OBJECTIVES
1 List the typical hemodynamic measurements (includng central venous pressure, pulmonary arterial pressure, pulmonary artery wedge pressure, and cardiac output) in a normal patient and a patient with hypovolemic shock, cardiac tamponade, or acute myocardial infarction
2 Discuss the advantages of, indications for, and possible complications of a central venous line versus a Swan-Ganz catheter
3 Discuss the unique contributions of extracorporea circulation and myocardial preservation techniques to cardiac surgery.
4 List the risk factors and common clinical symptoms in a patient with ischemic heart disease, and discuss the differential diagnosis
5 List treatment options for patients with ischemic heart disease
6 Describe the indications for surgical intervention in a patient with ischemic heart disease, and evaluate the variety of techniques available, including minimally invasive approaches
7 Explain the physiologic benefits of intra-aortic balloon pump counter pulsation, as well as its complications and contraindications
8 Describe the appropriate management of acute cardiac tamponade
9 Describe the complications of a myocardial infarction
10 State the clinical indications for aortic, tricuspid, and mitral valve replacement
11 List the American Heart Association indications for prophylactic antibiotics in patients with heart disease.
12 List the indications for pacemaker insertion
13 Discuss the common congenital cardiac abnormalities, including patent ductus arteriosus, tetralogy of Fallot, coarctation of the aorta, septal defects, and transposition of the great vessels, and stratify these abnormalities according to cyanotic or acyanotic presentation
14 Describe the evaluation and treatment of traumatic rupture of the aorta, and discuss the complications of treatment
15 Describe the evaluation and treatment of aortic dissection
16 Describe the clinical manifestation, anatomy, and treatment of thoracic aortic aneurysms
17 Discuss the diagnostic modalities available to investigate chest lesions (e.g., pulmonary mass, chest wall tumor, pleural disease)
18 Discuss the evaluation and differential diagnosis of hemoptysis
19 Create an algorithm to evaluate a solitary lung nodule
20 Describe the common causes of pleural effusion, and discuss the distinctions between exudate and transudate
21 Discuss the etiology and management of lung abscess and empyema
22 Define and discuss the management of various types of chest trauma, including open pneumothorax, tension pneumothorax, hemothorax, and flail chest.
23 Discuss the workup and management of patients with chest wall and mediastinal pathology.
24 Discuss the preoperative evaluation of patients for thoracic surgery.
25 Describe the common pathologic lesions of the anterior, posterior, and superior portions of the mediastinum
26 Discuss the risk factors and symptoms of lung cancer.
27 Discuss the management of primary lung neoplasms
28 Describe the preoperative preparation and assessment of patients who undergo pulmonary resection
29 List the most common sources of metastatic lesions in the lung, and discuss the management of metastatic disease in the chest
This chapter is divided into sections on the heart, the thoracic aorta and great vessels, and the thoracic cavity, which includes the chest wall, mediastinum, and lungs. Diseases of the esophagus that are treated by thoracic and general surgeons are covered in Chapter 13 of Lawrence et al, Essentials of General Surgery, 4th ed.
DISEASES OF THE HEART
This section discusses cardiac structure and function and demonstrates the surgeon’s approach to ischemic heart disease, valvular heart disease, congenital heart disease, and disorders of the pericardium. Cardiovascular diseases are the most common causes of significant morbidity and mortality in all sectors of the population. Enormous resources are consumed in treating these diseases. Heart disease is of major concern to patients, physicians, and policy makers alike. According to the American Heart Association, in 2002 coronary artery disease, myocardial infarction, angina, and congestive heart failure affected 13,000,000, 7,100,000, 6,400,000, and 4,900,000 people, respectively, in the United States alone. Approximately 927,000 deaths occurred related to cardiovascular diseases. Congenital heart disease ranks as the second leading cause of death (following accidents) in children 15 years of age or less. Almost 210,000 cardiovascular procedures were performed on this younger age group. More than $383 billion were spent on treatment and prevention of cardiovascular diseases affecting all segments of the population.
Anatomy
The heart is a hollow, muscular organ that provides the physical force necessary to deliver oxygen-rich blood to the body and to return oxygen-poor blood to the lungs. The anatomy of the normal heart is well suited for this task, because blood destined for the lungs is kept separate from blood destined for the periphery. The atria receive blood from the peripheral or central circulation. They allow this blood to pass through one-way atrioventricular valves (tricuspid on the right; mitral on the left) into the primary pumping chambers, or ventricles. The left ventricle, which delivers blood against systemic vascular resistance, is more muscular than the right ventricle, which delivers blood to the lower resistance pulmonary circuit. The heart is an end-organ that receives its circulation from the epicardial coronary arteries that arise from the left and right sinuses of Valsalva, distal to the aortic valve (Fig. 4-1). The left main coronary artery branches into the left anterior descending coronary artery and the circumflex coronary artery. Each of these in turn may give off additional branches. The right coronary artery usually supplies the posterior descending coronary artery distally and a posterolateral branch. The blood supply to the sinus and atrioventricular nodes usually arises from the right coronary artery and often explains the occurrence of heart block in the presence of a right coronary occlusion or infarct.
As an end organ, the heart is affected by the same physiologic disturbances that affect peripheral organs. The ability of the heart to provide blood to the periphery may be adversely affected by any alteration in the normal anatomy of the heart (e.g., defects of the atrial or ventricular septum, valvular incompetence or stenosis, coronary artery obstruction).
Figure 4-1 Diagram of heart and coronary arteries.
Physiology: Cardiac Function and Its Assessment
Physiologic manipulation of the circulation is essential for treating patients with cardiovascular disease (and therefore is covered here as well in the Critical Care chapter in Lawrence et al, Essential of General Surgery, 4th ed). It should always be recognized that cardiac function can be clinically assessed with simple parameters (blood pressure, urine output, skin color and texture, mental status, and heart rate). Unfortunately, these measurements do not always reflect changes in cardiac function until deterioration has occurred, sometimes irreversibly. Earlier detection of myocardial dysfunction is possible with both noninvasive and invasive techniques that permit pharmacologic and mechanical intervention to halt deterioration.
A catheter can be placed via a central vein so that its tip lies within the thoracic cavity to monitor central venous pressure (CVP). The limitation of the CVP is that it does not directly measure the function of the left side of the heart. It measures the ability of the right side of the heart to deal with the volume load delivered by systemic veins and can be useful in detecting compromised left ventricular function (in patients who do not otherwise have heart disease). When there is intrinsic cardiac disease, the response of the left side of the heart will likely differ from that of the right side. Further, any clinical derangement that affects both the systemic volume and, indirectly, pulmonary vascular resistance may alter CVP without actually altering cardiac function. A single CVP measurement will probably overassess or underassess the dysfunction. A record of changes in CVP over time is more useful than an isolated measurement.
A flow-directed pulmonary artery catheter may be inserted into a large central vein and “floated” through the right heart and out into the pulmonary artery (Figs. 4-2 and 4-3). This is usually done with a Swan-Ganz catheter, which can be used to measure CVP, pulmonary artery pressure, pulmonary capillary wedge pressure, and cardiac output. When the balloon on the end of the catheter is inflated, the catheter can be wedged into a small pulmonary artery. The pressure measured in this position is called the pulmonary capillary wedge pressure (PCWP). By the laws of hydraulics, this will reflect left atrial pressure, which, in the absence of mitral valve disease, reflects pressure in the left ventricle at the end of diastole when the mitral valve is open (left ventricular end-diastolic pressure [LVEDP]). In turn, this pressure reflects the left ventricular end-diastolic volume component of cardiac output. According to Starling’s law, left ventricular muscle contractile force is proportional to myocardial fiber stretch: the greater the volume of blood within the ventricle at the end of diastole, the greater is the force of contraction and therefore of cardiac output. Because rapid and accurate bedside methods to measure end-diastolic volume are not readily available, measurements of LVEDP are used instead. LVEDP is used as a guide to the volume status, or filling pressure, of the heart. The translation of left ventricular end-diastolic volume into a clinically useful pressure measurement (LVEDP) assumes that compliance of the ventricle remains constant. However, that is not always the case. Certain clinical conditions (e.g., left ventricular hypertrophy, acute myocardial infarction) that acutely alter left ventricular compliance can change the relation between left ventricular end-diastolic volume and pressure.
Figure 4-2 A flow-directed pulmonary artery catheter in place
Figure 4-3 Tracings from a flow-directed pulmonary artery catheter. RA, right atrium; RV, right ventricle; PA, pulmonary artery; PCW, pulmonary capillary wedge.
Cardiac output can be measured using the Swan-Ganz catheter by means of thermodilution techniques that use a thermistor at the tip of the catheter. This calculation is combined with blood pressure, CVP, and PCWP to calculate resistance and assess cardiac function. Adding a fiberoptic system that can provide realtime, online measurement of oxygen saturation in the pulmonary artery allows further discrimination in such assessment. The mixed venous oxygen saturation (SVO2) reflects cardiac index and systemic oxygen delivery as long as hemoglobin levels and oxygen extraction (metabolic activity and work) are accounted for. A decrease in SVO2 can be caused by a decrease in cardiac output, a decrease in hemoglobin levels, or an increase in the amount of oxygen extracted at the cellular level. Careful assessment is necessary to decide which variable is operational in any given patient. The mixed venous oxygen saturation, although affected by hemoglobin concentration, is a clinically useful tool for moment-to-moment assessment of cardiac function. Table 4-1 shows the basic formulas used to assess myocardial function based on measurements available in an intensive care unit.
When these types of invasive monitoring are available, it is easy to distinguish the etiology of hypotension and shock. A patient who is in hypovolemic shock may be diagnosed by clinical signs (trauma, hemorrhage, vomiting, diarrhea, dehydration) and simple bedside measurements (assessment of mucous membranes, skin turgor, urine output, blood pressure, pulse rate). However, when clinical indicators are equivocal, invasive measurements that show low pulmonary artery wedge pressure (PAWP), CVP, and cardiac index usually confirm a low circulating volume. Appropriate therapy is transfusion with blood products or balanced electrolyte solutions. Both clinical and invasive measurements are used to gauge patient response.
Usually, patients who have heart failure because of chronic conditions (e.g., valvular heart disease) or acute problems (e.g., acute myocardial infarction) have elevated PAWP because of inadequate myocardial pump function and diminished cardiac index. However, the caution about the integration of myocardial compliance, wedge pressure, and cardiac index is often forgotten in the setting of acute cardiac dysfunction. For example, a patient who has an acute myocardial infarction, a cardiac index of 1.8 L/min/ m2, and a wedge pressure of 6 mm Hg is hypovolemic. The patient’s edematous infarcted myocardium may require filling pressures greater than the “normal” wedge pressure of 12 to 15 mm Hg to stretch the myocardium sufficiently to generate adequate cardiac output. Conversely, another patient who has an acute myocardial infarction may have a cardiac index of 1.8 L/min/m2, but a wedge pressure of 25 mm Hg. This patient is clearly in pulmonary edema and will benefit from a reduction in the filling pressures of the left ventricle to allow improved coronary perfusion of the critical areas of subendocardial myocardial muscle mass. According to Laplace’s law, wall tension is directly proportional to the radius of the heart chamber and the pressure within it. Therefore, a dilated left ventricle will have subendocardial perfusion deficits based on transmyocardial pressure gradients within the coronary circulation (especially during diastole, when most coronary perfusion occurs). Reducing the radius of the left ventricle with pulmonary vasodilators (e.g., morphine, nitroglycerin), diuretics (e.g., furosemide), or phlebotomy (actual or rotating tourniquets) will decrease wall tension and increase subendocardial perfusion and therefore improve overall cardiac function. In the presence of hypertension, afterload reduction is likewise helpful. Continued low cardiac output may require the use of inotropes, intra-aortic balloon, or emergency invasive intervention.
Vasodilatory shock can be associated with early sepsis or other causes leading to lowering of systemic resistance. In this situation, hypotension may in fact be associated with a high cardiac output and normal or elevated filling pressures. Vasoconstrictive agents are indicated in this situation to maintain adequate pressure to perfuse the end organs.
The shock associated with cardiac tamponade can be very difficult to assess and can lead to a delay in treatment that may be fatal. In this situation, low blood pressure is associated with a low cardiac output, elevated filling pressure and, usually, elevated systemic resistance. One of the hallmarks of tamponade is “equalization of pressures,” that is, filling pressures on both sides of the heart tend to equalize, systemic pressure drops, pulmonary pressure rises, and pulse pressure may narrow. Treatment requires immediate relief of the tamponade by mechanical interventions such as pericardiocentesis or surgical drainage of the pericardium.
Although invasive monitoring devices provide valuable information, they can cause complications. Depending on the site of insertion, any central venous cannulation can cause hemorrhage and pneumothorax. In a critically ill patient whose condition is acutely deteriorating, proper insertion techniques must be used. The safest approach to introducing a pulmonary artery flotation catheter is a posterior-superior approach to the right internal jugular vein. In this approach, the introducer needle is never in contact with the lung. Lower jugular or subclavian approaches are more likely to cause pneumothorax. They are used especially cautiously in patients with blood dyscrasias and clotting abnormalities, because direct pressure cannot be applied to the subclavian vein or the internal jugular vein beneath the clavicle. In these patients, a high cervical approach to the central veins or the temporary use of the femoral vein is recommended. Perforation of the pulmonary artery with massive hemoptysis may occur after insertion of a pulmonary artery catheter. In addition, cardiac arrhythmias can occur when the catheter is passed through the right ventricle. Infectious endocarditis is also a potential problem, especially when the catheter is in place for more than 3 days.
Additional noninvasive means of assessing cardiac function include radionuclide scanning, echocardiography, and cardiac magnetic resonance imaging (MRI). Multiple gated acquisition (MUGA) scans show ejection fractions quite accurately and therefore can categorize cardiac function (Fig. 4-4), but they are not easily performed at the bedside. Echocardiography and transesophageal echocardiography can be performed at the bedside, but must be interpreted by an experienced echocardiographer. Cardiac MRI is a newer method of cardiac evaluation and can be very accurate in the assessment of cardiac function, but again is not available at the bedside. During surgical procedures, a transesophageal echocardiogram (TEE) can be used to continuously monitor cardiac volume, function, and wall motion.
Figure 4-4 Radionuclide angiocardiography. Frames representing end-diastole (ED) and end-systole (ES). An automatic edge detection algorithm was applied for definition of the edges. Left atrium (LA) is correctly excluded from the left ventricular (LV) region of interest. Background region (arrows) is in standardized position and covers representative background area (BKG). Here the camera is positioned in left anterior oblique 45-degree position. RV, right ventricle. (Reprinted with permission from Images. MD, The Online Encyclopedia of Medical Images. Taken from Mannting F. Atlas of Cardiac Imaging. Edited by Richard Lee, Richard T. Lee, Eugene Braunwald. Current Medicine LLC: 1998.)
Cardiopulmonary Bypass and Myocardial Protection
The ability to perform surgery on the heart and great vessels awaited the development of a method to artificially support the circulation and respiration while, at the same time, preventing significant damage to the heart. In the 1930s, Gibbon began work on a technique of circulatory support to allow pulmonary embolectomy; a procedure that he believed was inadequately dealt with by the closed techniques of Trendelenburg. It was not until the early 1950s that cooperation among Gibbon, the IBM Corporation, the University of Minnesota, and the Mayo Clinic produced the first clinically successful pump oxygenators. The heart–lung machine was used successfully for the first time in 1953 to repair a secundum atrial septal defect in a young girl. Numerous failures occurred before this case. The mortality rate for early open-heart surgery approached 40% to 50%. Over time, biomechanical improvements resulted in safer cardiopulmonary bypass.
There are multiple components to the heart-lung machine (Fig. 4-5). All of the “foreign” surfaces involved are thrombogenic and require the use of systemic heparinization in order to prevent thrombosis. Today, the roller pump and the vortex pump are the most commonly used devices to provide for the actual circulation of blood. Membrane oxygenators of various designs provide for gas exchange and take over the function of the lungs. Temperature regulation is maintained by a heater–cooler, and scavenging of blood from the operative field is done by suckers that return heparinized blood to the machine. Filtering devices are present, as are sensors and traps to prevent air embolization during the procedure. Blood is withdrawn from the venous circulation, either through a single cannula in the right atrium or through separate cannulae in the inferior and superior vena cavae. The blood passes through the oxygenator, where oxygen levels are replenished and carbon dioxide is removed. With the appropriate pump mechanism, blood is reintroduced into the circulation through either the ascending aorta or the femoral artery. Mild, moderate, or deep hypothermia is often employed because metabolic demands are diminished at lower temperatures. The responses of the body to this insult are manifest in many ways and are frequently characterized by a total body inflammatory response that can lead to or be associated with many of the complications of using the heart–lung machine, including organ failure and coagulopathy.
Figure 4-5 Diagram of a typical cardiopulmonary bypass circuit with vent, field suction, aortic root suction, and cardioplegic system. Blood is drained from a single “two-stage” catheter into the venous reservoir, which is part of the membrane oxygenator/heat exchanger unit. Venous blood exits the unit and is pumped through the heat exchanger and then the oxygenator. Arterialized blood exits the oxygenator and passes through a filter/bubble trap to the aortic cannula, which is usually placed in the ascending aorta. Blood aspirated from vents and suction systems enters a separate cardiotomy reservoir, which contains a microfilter, before entering the venous reservoir. The cardioplegic system is fed by a spur from the arterial line to which the cardioplegic solution is added and is pumped through a separate heat exchanger into the antegrade or retrograde catheters. Oxygenator gases and water for the heat exchanger are supplied by independent sources. (Reprinted with permission from Cohn LH, Edmunds LH Jr, eds. Cardiac Surgery in the Adult. New York: McGraw-Hill, 2003. Available at: http://cardiacsurgery.ctsnetbooks.org/cgi/content/full/2/2003/317_Pumps.)
Myocardial Protection
The goal of myocardial protection is to provide a quiet, bloodless field, the optimal environment for working on or in the heart, without causing damage to the heart muscle. To achieve this, cardiac activity must be stopped, the coronary circulation occluded, and protection provided to the myocardial cells. The cells are protected by minimizing the metabolic demand of the heart while it is deprived of its normal circulation and oxygen supply. Recalling the determinants of oxygen demand (wall tension, heart rate, and contractility), this is achieved by emptying the heart, stopping the heart, and eliminating any work it must do (taking over its pumping function). A major improvement in the survival of patients undergoing cardiac surgery came with the introduction of potassium cardioplegia in the late 1970s. Since its inception, the principles of cardioplegic arrest have been adopted as the standard method of myocardial protection. The heart is stopped after initiating cardiopulmonary bypass by applying a cross-clamp to the aorta and delivering potassium cardioplegia into the aortic root and down the coronary arteries. The high potassium content (20–25 mEq/L) of the solution eliminates the concentration gradient of potassium across the myocardial cell membranes and induces electrochemical paralysis of myocardial cellular function. The composition of the cardioplegic solution varies among institutions, but it usually consists of a crystalloid or a blood carrier, a buffer to antagonize the ischemic acidosis, and a substance (e.g., mannitol) to render the solution hypertonic to the myocardial cell to minimize edema. Various modifications of this solution are used for cardioplegia induction and reperfusion. To overcome the heterogeneous distribution of antegrade cardioplegia because of coronary artery stenoses, some surgeons will instill cardioplegic solution in a retrograde fashion through the coronary sinus. The coronary venous system has no valves and is not subject to atherosclerotic obstruction, allowing homogeneous distribution of the cardioplegia. Periods of ischemic cardioplegic arrest lasting 1 to 3 hours are well tolerated. Cardiac activity resumes after normal perfusion is restored. Any damage that occurs during reperfusion after ischemic arrest can be aggravated by various electrochemical elements (e.g., oxygen free radicals). Free-radical scavengers can improve cardiac performance after ischemic arrest.
Ischemic Heart Disease
Pathophysiology
The most commonly recognized cause of ischemic heart disease is the occlusion of large epicardial vessels in the heart by atherosclerotic cardiovascular disease. Other etiologies include valvular heart disease, vasculitis, congenital coronary anomalies, and dissection of the thoracic aorta if the ostia of the coronary arteries are involved. In all of these cases, the underlying pathogenesis is the mismatch of myocardial oxygen supply and demand. This mismatch is most commonly caused by the obstruction of flow through the coronary bed by a narrowing of more than 50% of the cross-sectional diameter of the vessel (Fig. 4-6). The usual manifestation of ischemia is angina pectoris characterized by substernal chest pain or pressure that may radiate down the arm. Other “anginal equivalents,” however, may be present (e.g., jaw pain, throat pain, arm pain, dyspnea on exertion). Diabetics in particular are likely to manifest dyspnea on exertion as their anginal equivalent because of decreased neural sensitivity from the heart.
Figure 4-6 Myocardial perfusion imaging. Myocardial perfusion scan from a 61-yearold woman presenting with recurrent typical exertional chest pain (CP). Test stopped due to typical CD; electrocardiography shows 1-mm ST depression. Findings: No rest perfusion abnormalities. During stress, moderate severe ischemia in the lateral wall from apex to base (6 of 25 segments, typical left circumflex coronary artery [LCx] territory). Subsequent coronary angiogram: LAD 40% stenosis, LCx totally occluded distally, obtuse marginal branch 95% stenosis, right coronary artery small and totally occluded. Thus, LCx and RCA are both occluded. Both the lateral and the inferior still show normal perfusion at rest. This appearance can be explained by sufficient collateral flow at rest, but insufficiency of the collateral flow during stress first manifested in the lateral wall. (Reprinted with permission from Images. MD, The Online Encyclopedia of Medical Images. Taken from Mannting F. Atlas of Cardiac Imaging. Edited by Richard Lee, Richard T. Lee, Eugene Braunwald. Current Medicine LLC: 1998.)
The physiology of oxygen supply to the heart is unique compared with that of other end organs. Although other organs are perfused mainly during systole, the heart receives most (approximately 80%) of its blood flow during diastole, when intracavitary pressure of the heart and the resulting transmural pressure gradient are low. The heart extracts 70% or more of the oxygen carried by capillary blood before the blood is returned to the venous system. Consequently, the heart cannot meet increased metabolic demands by increasing the extraction of oxygen from the blood, as most other organs do. Instead, it must rely on increased blood flow to the myocardium to meet these needs.
The supply of oxygen to the heart is determined by the duration of the diastolic interval (which decreases as heart rate increases or obstruction to left ventricular outflow occurs); the oxygen-carrying capacity of the blood (hemoglobin level and oxygen saturation); and the unimpeded flow of blood through the coronary arteries. Stenosis that narrows the cross-sectional area of a large epicardial coronary artery by more than 75% (50% cross-sectional diameter) impedes the required increase in blood flow to the bed supplied by that coronary artery when metabolic demand increases.
Demand of the heart for oxygen has three major determinants: (a) wall tension as measured by Laplace’s law (tension = [pressure × radius]/2 wall thickness); (b) heart rate; and (c) the level of contractility of the heart (Starling’s law). Laplace’s law summarizes the effects of preload (radius of the chamber and pressure in the chamber during diastole), afterload (pressure during systole), and wall thickness (compensatory changes to chronic increases in afterload).
The etiology of atherosclerotic cardiovascular disease is multifactorial. It is a progressive disease that can appear microscopically, even in infants. Thelesions develop over time as a complex interaction of lipid deposition within the intima of the vessel wall, along with blood and blood products, fibrous tissue, and calcification. After significant stenoses occur, symptoms may ensue. In addition, dynamic plaques (intermittent closure), rupture of plaques with hemorrhage into the wall, or acute thrombosis superimposed on these plaques can contribute to episodes of ischemia or to the development of myocardial infarction, even if oxygen demand on the heart is not increased. More recently, factors associated with inflammation and the inflammatory response have been implicated in these changes.
Many factors are recognized as contributing to the development of atherosclerotic cardiovascular disease. These include genetic factors, hypertension, diabetes, obesity, hypercholesterolemia, stress, inactivity, and smoking. Many of these factors can be modified to alter the progressive course of the disease.
Clinical Presentation and Evaluation
The diagnosis of ischemic heart disease may be complex and depends on the presentation of the patient. Some patients come to the hospital with severe unstable angina or acute myocardial infarction that requires rapid diagnosis and treatment.
History
Patients may present emergently with acute onset of severe chest pressure or pain (often radiating down the arm), diaphoresis, nausea, and vomiting. Some patients have heart failure manifested by pulmonary edema or hypotension. Such patients are likely to be evolving an acute myocardial infarction or having severe unstable angina. These patients require hospitalization for further emergency diagnosis and treatment.
Other patients have more chronic forms of ischemic heart disease. They often have angina, especially associated with physical activity. A complete, careful history and physical examination often raise the suspicion of ischemic heart disease. The history should focus on defining the nature of the discomfort and identifying factors that bring on the symptoms (e.g., exercise, sexual intercourse, emotional stress) or other factors that significantly increase myocardial oxygen demand. Angina must be differentiated from other causes of chest pain, such as the epigastric discomfort of heartburn, the chest pain of pericarditis or pleuritis, or the discomfort of bursitis or inflammatory problems in the chest wall. Additional inquiries should be made regarding family history and personal history of myocardial infarction, heart murmur, hypertension, diabetes, or connective tissue disorders. Questions should be asked about smoking, exercise, dietary habits, and use of medication.
The examiner should attempt to elicit signs of coronary artery disease or other diseases that may be associated with atherosclerotic disease of the heart. Peripheral signs of atherosclerosis include carotid bruits, abdominal aortic aneurysms, loss of peripheral pulses, and symptoms of leg ischemia and cerebral ischemia. Signs of heart failure include rales, peripheral edema, and hepatic enlargement. On cardiac examination, the patient may show signs of increased cardiac size and abnormal heart sounds that suggest heart failure. The presence of any cardiac murmurs should prompt a search for valvularlesions, usually by echocardiography.
Diagnostic Evaluation
In the emergency setting, acute changes are often noted on electrocardiogram (ECG), especially when there is ongoing pain. These changes may include Q waves that indicate myocardial cellular necrosis because of the inability of dead cells to become electrically excitable; ST segment elevations caused by the inability of the affected myocyte to repolarize normally; and T wave inversions and QT prolongations that reflect ongoing cardiac ischemia because of delayed repolarization. Creatine phosphokinase (CPK) isoenzymes and troponin levels are elevated when myocardial cellular damage has occurred.
In the more chronic situation, if the index of suspicion for ischemic heart disease is high, further diagnostic studies are often needed. The usual process is to proceed to stress testing. Stress is induced to increase coronary blood flow. This can be accomplished by physical exercise, increasing the heart rate by pacing, or coronary vasodilatation by dipyridamole or adenosine (strong coronary vasodilators.) The patient who is physically able exercises progressively to gradually increase heart rate and myocardial O2 consumption, usually by walking and running on a treadmill at increasing rates and levels of inclination while blood pressure and ECG are monitored. The patient is questioned about any symptoms of angina. The onset of symptoms, especially when associated with significant ECG changes, is considered positive for ischemia. The development of hypotension associated with a stress test is an ominous sign that strongly suggests left main vessel or critical triple-vessel disease. The accuracy of a stress test alone is approximately 70%. The accuracy can be increased to approximately 90% with the addition of thallium or technetium. A stress thallium examination shows electrocardiographic changes of ischemia if they are present and graphically locates the ischemic myocardium by absence of tracer images in the ischemic area. In this test, the radioisotope is injected intravenously and the heart is scanned before and after stress is induced. Ischemic areas show diminished radiolabeled tracer activity (Fig. 4-7). Resting echocardiography easily shows regional wall motion abnormalities consistent with ischemia or infarction, and allows estimates of valvular dysfunction, pulmonary artery pressures, and chamber size. In patients with normal resting values, an inotropic challenge with dobutamine may unmask an ischemic myocardium (Fig. 4-8).
Figure 4-7 Dobutamine stress echocardiogram from a patient with prior inferior wallmyocardial infarction. End-diastolic and end-systolic frames from the apical twochamber left ventricular view are presented. End-diastolic and end-systolic screens are divided into four quadrants, with representative images at four dobutamine doses: 0, 10, 20, and 40 mcg/kg/min. Note decreasing chamber size with augmenting dobutamine stress. The inferior wall, indicated by black arrows, is akinetic at rest and remains akinetic at 40 mcg/kg/min dobutamine. At intermediate doses, there is no evidence for enhanced inferior wall systolic thickening, while the anterior wall, seen contralateral to the inferior wall, augments systolic motion with increasing dobutamine levels. Anatomically, the inferior wall should show extensive scar formation, with very little functioning or viable myocardium. Such a region would not respond favorably to revascularization.
Figure 4-8 Atherosclerotic obstruction of a coronary artery that causes marked reduction of blood flow to the affected heart muscle.
The decision to proceed with more invasive testing depends on the index of clinical suspicion (even in the absence of a positive stress test), the severity of the disease, and the concern over impending cardiac events (e.g., myocardial infarction). Invasive testing is recommended for symptomatic patients with high-risk clinical indicators (e.g., ischemic abnormalities on ECG, ST depression during exercise testing, concomitant peripheral vascular disease, age, diabetes, smoking history, and family history of atherosclerotic heart disease). All patients in whom ischemic heart disease is suspected ultimately undergo cardiac catheterization to determine whether coronary artery or valvular heart disease is present. This test remains the gold standard in the diagnosis of ischemic heart disease, although MR angiography and CT angiography may replace diagnostic coronary angiograms in the near future. A catheter is placed through a large peripheral artery (usually femoral) and passed retrograde to the heart and coronary arteries (left heart catheterization), or through a large vein (again, usually femoral) into and through the right side of the heart and out the pulmonary artery (right heart catheterization). Left heart catheterization includes coronary angiography, in which dye is injected directly into the coronary arteries to define their anatomy (Fig. 4-9) and measurements of pressure in the aorta and left ventricle. Left ventriculography (in which dye is injected into the left ventricular cavity to evaluate left ventricular function) is usually performed and the results are reported as a global ejection fraction with additional description of regional wall motion abnormalities. If valvular lesions are suspected, more extensive pressure measurements may be required.
Figure 4-9 Coronary angiography showing an obstruction in the proximal left anterior descending artery.
Coronary anatomy is commonly discussed in terms of the two major coronary arterial systems: the left main coronary artery and the right coronary artery. The left main divides into the left anterior descending (LAD) coronary artery and the circumflex coronary artery. The right coronary usually supplies a branch to the atrioventricular node and is the origin of the posterior descending coronary artery 80% to 85% of the time (right dominant system). Branches of the LAD are referred to as diagonal arteries and septal perforators. Obtuse marginal arteries are branches of the circumflex coronary artery. Obstruction of the left main coronary artery carries a significantly worse prognosis because both the circumflex and the anterior descending systems are included within its watershed. A 50% stenosis of a coronary artery visualized in two planes at angiography is a hemodynamically significant lesion. Studies of the natural history of coronary artery disease show that the prognosis worsens as the disease increases from one to three vessels, as would be expected when larger amounts of myocardium are placed in jeopardy. It is not enough, however, to denote single-, double-, or triple-vessel disease without allowing for variations in the amount of myocardium served by each vessel (i.e., myocardium at risk). For example, high-grade stenosis of a nondominant right coronary artery is not an indication for coronary revascularization, but high-grade stenosis of a large, dominant right coronary artery would be likely to require revascularization of some kind.
The presence of left ventricular dysfunction is significant in terms of prognosis, with and without further therapy, and may dictate treatment. When ventricular dysfunction is associated with large areas of scarred myocardium, revascularization may be of no value. However, “hibernating” myocardium that is nonfunctional because of chronic ischemia or “stunned” myocardium that is nonfunctional because of an acute ischemic episode from which it has not recovered may benefit from revascularization and may have improved long-term outcome. Current strategies allow safe coronary revascularization for patients with a very low (20%) ejection fraction when ischemic myocardium is present, but patients with ejection fractions of less than 20% often are not candidates for revascularization.
Treatment
Three types of therapy are available to patients with ischemic heart disease: medical, percutaneous interventions, and surgery. Treatment decisions must be individualized and are based on the symptoms, the anatomy, and the risks of the selected therapy. In all patients, however, risk factor modification of lifestyle and dietary habits may be of considerable benefit, especially when coordinated with smoking cessation, cholesterol-lowering strategies, and medical regimens selected on an individual basis.
Medical Management
When the risk of impending myocardial infarction is low, medical therapy to control symptoms may be appropriate. Medical therapy includes treatment with (β-blockers to minimize increases in heart rate in response to physical and emotional demands, calcium channel blockers to decrease afterload and prevent coronary spasm, and nitrates to decrease preload and dilate the vessels that supply ischemic coronary beds. In theory, only after all three modes of medical therapy have been used simultaneously and at maximally tolerated doses is a patient considered to have “failed medical therapy” and to be a candidate for another more invasive mode of treatment.
Percutaneous Interventions
Percutaneous transluminal coronary angioplasty (PTCA) was first introduced in the United States by Gruntzig in the early 1980s and radically changed the approach to coronary artery disease. PTCA also led to the further development of many procedures performed in the cardiac catheterization laboratory to open partially occluded coronary vessels percutaneously. In addition to PTCA, these procedures include laser angioplasty, directed atherectomy, and the placement of intracoronary stents. With techniques similar to cardiac catheterization, a guidewire is directed across the coronary lesion under fluoroscopic control. A PTCA balloon is passed over the guidewire and across the lesion. After the balloon is inflated, it compresses the lesion against the walls of the vessel (Fig. 4-10). Intracoronary stents made of fine metal mesh have been developed, and results show an increased likelihood of longer patency after angioplasty, as well as decreased risk of emergency surgery at the time of the procedure.
Figure 4-10 A, Narrowing of the coronary artery. B, Balloon inflated across the obstruction. C, The end result is near-normal diameter at the site of the previously obstructing lesion. (Reprinted with permission from Vogel JHK, King SB III. Practice of Interventional Cardiology, 2nd ed. St. Louis: Mosby-Year Book; 1993:84.)
Patients who undergo these procedures usually suffer little disability, and hospitalization is usually quite short. Return to normal activities within 1 to 2 weeks is not uncommon. There are some potential disadvantages, however. Recurrence rates of 25% to 30% are not uncommon within 6 months of the procedure, even when stents are used. In many cases, PTCA can be repeated. A new generation of “drug-eluting” stents to prevent local recurrence is being developed and may result in better long-term patencies. During 1% to 3% of all PTCA procedures or stent insertions, a patient may require emergency surgery because of a complication. In these cases, the surgical results are not as good as those for elective surgery. The rates of perioperative myocardial infarction and mortality are both higher.
Surgical Treatment
The decision to use coronary artery bypass grafting (CABG) to treat patients with ischemic heart disease is based on the anatomy, the symptoms, and the potential risks to the patient, as well as the long-term benefits of the operation. The American Heart Association and the American College of Cardiology have developed specific guidelines for the selection of patients being considered for CABG. Certain anatomic situations alone (e.g., left main artery disease, left main equivalent, proximal LAD, and circumflex occlusions) may warrant surgery, even in the absence of symptoms, because of the large amount of myocardium in jeopardy and the recognized high mortality rate without surgical treatment (especially sudden death). Patients with stable angina that is unresponsive to medical therapy, unstable angina (e.g., pain at rest, preinfarction angina, postinfarction angina), or double- or triple-vessel disease with diminished left ventricular function are likewise candidates for surgical intervention. In these patients, surgery usually relieves symptoms, prevents myocardial infarction, and prolongs life. In addition, concomitant CABG may be indicated for patients who undergo surgery for complications of myocardial infarction (e.g., acute mitral regurgitation, ventricular septal defect, free rupture of the heart) or those who undergo elective valve replacement procedures when critical vessel occlusions are present. Recent comparisons of PTCA alone and CABG, when both are feasible, have favored surgery for patients with long life expectancies.
CABG can be performed with or without the use of the heart–lung machine and its potential complications. Traditionally, cardiopulmonary bypass has been used to provide a stable, bloodless field in which to perform the precise distal anastomoses to the coronary arteries required for successful long-term results. Off-pump coronary artery bypass surgery (OPCAB) has been introduced as a method of performing CABG without the use of cardiopulmonary bypass in the hope of reducing some of the complications (especially neurologic) associated with its use. OPCAB is done through a standard median sternotomy or, in certain circumstances, via a limited thoracotomy. Once the technique has been mastered and the “learning curve” has been passed, many centers report excellent results, especially with the use of new stabilizing devices developed specifically for this procedure. Long-term results of OPCAB must be evaluated and compared with the standard techniques of coronary bypass surgery before they can be readily accepted.
For some patients, the risks of surgery far outweigh its benefits. For example, patients with limited life expectancy from other diseases, the very elderly, and the physically impaired might not be surgical candidates because of associated conditions. In these cases, further medical treatment or attempts at partial revascularization with PTCA may be more appropriate. Although the benefits of CABG to patients with decreased ventricular function and double- or triple-vessel disease are well recognized, poor ventricular function adds to the mortality of patients who undergo the operation. Other factors that increase the mortality and morbidity rates associated with CABG and other open-heart procedures include age greater than 70 years, morbid obesity, diabetes, chronic obstructive pulmonary disease, hypertension, history of myocardial infarction, reoperation, chronic renal insufficiency or failure, peripheral vascular disease, and, possibly, female sex.
On the day of surgery, the patient receives preoperative medications, including prophylactic antibiotics to minimize the risk of perioperative infection. In many cases, the morning dose of cardiac drugs is given, especially if the patient has been taking a (β-blocker. Monitoring devices (Swan-Ganz catheter, CVP, arterial line) are inserted and the patient is anesthetized. Once the patient has been prepped and draped, the incision used to expose the heart (usually a median sternotomy) is performed. For almost all CABG procedures, one or both of the internal mammary arteries is harvested from beneath the chest wall. The other conduits to be used for bypasses (saphenous veins from the leg or radial artery from the forearm) are harvested simultaneously under direct vision or using endoscopic techniques to minimize the extent of the skin incisions. The patient is heparinized and cardiopulmonary bypass is initiated with the heart–lung machine. The aorta is cross-clamped and the heart is arrested with cardioplegia to provide a stable, bloodless field in which to perform the distal microsurgery and to protect the heart during these periods of global ischemia. Bypasses are then performed. While using surgical loops to magnify the operative field, the surgeon makes a small opening in the native coronary artery. The size of the opening is approximately twice the diameter of the artery. The conduit used to bypass the obstruction in the coronary artery is then sutured to it. The bypass is completed by suturing the proximal end of the conduit to the aorta with the cross-clamp still in place or with a side-biting clamp after the cross-clamp has been removed. The proximal end of the mammary artery, where it branches from the subclavian artery, is left intact. After the patient is weaned from the heart–lung machine, the heparin is reversed with protamine, hemostasis is obtained, and the incisions are closed. Temporary pacing wires are usually placed on the atrium and ventricle, should they be needed. Chest tubes are placed to drain blood from around the heart and from the pleural space.
Preoperative and Postoperative Care. After the decision is made to proceed with surgery, appropriate preparation is necessary. Most elective CABG and valve procedures are now performed with the patient admitted to the hospital on the morning of surgery. Anticipated discharge home for low-risk patients is on the fourth or fifth postoperative day. Consequently, almost all preparations are made on an outpatient basis. Other patients with acute symptoms or unstable coronary syndrome (as many as 50% in some institutions) will require surgery while in the hospital following catheterization. Regardless of their status, a complete history and physical examination is required. In addition, attention is directed to documenting the absence of associated carotid disease, which could increase the risk of stroke at the time of CABG. The finding of significant carotid stenosis may prompt pre-CABG carotid endarterectomy or a simultaneous approach to decrease the risk of perioperative stroke. The presence of suitable conduits for the procedure must be verified, especially in patients with varicose veins, a history of deep-vein thrombophlebitis, a previous operation, or severe peripheral vascular disease. The patient and the family are given extensive education about the short- and long-term risks and benefits of the procedure, about the hospitalization itself, and about the postoperative recuperation. Many centers promote autologous blood donation in elective cases to reduce the need for homologous blood transfusion.
Years of experience and attention to detail in the preoperative, intraoperative, and postoperative care of patients who undergo CABG has resulted in almost routine care of these patients postoperatively. Many patients are extubated shortly after they return to the recovery unit. They are often transferred from the intensive care unit in less than 24 hours. Early mobilization is emphasized, as are the prevention of pulmonary problems and the prophylactic treatment of atrial fibrillation (incidences of up to 40% are reported) with (β-blockers or other drugs. Aspirin is prescribed to increase long-term bypass graft patency, unless contraindicated. Because of the fluid weight gained during the surgery, aggressive diuresis to return patients to their preoperative weight is emphasized. Discharge from the hospital on the fourth or fifth postoperative day is not uncommon, especially for patients younger than 65 years of age who do not have associated disease.
Patients who are discharged from the hospital usually return to a lifestyle similar to the one they had before the onset of the ischemic syndrome. At discharge, they are likely to feel somewhat weakened, as though they had a “bad case of the flu.” These patients should be ambulatory, however, soon after they return home. Early complications of heart failure, leg wound infection, or sternal infection are not common, but must be recognized and treated appropriately. Recovery over 4 to 6 weeks is one of gradually increasing ambulation and activity. At some point, most patients undergo a repeat stress test. If the stress test documents the absence of ongoing ischemia, most patients are enrolled in an exercise rehabilitation program for rapid reconditioning. Most patients can return to work within 6 to 8 weeks of discharge from the hospital, especially if their work is primarily sedentary. Patients whose work requires heavy physical activity may need to wait 3 to 6 months after discharge to permit the sternum to heal completely.
Postoperative Complications. Some patients who undergo CABG, as well as other cardiac surgery procedures, have a course that is far from routine. The care of these patients can be a challenge to the surgical team. The survival of these patients is based on rapid recognition of problems as they develop and rapid and appropriate responses to them. Close monitoring with invasive devices (e.g., arterial line, Swan-Ganz catheter) and appropriate therapeutic interventions can prevent many complications. Monitoring of blood pressure, pulmonary capillary wedge pressure, CVP, and cardiac output has made it easier to differentiate among the many major early postoperative complications. Hypotension caused by volume loss, cardiac tamponade, low peripheral resistance, or cardiac failure can be diagnosed and treated. Hypovolemia requires rapid replacement of blood volume. Signs of tamponade necessitate early return to the operating room or emergency opening of the median sternotomy incision in the intensive care unit to relieve the tamponade and correct the source of bleeding. Low resistance may require α-adrenergic agents to improve vascular tone. Myocardial failure requires treatment with inotropic agents. Agents that have direct (β-adrenergic effects on the heart are most appropriate and include dopamine, dobutamine, and epinephrine. The newer phosphodiesterase inhibitors (e.g., milrinone) are also helpful agents to increase cardiac contractility.
If these interventions do not reverse a low-output state due to cardiac failure, the use of an intraaortic balloon pump
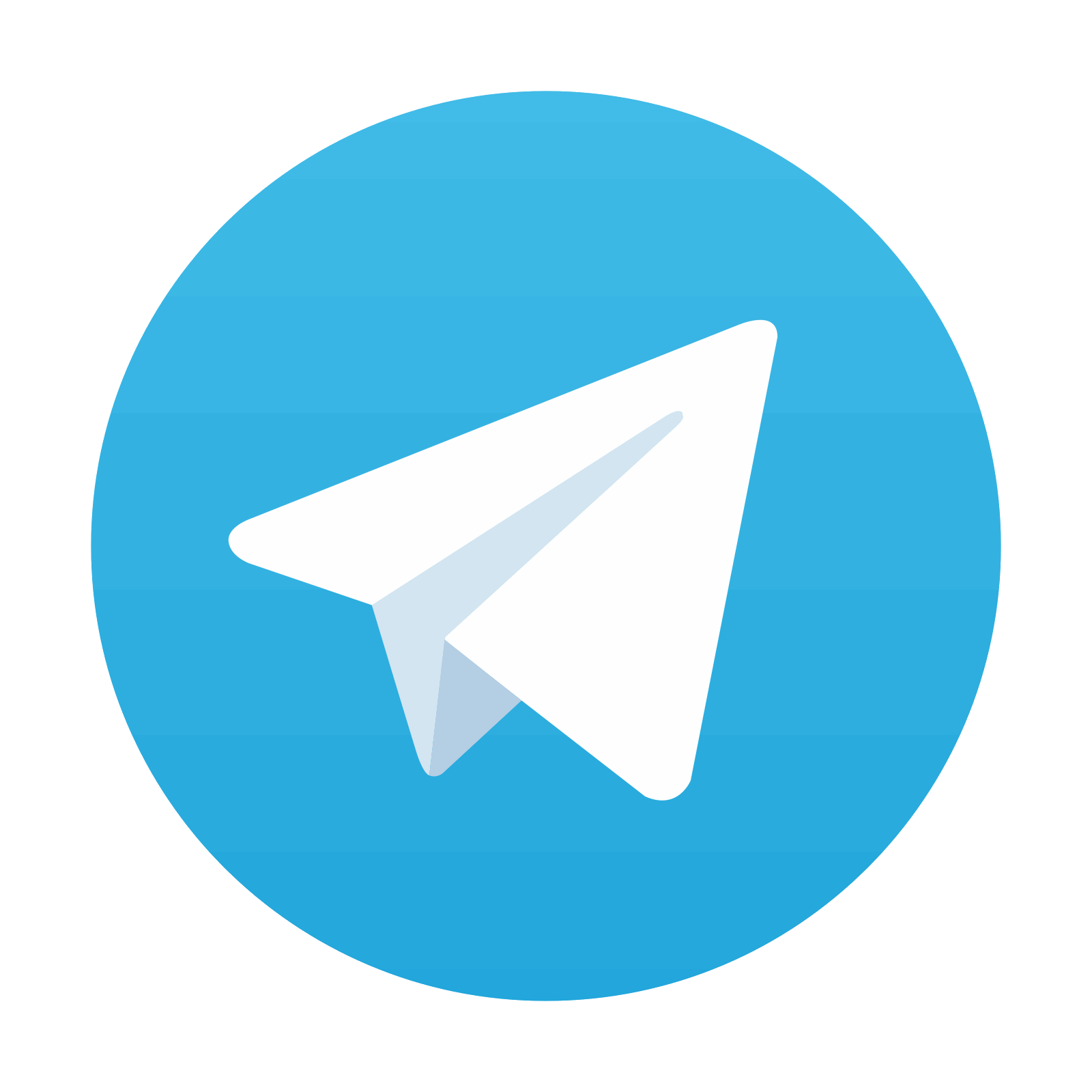
Stay updated, free articles. Join our Telegram channel

Full access? Get Clinical Tree
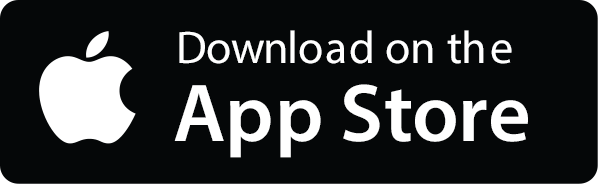
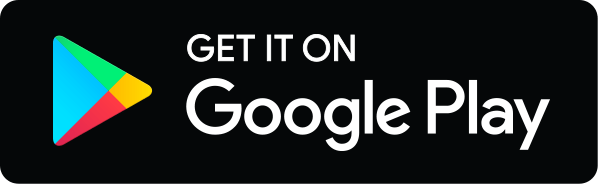