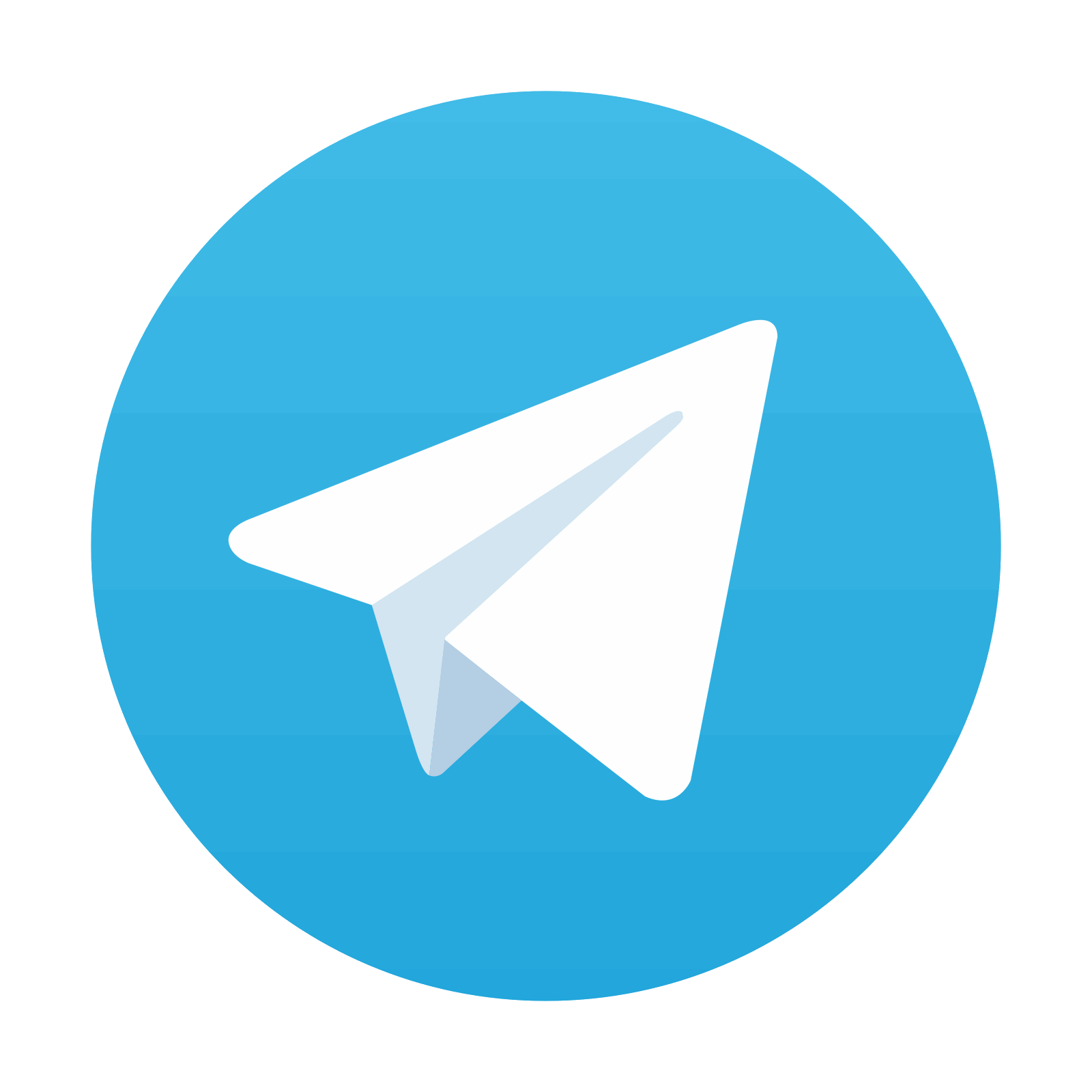
Stay updated, free articles. Join our Telegram channel

Full access? Get Clinical Tree
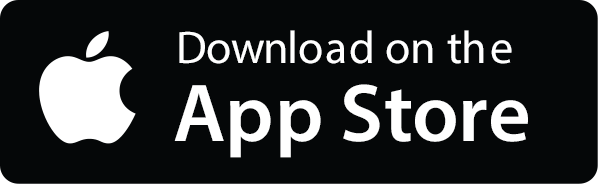
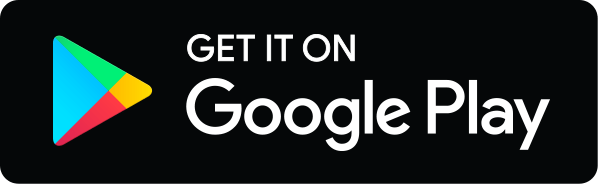