OBJECTIVES
1 Describe the information that the anesthesiologist should obtain from the patient during the preanesthetic visit.
2 Differentiate among the types of anesthesia, and give the indications for selection of type, based on the various surgical procedures.
3 Distinguish between general and regional anesthetics in terms of time course, general pharmacology, and toxicology.
4 Describe the pharmacologic and physiologic properties of commonly used sedative and local anesthetic agents.
5 Discuss the basic principles of airway management.
6 Describe the methods and devices used in anesthesiology to monitor the patient’s oxygenation, circulation, respiration, and temperature.
7 Describe the immediate complications of anesthesia that occur in the postanesthesia care unit and discuss their management.
8 Discuss the management of postoperative pain.
9 Discuss the ways in which chronic medical conditions affect the patient in the perioperative period, and describe any special preoperative testing and preparation that is required.
Anesthesiologists render patients insensitive to surgery, manage their medical problems, and maintain their physiology at near normal during and immediately after the operative period. They are also involved in caring for patients in critical care units, managing the pain of labor and delivery, treating acute pain in the postoperative or postinjury period, and managing chronic pain syndromes. Basic knowledge in anesthesiology is derived from the fields of pharmacology and physiology and is helpful to physicians who manage patients who require surgery. This chapter discusses the role of the anesthesiologist in perioperative care, specific intraoperative drugs and anesthesia techniques, monitoring in the operative period, management of acute postoperative pain, and implications of concurrent medical conditions in the perioperative period. This chapter will familiarize students with the risks and hazards of anesthesia so that they can learn to prepare a patient, both physically and psychologically, for anesthesia and surgery. Students will also become acquainted with problems that are specific to anesthesia so that they can consult with the anesthesiologist about medical problems that may affect the patient’s response to anesthesia and surgery. Because many types of physicians supervise sedation for minor procedures, a brief discussion of local anesthetic and sedation drugs will provide basic knowledge necessary to ensure patient safety during those procedures.
PREOPERATIVE EVALUATION
Except in an emergency, no patient should undergo surgery until he or she is in optimal medical condition. Before surgery, the anesthesiologist must evaluate the patient’s risk for the anesthetic and facilitate plans for anesthetic technique, monitoring, and postoperative care. During the preoperative evaluation, the anesthesiologist must prepare the patient and family psychologically for the upcoming procedure and must also establish physician–patient rapport. The anesthesiologist should discuss the planned anesthetic technique with the patient and document that the risks and benefits have been reviewed. The anesthesiologist should tell the patient when to stop eating and drinking before surgery, indicate the projected time of surgery, discuss the planned premedication, and explain what monitors will be used in the operating room. The patient should be prepared for the recovery room and recognize the possible need for ventilatory assistance, prolonged endotracheal intubation, or invasive monitoring. The patient should be told to expect some discomfort, be aware of postanesthesia procedures, and understand how he or she can contribute to restoring normal function (e.g., by coughing and deep breathing). Finally, the patient is asked to give informed consent for the anesthetic and monitoring interventions.
Traditionally, the preoperative evaluation for anesthesia was performed in the hospital on the day before surgery. Early preoperative evaluation allows the anesthesiologist and surgeon time for appropriate laboratory tests and consultations. Today, many patients arrive at the hospital on the day of surgery and must be evaluated either as outpatients or immediately before surgery. Test results or consultations may be difficult to obtain on short notice, so a clinic visit a few days before surgery is often arranged for any patient who is suspected of having a medical condition that might affect the choice of anesthetic technique. Some practices use telephone or computer-assisted screening to determine which patients should come to the preanesthesia clinic. In emergency procedures, the preoperative visit is made just before surgery because little time is available to test or adjust the patient’s condition.
On completion of the preoperative visit, a summary of pertinent findings is written in the patient’s record. Included in the summary are details of the patient’s medical and anesthesia history, the patient’s medication history, pertinent family history, physical examination findings, and laboratory data. Healthy, asymptomatic women who are younger than 40 years of age and have normal findings on physical examination need few laboratory tests (e.g., complete blood count [CBC] or just a hematocrit), although many experts think even these are not productive. Men with a similar profile often require no tests. The laboratory data that are traditionally requested for healthy patients who are older than 40 years of age include CBC, urinalysis, serum electrolytes, renal function tests, electrocardiogram, and chest x-ray, if indicated. Recent recommendations for patients who are 40 to 49 years old do not include a chest x-ray (unless there is a history of cardiac or pulmonary disease) or CBC, unless major blood loss is anticipated. Pregnancy tests are often ordered for women of childbearing age, but the American College of Obstetricians and Gynecologists recently issued a consensus report indicating that the routine use of such a test for anesthesia purposes is not cost-effective because little information indicates that anesthesia presents a risk to the fetus. Further tests are requested based on history, symptoms, or surgical procedure. Finally, a physical status classification is assigned based on criteria established by the American Society of Anesthesiologists (ASA). The ASA classification is not a prediction of anesthetic risk (of death) per se, but rather a description of the overall condition of the patient for purposes of comparison with other patients (Table 1-1). In cases of elective surgery where the patient is not in optimal medical condition, the anesthesiologist should discuss with the patient’s primary physician or surgeon the possibility of postponing surgery.
History
The preanesthetic history should include a review of the following systems: cardiovascular, pulmonary, renal, hepatic, endocrine, metabolic, hematologic, and central nervous. For a thorough discussion of the interaction between disease and anesthesia, see the section on patient factors that influence anesthesia. A brief dental history should also be obtained, and the presence of dentures, loose teeth, and chipped, missing, or capped teeth noted. The time, composition, and amount of the last oral intake should be determined. In women, an obstetric and gynecologic history that includes the date of the last menstrual period should be obtained. A social history should include drug, alcohol, and tobacco use. A history of previous surgical and anesthetic procedures is important, including type of anesthesia and complications. A family history of anesthetic problems should also be obtained to rule out inherited conditions (e.g., cholinesterase abnormalities, malignant hyperthermia, porphyria). The medication history should include the names of drugs, their dosage and schedule, and allergies or unusual reactions. The time of the last dose is also noted. Dietary supplements and other herbal or nontraditional medicines are investigated because of the actions many have on sedative pharmacodynamics and coagulation function. Most medications are continued until the time of surgery. The exceptions are anticoagulants, herbal medicines that cause anticoagulation effects, and aspirin. If anticoagulant therapy is needed, warfarin should be withdrawn and heparin substituted for titration through the perioperative procedure.
Physical Examination
The physical examination is usually confined to the upper airway, the lungs, and the cardiovascular system. To assess possible difficulties in airway management, evaluation of the head and neck should include the size of the mouth and tongue, the condition of dentition, and the range of motion of the temporomandibular joint and cervical spine. Obesity, short neck, and tracheal deviation signal potential difficulties. The lungs are auscultated for the presence of abnormal breath sounds, rales, and wheezing. The cardiovascular examination consists of measurements of blood pressure, skin turgor, heart rate and rhythm; auscultation for cardiac murmurs and carotid bruits; and, in appropriate situations, assessment of intravascular volume status. If nasotracheal intubation is anticipated, the patency of both nasal passages should be assessed. Sites for intravenous access, invasive monitoring, and regional anesthesia should be examined for infection and anatomic abnormalities.
Psychological Preparation and Preoperative Medication
The goals of premedication are sedation, anxiolysis, and pain relief. In the immediate preoperative period, the patient should be calm and pain-free, but cooperative and easy to arouse. There is no recipe for this mental state, and drugs and doses must be tailored to the individual patient. Some anesthesiologists believe that the psychological preparation provided by the preoperative visit should address the patient’s fear, thereby precluding the need for pharmacologic premedication. Elderly and debilitated patients usually require reduced or no premedication. Patients with increased intracranial pressure (ICP), chronic or acute lung disease, blood volume depletion, or altered levels of consciousness should not be premedicated until they are under the constant observation of the anesthesiologist, if at all. Premedication is often avoided in outpatients because it may prolong recovery time. Premedicants are classified as sedatives, narcotics, and anticholinergics. Other drugs administered before anesthesia might include those designed to reduce the possibility of regurgitation and aspiration of gastric contents or to prevent an allergic reaction to intravenous dyes and other drugs administered intraoperatively (most notably vancomycin). Table 1-2 lists commonly used premedication drugs with their doses and timing of administration.
Sedatives
Benzodiazepines and antihistamines are commonly used as preanesthetic sedatives. Benzodiazepines are tranquilizers that produce minimal cardiorespiratory depression when used in appropriate doses. They usually produce anterograde amnesia and may cause prolonged sedation. However, they are well known to ameliorate postanesthesia delirium, especially in children. Antihistamines have both sedative and antiemetic properties. They potentiate the sedative and analgesic properties of narcotics.
Narcotics produce analgesia in patients with preoperative pain. They are also used to reduce discomfort in patients who are undergoing insertion of invasive monitoring lines or regional anesthesia. Because narcotics produce respiratory depression, they are best avoided in patients with decreased pulmonary reserve and in those in whom respiratory depression may be harmful (e.g., patients with increased ICP). Side effects include nausea, vomiting, orthostatic hypotension, and smooth muscle contraction. Narcotic premedication should be administered intravenously only when the patient can be monitored full time until induction of anesthesia.
Anticholinergics
Anticholinergic drugs are administered for their antisialagogue (salivation-reducing) effect, for prevention of reflex bradycardia (especially in children), and for their sedative and amnesic effects. Their intramuscular administration 1 hour before surgery has fallen into disfavor with many anesthesiologists because all of these agents are as effective and much more comfortable for the patient when given intravenously immediately before the induction of anesthesia. An antisialagogue effect is useful for intraoral surgery, when intraoral or nasal topical anesthesia is used, when intubation is planned with a fiberoptic bronchoscope, or when the use of a laryngeal mask airway is anticipated. Scopolamine and, to a lesser degree, atropine cross the blood–brain barrier and produce sedation and amnesic effects.
Side effects of anticholinergic drugs include central nervous system (CNS) toxicity (delirium or prolonged somnolence after anesthesia), tachycardia, elevation of body temperature, relaxation of the lower esophageal sphincter that results in passive gastric reflux, mydriasis, and cycloplegia. If the drugs are in effect while patients are conscious, patients usually complain of a dry mouth.
Prophylaxis for Pulmonary Aspiration
Sedation or anesthesia decreases both gastroesophageal sphincter integrity and airway protective reflexes, increasing the risk of aspiration. Although there is still some controversy about this, normal patients are usually assumed to have an empty stomach if they have refrained from solid food for 6 hours and from clear liquids (anything that can be seen through well enough to read) for at least 2 hours before induction. Infants may have milk up to 4 hours before anesthesia. Characteristics of patients who are at high risk for aspiration pneumonitis are listed in Table 1-3. Gastric contents flow into the pharynx either actively or passively and then are inhaled into the trachea during spontaneous or controlled respiration. An endotracheal tube with the cuff inflated is not a reliable seal of the trachea. The adverse effect of aspiration pneumonitis is markedly reduced by decreasing the acidity and volume of gastric contents.
Acid Reduction
The H2 antagonists cimetidine (Tagamet), ranitidine (Zantac), and famotidine (Pepcid) increase gastric fluid pH by inhibiting the ability of histamine to induce the secretion of gastric acid. Cimetidine prolongs the elimination of many drugs, including diazepam, lidocaine, theophylline, and propranolol. Proton pump inhibitors are effective for at least a day after regular use is discontinued and effectively prevent acid reflux. They include omeprazole (Prilosec), esomeprazole (Nexium), rabeprazole (Acidophex), lansoprazole (Prevacid), and pantoprazole (Protonix). Because particulate antacids (e.g., Maalox) can produce serious pneumonitis if aspirated, they are not used in this setting. Sodium citrate (Bicitra) orally on call to the operating room or immediately preinduction, is a safe, effective means of increasing the pH of gastric contents when a full stomach is anticipated. Although this agent increases the volume of gastric contents slightly, its ingestion does not increase the incidence of regurgitation or vomiting on induction of anesthesia.
Volume Reduction
Metoclopramide (Reglan) is a cholinergic stimulant that speeds gastric emptying by increasing the motility of the upper gastrointestinal tract and decreasing pyloric sphincter tone. It decreases intragastric volume, but has no effect on gastric fluid pH. It should be included in the preoperative preparation of patients who are suspected of having a significant volume of gastric contents.
OPERATIVE ANESTHETIC TECHNIQUES
Anesthetic techniques fall into one of three categories: general, regional, or local. These may be used either alone or in combination. General anesthesia is maintained by the use of gas, volatile anesthetics, intravenous drugs (narcotics, sedatives), and often muscle relaxants. Regional anesthesia is achieved by central or regional techniques. Central techniques include spinal, epidural, and caudal anesthesia. Peripheral techniques include nerve, plexus, and intravenous (Bier) blocks. The surgeon usually performs local anesthesia (infiltration of the tissues at the surgical site), although the anesthesiologist may stand by to monitor and sedate the patient, thus managing the patient’s ongoing medical condition during surgery. The choice of anesthetic technique is the combined responsibility of the anesthesiologist, surgeon, and patient; often, there are several comparable options. After the alternatives, risks, and benefits of each are explained, the patient should be allowed to choose a technique. When deciding on anesthetic technique, several factors are taken into consideration. Most important is whether a local or regional technique will provide adequate analgesia given the site of surgery. The duration of the procedure and the position of the patient during the procedure must also be considered. Patient factors that influence the choice of anesthetic include the presence and type of coexisting diseases, bleeding tendencies, infection, patient age, and the possibility of a full stomach. Finally, the surgeon’s and anesthesiologist’s personalities and skill are important to consider when suggesting that a patient be conscious during surgery.
General Anesthesia
General anesthesia should provide analgesia, unconsciousness, muscle relaxation, and autonomic control. It is used whenever complete insensitivity is required, when the surgery involves a part of the body that is not amenable to regional or local anesthesia, when mechanical ventilation is necessary, or when the position required for the procedure does not allow the patient to be comfortable when awake. General anesthesia is achieved with a combination of inhalational anesthetics and other drugs (e.g., narcotics, sedatives, muscle relaxants).
Induction
Before induction, the patient is preoxygenated to minimize hypoxemia on induction. Induction begins with the intravenous injection of an ultra–short-acting drug, usually a barbiturate or propofol. Table 1-4 lists other drugs that cause rapid loss of consciousness and may be used. When intubation of the trachea is planned, a depolarizing (succinylcholine) or nondepolarizing (pancuronium, atracurium, vecuronium, mivacurium, cisatracurium, rocuronium) neuromuscular-blocking agent (muscle relaxant) is given to facilitate the process. An alternative to intravenous induction is inhalation induction, which is more gradual and may not be as pleasant for the patient. For inhalation induction, the patient breathes oxygen with or without nitrous oxide and a gradually increasing concentration of volatile anesthetic agent, usually sevoflurane or halothane. Enflurane, isoflurane, and desflurane are usually considered too pungent for a smooth mask induction.
Intravenous Induction Agents
Intravenous induction agents cause rapid loss of consciousness because their early peak plasma levels are delivered directly to the brain. Consciousness returns when the drug is redistributed to other tissues. Differences in their elimination half-lives cause lingering mild sedation after one dose with some agents and, in most cases, prolonged effects with continuous infusion or repeated doses. Specific agents and their characteristics are listed in Table 1-4. The barbiturate thiopental is most commonly used because it is inexpensive. Pharmacologic differences among the induction agents are summarized in Table 1-5.
The choice of an induction agent depends on the patient’s preexisting medical condition, the length of the surgery, the anticipated length of hospital stay, and the particular needs of the anesthetic. Rectal or intramuscular induction is used in children who will not allow an intravenous catheter; it also avoids mask induction with inhalation agents. Either route allows a young patient to fall asleep outside the operating room while held by the parents. The child is then transported to the operating room. Monitors and an intravenous catheter are placed, and anesthesia is begun without frightening the child. Induction by these alternative routes has the disadvantage of prolonging the recovery period. Ketamine is different from the other induction agents, whether used intramuscularly or intravenously, because of its unique CNS actions. The drug is a hallucinogen in adults and causes signs of excitement in children. Anesthetic depth is difficult to assess because there are often neuromuscular excitatory signs such as nystagmus, purposeful and nonpurposeful movements, and myoclonus. During the recovery period, auditory stimulation causes excitement in children that may be secondary to nightmares. Although ketamine induces signs of CNS stimulation, paradoxically, it suppresses seizure activity. When administered in very small doses, ketamine is an excellent sedative for use with local or regional anesthesia. Its profound analgesic effect is not seen in any other nonnarcotic intravenous anesthetic, so it is often used for short, painful procedures (e.g., dressing changes, suture removal or insertion, fracture reductions in children).
Barbiturates remain the most popular induction agents. Porphyrias and allergy are the only contraindications. Although etomidate, the benzodiazepines, and ketamine support blood pressure in normal patients, this effect does not extend to patients with hypovolemia or little cardiac reserve. The search for new induction agents results from a desire to identify one that has a very short elimination period, a good analgesic effect, and no undesirable emergence effects. Propofol is especially popular in the ambulatory setting because it approaches the ideal in its characteristics, although it is the most expensive agent.
Maintenance
Inhalation agents, intravenous agents (discussed earlier), or a combination of both are used to maintain anesthesia. These agents may be supplemented with a muscle relaxant, depending on the need for operative exposure and controlled ventilation. All drugs used for anesthesia are selected according to their pharmacologic effects on the patient’s physiologic function, keeping in mind the patient’s medical condition and the surgical requirements. The anesthesiologist’s goal is to minimize the stress response to surgery while maintaining the patient’s cardiovascular system as close to the preoperative state as possible.
Inhalation Agents
Inhalation agents have the most profound effects on the physiology of the patient, adding to or even potentiating the effects of other drugs. The five agents commonly used are nitrous oxide, halothane, isoflurane, desflurane, and sevoflurane. Enflurane is still available but is used less commonly in the United States. The physical characteristics of these agents are compared in four areas: the minimum alveolar concentration (MAC), vapor pressure, blood–gas solubility, and lipid–gas solubility. MAC is the alveolar concentration of gas at 1 atmosphere that prevents movement in response to a surgical stimulus (e.g., skin incision) in 50% of patients. It is the standard by which the potency of anesthetics is compared and is roughly proportional to the lipid solubility. The vapor pressure determines the concentration of agent in the carrying gas. Blood–gas solubility is a measure of how much of the agent remains dissolved in the blood as it is carried to the brain and other tissues. Lipid–gas solubility determines how easily the agent enters brain and neural tissue to exert its anesthetic effect and is roughly proportional to its potency. Table 1-6 summarizes the important physical characteristics of inhaled agents currently in use and gives the MAC of each. Table 1-7 summarizes the physiologic effects of the commonly used inhalation agents. All of the agents depress respiration (N2O less so than the volatile agents) and the cardiovascular system, although these effects are moderated by varying degrees of autonomic reflex depression.
Nitrous oxide is different from the other inhaled agents (see Tables 1-6 and 1-7). First, it is a gas, whereas the others are volatile agents: they are supplied as liquids and are vaporized in a carrier gas. In most hospitals, N2O is piped in through a central system. Otherwise, it is supplied in blue cylinders that attach to the anesthesia machine. Its MAC of 104%, determined under hyperbaric conditions (greater than atmospheric pressure), indicates its relative impotency. Because anesthetic gas mixtures should deliver at least 30% oxygen to ensure adequate tissue oxygenation, it is possible to administer safely a maximum of only 70% N2O at sea level, and less at high altitudes. It is evident, then, that N2O cannot be used as the sole anesthetic in most patients. It must be supplemented with narcotics, intravenous induction agents (e.g., propofol) in a continuous infusion, or a volatile agent to cause unconsciousness and analgesia. Because it has few effects on the cardiovascular system and is an excellent analgesic, it is often used to potentiate the effects of other anesthetic agents. Its principal drawback is that it diffuses into closed air spaces much faster than nitrogen can diffuse out, and so causes a rapid increase in the volume of entrapped air. Thus, it is not indicated for use in surgery for an obstructed bowel or for any other abdominal surgery where an increased volume of bowel gas would interfere with the surgical procedure, increase trauma, or make closure difficult. Nitrous oxide is contraindicated in patients with pneumothorax, large alveolar bullae, and pneumocephalus. It must be used with caution in patients who need a high inspired oxygen concentration for any reason. It is also the only inhaled anesthetic that has toxic effects with prolonged exposure. Bone marrow depression, chronic neuropathy, and possible teratogenicity are also reported. It inactivates methionine synthetase, which in turn inhibits vitamin B12 for up to 4 days after exposure. This inhibition is postulated to be the reason for its toxicity. It must be emphasized, however, that N2O is still used in many general anesthetics, and it is often the analgesic of choice for dental and other procedures. When patient outcomes are compared, it is the safest of all anesthetics. The advantages of N2O are that it is not metabolized, is rapidly taken up and exhaled, is easy to administer, and causes minimal cardiovascular and respiratory depression at clinical concentrations.
The hepatic microsomal enzymatic system metabolizes all of the volatile agents to varying degrees. The kidneys excrete most of the metabolites (Table 1-8). The lungs excrete unmetabolized drug. In hepatic hypoxia, a reductive pathway may metabolize halothane. It is postulated that the metabolites from this reductive metabolism cause hepatocyte toxicity. Thus, halothane has the undeserved reputation of being a liver toxin. In the national halothane study of the 1960s, halothane hepatitis, or massive hepatic necrosis, occurred in 1 of 35,000 administrations. Besides the direct toxicity, in some patients there appears to be an immune component to halothane-related liver dysfunction. Current recommendations are that halothane not be administered to any patient who has a history of unexplained liver dysfunction after a previous halothane anesthetic or to one who has altered liver perfusion for any reason. Hepatic dysfunction after the administration of the other inhaled anesthetics is believed to be related to global or surgical effects on liver perfusion rather than to the agent itself.
The metabolites of all volatile agents include small amounts of inorganic fluoride ion, but in concentrations that are too low to cause renal toxicity. Halothane metabolism may also cause a measurable blood level of bromine, which may have some sedative effects of its own. Halothane is measurable in exhaled air many hours after the anesthesia is discontinued. Its metabolites are measurable in urine up to several days postoperatively.
In summary, with the exception of halothane, the inhaled anesthetics are remarkably inert metabolically. They rarely cause toxicity. Although they cause profound cardiovascular, respiratory, and neurologic system depression, they are safe when used under controlled conditions. Combining volatile agents with N2O, and all inhaled agents with the adjunctive drugs discussed below, produces profound general anesthesia with little interference with tissue perfusion and oxygenation.
Muscle Relaxants
Muscle relaxants allow a much lighter plane of anesthesia to provide conditions required for surgery. They facilitate such procedures as endotracheal intubation and abdominal surgery, which require a degree of muscle relaxation that often cannot be safely achieved by inhalation agents alone. Controlled ventilation is also facilitated by muscle relaxants, extending their use beyond the operating room and into intensive care units.
The normal reflex response to a noxious stimulus is withdrawal. Movement of a patient during anesthesia indicates that the depth of anesthesia is not sufficient to prevent this motor response. Because muscle relaxants might mask a sign of inadequate anesthesia, the anesthesiologist relies on autonomic rather than motor reflexes as a sign of depth of anesthesia. These reflexes include increases in heart rate or blood pressure, attempts at spontaneous respiration, sweating, increased respiratory tract secretions, and bronchoconstriction. Thus, the use of muscle relaxants is a safe method to reduce inhalational anesthetic requirements to a level that does not cause dangerous cardiovascular depression and other ill effects.
Two classes of muscle relaxants are in use today. The depolarizing relaxants activate the postsynaptic cholinergic receptor of the neuromuscular junction, then dissociate slowly from that receptor, binding it so that it will not fire again until it is free. The nondepolarizing relaxants prevent muscle contraction by competitively blocking the postsynaptic receptor from binding with acetylcholine, but do not activate the receptor. Monitoring the response to single and repeated supramaximal twitch stimuli and tetanic stimulation differentiates the two types (Table 1-9) and determines the percentage of receptors blocked at any one time. A simple nerve stimulator applied over the ulnar nerve at the elbow or wrist or the ophthalmic branch of the trigeminal nerve that is just anterior to the ear is used to monitor twitch response. A single stimulus applied at a rate of 1 per second, a train-of-four stimulation applied at a rate of 2 per second, or a tetanic stimulation applied at 50 or 100 cycles per second may be used to determine the percentage of muscle receptors blocked by the muscle relaxant. As shown in Table 1-9, 75% to 80% of receptors must be blocked before the twitch response is depressed, whereas 90% to 95% of receptors must be blocked before there is no response to a single twitch. Fade (a progressive decrease in the height of successive twitches) is seen in the train-of-four stimulation sequence when 70% to 75% of receptors are blocked. A train-of-four sequence consists of four supramaximal stimuli delivered at a rate of 2 cycles per second (2 Hz). Tetanic stimulation at 50 Hz leads to appreciable fade when 75% to 80% of receptors are blocked. At 100 Hz, fade is seen when 50% of receptors are blocked.
Succinylcholine, the only depolarizing agent currently in use, consists of two acetylcholine molecules joined together. It produces relaxation of short duration after initial generalized contraction, which is clinically visible as fasciculations. Succinylcholine is not metabolized at the neuromuscular junction by acetylcholinesterase. Instead, it diffuses into the blood, where it is rapidly hydrolyzed by plasma cholinesterase (pseudocholinesterase). Its onset of action is almost immediate, and a single dose of 1 mg/kg has a duration of approximately 10 minutes. Despite its side effects (Table 1-10), it is the choice of many anesthesiologists for endotracheal intubation because of its rapid onset. It is the relaxant most used for emergency intubations. Many of these side effects can be ameliorated by preceding the injection with a small, defasciculating dose of a nondepolarizing relaxant (d-tubocurarine 3 mg). However, this action also increases the time to onset, the duration, and the dose of succinylcholine required to suppress neuromuscular transmission. Nondepolarizing relaxants do not have these side effects.
Hyperkalemia after succinylcholine injection occurs as a consequence of depolarization. It can be clinically significant in patients with massive soft tissue injury, including burns (at least 48 hours old), acute and chronic denervation injuries, renal failure, or preexisting hyperkalemia. In these situations, cardiac arrest is not uncommon and is not effectively prevented by pretreatment with nondepolarizing agents. Increases in intracranial and intraocular pressure occur with succinylcholine, even in the absence of both fasciculations and increased venous pressure. The reason for the increase in ICP is not known, but the rise in intraocular pressure is initiated by contracture of extraocular muscles and may be enhanced by transient dilation of choroidal blood vessels. Therefore, extrusion of vitreous may occur in patients with an open globe injury. The increase in intra-abdominal pressure is caused by contractions of the abdominal muscles and may be significant enough to cause regurgitation of stomach contents and aspiration into the lungs if protective measures are not taken. Nonetheless, succinylcholine is the favorite relaxant of many anesthesiologists for rapid endotracheal intubation, especially in patients with a full stomach, because of its rapid, reliable onset of action.
Succinylcholine triggers the onset of malignant hyperpyrexia (MH) in susceptible individuals. MH is a rare, inherited myopathy in which muscles undergo sustained contracture when triggered by depolarizing relaxants, inhaled anesthetics, or other physiologic stressors. A hyper-metabolic state ensues that is characterized by hypertension, tachycardia, hyperkalemia, hypercapnia, and hyperthermia. MH is often associated with chronic neuromuscular diseases. Because these diseases may be subclinical in small children at the time of surgery, some anesthesiologists believe that the drug should be used only when indicated in children.
The action of succinylcholine is prolonged in patients with abnormal or deficient plasma cholinesterase and in those with chronic neuromuscular disease. Severe liver failure results in low levels of plasma cholinesterase. Succinylcholine may be safely used as a continuous-infusion relaxant for short procedures, with constant assessment of its effect with a twitch monitor. Large doses of the drug cause a phase II (or desensitization) block, a prolongation of effect that cannot reliably be reversed pharmacologically and must be allowed to wear off gradually. Monitoring the twitch shows the onset of signs of a nondepolarizing blockade (fade on tetanic stimulation and posttetanic facilitation) when this phase II block develops.
Nondepolarizing agents are used for prolonged relaxation during the surgical procedure. They can be used for endotracheal intubation, but they take longer than succinylcholine to complete relaxation of the jaw (approximately 2 minutes) when twice the ED95 (dose required to block 95% of receptors) is administered. The nondepolarizing agents that are currently in use and their doses, durations, and side effects are listed in Table 1-11.
The cardiovascular effects of nondepolarizing relaxants are the result of ganglionic blockade, vagal stimulation, and histamine release. Ganglionic blockade and histamine release result in hypotension, whereas vagal blockade results in tachycardia. The way in which drugs are metabolized and eliminated determines which drugs are used for patients with hepatic, renal, or some metabolic dysfunctions (Table 1-12). Most nondepolarizing relaxants are partially metabolized in the liver. The rest are excreted unchanged in urine or bile.
Most anesthesiologists monitor the twitch response when using neuromuscular-blocking agents because the concurrent administration of inhalational agents potentiates their effect, and many physiologic factors may affect their excretion and metabolism. Although relaxation may be antagonized by the administration of neostigmine, pyridostigmine, or edrophonium, this effect is not reliable when doses of nondepolarizing relaxants that are sufficient to block all twitch responses at the time of administration are used.
Airway Maintenance
The patient’s airway must be maintained when a general anesthetic is being administered. As soon as the patient loses consciousness, the upper airway structures relax and the tongue falls backward, obstructing the glottis. This obstruction is corrected by pulling the lower jaw upward and forward, hyperextending the neck, and inserting an oropharyngeal airway. However, if an oropharyngeal airway is inserted before the pharyngeal and laryngeal reflexes are obtunded, laryngospasm may occur. Obese patients and those with a short, muscular neck tend to obstruct easier and earlier during induction. If the patient is not anesthetized deeply enough for the mouth to be opened, a nasopharyngeal airway may be inserted.
It is critical to select the right size of oropharyngeal and nasopharyngeal airways. One may estimate the correctsized oropharyngeal airway by placing it against the side of the face, the flange (wide opening) at the lips. The tip of the airway should reach the angle of the jaw. If the airway is too large, it will not fit. If it is too small, it will cause obstruction by delivering the gas mixture to the soft tissue of the tongue. The proper length of the nasopharyngeal airway is from the nares to the tragus of the ear. If it is too long, it may damage pharyngeal structures or cause vagal stimulation resulting in bradycardia.
If the patient’s airway cannot be maintained with a mask, or if a long period of airway control is anticipated, a laryngeal mask airway or endotracheal intubation becomes necessary. The laryngeal mask airway is a recent innovation and provides a conduit for inspired gas directly to the supraglottic area (Fig. 1-1). However, it does not provide a reliable seal to protect from aspiration of saliva or gastric contents. It also does not provide a tight seal for controlled ventilation, because it is not in the trachea. The great benefit of this device is that it does provide an open airway in the vast majority of cases without requiring perfect positioning, and it may also be used as a conduit for endotracheal intubation in most patients if other methods have not been successful. Generally, if the patient requires help with ventilation, an endotracheal tube is required. Muscle relaxants facilitate intubation. However, because they inhibit spontaneous ventilation, they should not be given until the anesthesiologist can manually ventilate the patient through a mask. If mask ventilation is impossible, the depth of anesthesia must be decreased and the endotracheal tube must be inserted with the patient awake and breathing spontaneously. Intubation is facilitated by the use of a laryngoscope. Figure 1-2 shows a laryngoscope with a curved, Macintosh-3 blade. Batteries in the handle of the laryngoscope power a small light bulb on the side of the blade. The operator holds the handle in the left hand and opens the patient’s mouth with the right hand. The blade is inserted from the right side of the mouth and slides back along the right margin of the tongue to the vallecula (the area between the base of the tongue and the epiglottis). With the blade in the proper position and the patient’s neck slightly extended in the sniffing position, lifting the handle raises the epiglottis and brings the vocal cords into direct vision (Fig. 1-3). Figure 1-4 shows the difference between the curved and the straight blades in various sizes. In all patients except newborns, the straight blade is placed over the epiglottis to lift it, along with the base of the tongue, to expose the larynx. Another device being used with increasing frequency, especially in the case of difficulty visualizing the glottis with the laryngoscope, is the fiberoptic laryngoscope. This device is similar to the fiberoptic bronchoscope but is smaller in diameter to allow the endotracheal tube to be inserted over it. The tube is placed over the bronchoscope and held at its top while the glottis is visualized and the scope is advanced into the trachea. The tube is then advanced over the bronchoscope while the operator continues to visualize the carina. This intubation method obviously requires considerable practice, but saves many patients from undergoing an emergency surgical airway (tracheostomy) when difficulty is encountered with routine airway management.
Figure 1-1 A battery-operated laryngoscope with a 3-Macintosh blade.
Figure 1-2 A laryngeal mask airway in place
Figure 1-3 The position of the (A) curved blade and (B) straight blade in exposing the larynx.
Figure 1-4 Curved and straight laryngoscope blades of various sizes. Left to right: 1-1/2-Wis-Hipple and 1-Macintosh blades for small children; 3-Miller and 3-Macintosh blades for most intubations; 4-Miller and 4-Macintosh blades for very large adults.
In general, endotracheal intubation is required whenever the anesthesiologist does not have immediate access to the airway, when mechanical ventilation is required because of muscle relaxation or physical characteristics of the patient, or when there is risk of aspiration. Intubation of the trachea in a poorly anesthetized patient results in massive autonomic stimulation, which is seen as hypertension, tachycardia or bradycardia, arrhythmias, and bronchoconstriction. Endotracheal intubation never guarantees an adequate airway. Secretions may obstruct the tube, it may kink, it may become displaced either out of the trachea or down into a bronchus, or the patient may aspirate pharyngeal contents despite the presence of an inflated cuff. To minimize the risk of aspiration of gastric contents, patients who are suspected of having a full stomach can be intubated either awake under topical anesthesia or after being anesthetized with a rapid sequence (or crash) induction. In a rapid sequence induction, the patient is preoxygenated until the expired oxygen concentration approaches 100%. Then anesthesia is rapidly induced with the induction agent and muscle relaxant while an assistant provides downward pressure on the cricoid cartilage of the neck. This pressure is applied to compress the lumen of the esophagus between the posterior ring of the cartilage and the vertebral body. It prevents passive regurgitation of gastric contents through the esophagus. To avoid pushing air into the stomach and increasing gastric pressure, the anesthesiologist intubates the trachea without ventilating the patient with the mask. When the position of the tube in the trachea is verified and the cuff is inflated, the assistant releases the cricoid pressure. Indications and complications of endotracheal intubation for general anesthesia are listed in Table 1-13.
If the uvula and tonsillar pillars are easily visible when the patient opens the mouth and sticks out the tongue, then the anesthesiologist can safely assume that intubation will be possible with direct laryngoscopy. A difficult intubation may be anticipated in patients who have a short, muscular “bull” neck, a receding chin, protruding upper incisors, or a high-arched palate in a narrow mouth. Inability to open the mouth wide or to extend the neck also predicts difficult laryngoscopy. Finally, any space-occupying lesion of the mouth, pharynx, larynx, or neck makes direct laryngoscopy difficult. In these cases, conventional induction and intubation may not be appropriate. Alternative methods include awake intubation under direct vision with topical anesthesia, awake blind nasal intubation, intubation over a fiberoptic bronchoscope inserted into the trachea with the patient either awake or asleep, and tracheotomy under local anesthesia.
Emergence
A patient who is not critically ill should be returning to a normal state when leaving the operating room. In this condition, respiratory obstruction, pulmonary aspiration, and cardiovascular instability are unlikely. Recovery from anesthesia begins when anesthetic drugs are discontinued. Oxygen at 100% is administered while the anesthetic gases are being excreted through the lungs. The patient’s alveolar ventilation, the lipid solubility of the agent, and the duration of the anesthetic determine how quickly gases are eliminated. An agent that is highly lipid-soluble tends to remain sequestered in body fat, dissolving slowly and providing a long period of low blood concentration as it dissolves. The recovery rate from intravenous anesthetics depends on the amount administered, time of last injection, lipid solubility, hepatic metabolism, and renal excretion. If muscle relaxants are used, the degree of remaining blockade is monitored with a nerve stimulator. If necessary, blockade effects are neutralized by neuromuscular antagonists. All monitoring continues until the patient leaves the operating room; it begins again in the recovery room. In unstable patients, monitoring continues during transportation. Because hypoxemia is documented consistently in the immediate postanesthesia period, oxygen is administered as the patient is transported to the recovery room.
Extubation of the Trachea
Patients are safely extubated when the criteria in Table 1-14 are met. Before extubation, the patient breathes 100% oxygen until the expired oxygen concentration is nearly 100% and the oropharynx is suctioned. The endotracheal tube may be suctioned if necessary. The cuff is deflated to allow easy removal of the endotracheal tube. Complications that may follow extubation postanesthesia include laryngospasm, aspiration, pharyngitis or laryngitis, and vocal cord dysfunction. The most common and most serious is laryngospasm, which occurs when the vocal cords are irritated. Laryngospasm is treated by administering positive pressure oxygen by mask and by maintaining an unobstructed airway. Occasionally, a small amount of muscle relaxant is necessary to relax the vocal cords sufficiently to allow air to enter. The patient’s respirations must be controlled until the relaxant wears off.
Regional Anesthesia
Regional anesthesia provides loss of pain perception in the area of surgical intervention. The patient usually remains awake and can breathe adequately. Monitoring and observation in the operating room and recovery room are identical to those for general anesthesia. The most common regional anesthetics are those of the lower spinal nerve roots (spinal, epidural, and caudal blocks) and the brachial plexus.
Figure 1-5 shows the lower spinal canal and the different needle placements for subarachnoid (spinal), epidural, and caudal blocks. Each approach provides adequate anesthesia and operating conditions for surgical procedures below the waist. If the procedure will be performed on the upper abdomen, or if extensive manipulation of the viscera is required, adequate analgesia may be achieved only with the addition of a celiac plexus block (to denervate the viscera). Combined techniques with regional and general anesthesia recently became popular, especially in small children. The regional block is designed to provide analgesia into the postoperative period.
Figure 1-5 The lumbosacral spinal canal showing needle placement for subarachnoid, epidural, and caudal blocks. The spinal cord terminates at approximately L2, and the dural sac terminates at approximately S2. Below S2, the sacral canal contains only the dura-enclosed sacral nerve roots.
Spinal Anesthesia
Spinal anesthesia is an ideal technique for surgery on the lower part of the body. When a small amount of local anesthetic is injected into the subarachnoid space at a level below L2, it diffuses through the cerebrospinal fluid (CSF) to a height determined by the specific gravity and concentration of the anesthetic solution and the position of the patient. After the drug is injected, an almost immediate sympathetic blockade occurs, which results in vasodilation, pooling of blood in dependent areas, and decreased cardiac output. This sympathetic effect is best treated by administering fluids and raising the patient’s legs to increase venous return. The Trendelenburg position is contraindicated soon after a spinal injection. It elevates the level of block to higher than desired in the case of a hyperbaric (specific gravity greater than that of CSF) anesthetic solution and to lower than expected in the case of a hypobaric (specific gravity less than that of CSF) solution. If the level of sympathetic blockade is higher than T1 to T4, the cardioaccelerator sympathetic nerves are blocked and bradycardia ensues.
During a spinal anesthetic, the sympathetic blockade is several levels higher than the sensory blockade, and the motor blockade is several levels lower than the sensory level. If the level of motor blockade is higher than C3, the diaphragm and intercostal muscles are paralyzed, and the patient cannot breathe adequately. Apnea may also occur secondary to ischemia of the medullary centers as a result of profound hypotension induced by the anesthesia. If respiration is compromised, the patient is intubated and ventilated until the level recedes enough for spontaneous respiration to resume. The anesthesiologist must ensure that the patient who is receiving a spinal anesthetic is fully monitored during and after the block. A reliable intravenous line must be running, oxygen must be available, and all anesthetic drugs and equipment must be within arm’s reach as the block is being performed. Patient preparation should be as complete for a regional technique as for a general anesthetic (i.e., the patient should be in optimal medical condition, have an empty stomach, and be psychologically prepared).
Headache, the most common complication of a spinal anesthetic, once occurred in approximately 25% of cases, mostly among younger patients. It is more common when anesthetic is continuously administered, a technique involving insertion of a small catheter into the subarachnoid space for repeated injections. Headache usually begins the day after the spinal and is related to posture, occurring when the patient is upright and disappearing when the patient lies down. The headache is caused by leakage of CSF through the dural puncture. Using a 25- or 27-gauge needle for the puncture reduces the probability of spinal headache to 5% to 10% in the most susceptible population. Use of a “pencil point” needle further reduces the incidence to 2%. Hydration and bed rest are usually adequate treatment, but if the headache is prolonged (1–2 days), it is treated by injecting 5 to 10 mL of the patient’s own blood into the epidural space at the level of the previous spinal injection (epidural blood patch).
Epidural Anesthesia
When anesthesia is needed in the lower half of the body, epidural anesthesia is another option. The epidural space is the area within the spinal canal outside the dura and arachnoid. In the area below L2, it contains fat, an extensive venous plexus, and the dura-enclosed cauda equina. A large volume of local anesthetic is injected into the space. The level of the block depends on the actual volume injected. Continuous analgesia is obtained by giving additional doses of the anesthetic through a catheter inserted through the needle at the time the epidural space is identified. The concentration of anesthetic determines whether the block is sympathetic, sensory, or complete (i.e., including motor fibers).
The onset of epidural anesthesia is slower than that of spinal anesthesia. However, the level and characteristics of the block are much more controllable because anesthetic can be added in small increments through the indwelling catheter placed in the epidural space. Because of anatomic abnormalities that may be present in the space, a “patchy” block is much more likely to result from an epidural than from a spinal block. Hypotension and respiratory insufficiency may develop, but less frequently than with a spinal anesthetic because of the more gradual onset of blockade. The needle used in an epidural block is larger than that used in a spinal block. For this reason, if the dura is accidentally punctured during its insertion, a spinal headache is more likely to ensue. Backache also is more likely after an epidural block because tissues are more likely to be injured when the larger, blunter needle is inserted through the ligaments that support the spinal column. Anesthetic unknowingly injected into the subarachnoid space because of unrecognized dural puncture results in a total spinal, with total blockade of all of the spinal nerves. If the anesthetic reaches the level of the brain stem, unconsciousness and apnea occur. If the anesthetic is injected into one of the numerous epidural veins, systemic toxicity occurs. These complications are rare and epidural anesthesia is the gold standard for many lower extremity, pelvic, and lower abdominal surgeries, as well as for analgesia during labor and delivery.
Caudal Anesthesia
The caudal block is a variation of the epidural block, in which the needle is inserted through the sacral hiatus at the bottom of the sacrum and anesthetic is injected into the epidural space. Larger volumes of anesthetic are required to achieve the same level of analgesia with this route, but there is virtually no possibility of an unrecognized subarachnoid injection. Because of anatomic variations that are present in a large percentage of the population, however, reliable anesthesia is often difficult to achieve with this technique. For this reason, it is used less often than the epidural block, except in small children, in whom the anatomy is less variable.
Brachial Plexus Blocks
The brachial plexus may be blocked in the interscalene area, supraclavicular area, or axilla. Any of these approaches results in reliable anesthesia of the forearm and hand. Anesthesia of the upper arm with a brachial plexus block is less reliable. The plexus is identified with a needle by eliciting paresthesia or by motor response to stimulation by a nerve stimulator attached to the needle. A relatively large volume (35–50 mL) of local anesthetic, usually 1.5% to 2% lidocaine, 0.5% bupivacaine, 1.5% mepivacaine, or 2% chloroprocaine, is injected when the plexus is located. Anesthesia of all of the nerves in the plexus depends on the volume and concentration injected. Complications of brachial plexus blocks are related to the volume of anesthetic or the proximity of the injection site to the carotid or axillary artery, the dome of the pleura, and the spinal canal.
Intravenous Regional Block (Bier Block)
When the surgical procedure can be performed with a tourniquet on the extremity, a Bier block provides reliable anesthesia of an arm or leg. The block is achieved by exsanguinating the extremity with an elastic compression bandage, inflating a proximal tourniquet above arterial pressure, and replacing the blood volume of the extremity with a dilute solution of local anesthetic (usually 0.5% lidocaine). Complete blockade occurs almost immediately and continues until the tourniquet is released. If a second tourniquet is placed just distal to the first and inflated after the block is effective, the proximal tourniquet may be deflated and tourniquet pain (ischemic pain under the tourniquet) is avoided. If the tourniquet is not inflated to a sufficiently high pressure, or if it is released or leaks during the procedure, the block fades as anesthetic escapes into the circulation. If this escape occurs within half an hour of the injection, systemic toxicity may occur because of the large load of anesthetic that may be released. At the end of the procedure, the tourniquet is released and the block diminishes rapidly. If the procedure lasted less than half an hour, the tourniquet is released slowly and intermittently to minimize systemic blood levels of local anesthetic.
Nerve Blocks and Local Infiltration Anesthesia
Nerve Blocks
Injections of local anesthetic are used to provide adequate analgesia for limited surgery. The following peripheral nerves are easily blocked: digital for finger or toe surgery; intercostal for thoracic or abdominal wall procedures; sciatic, obturator, and femoral for lower leg procedures; mandibular for lower jaw procedures; retrobulbar for eye procedures; penile for circumcisions; and ankle for foot procedures. Nerve blocks are effective for distal procedures that include skin, subcutaneous tissue, muscle, fascia, bone, joints, and periosteum. Peritoneum, pleura, and pericardium cannot be rendered insensitive by peripheral nerve block because they have both autonomic and somatic innervation. An epinephrine local anesthetic solution should not be used for nerve blocks on the digits or penis because epinephrine can cause local vasoconstriction that leads to peripheral ischemia. The only contraindications to nerve blocks for appropriate procedures are unacceptability to the patient, local infection at the injection site, coagulopathy, and allergy to both kinds of local anesthetic.
Local Anesthesia
Local anesthesia is achieved by infiltrating a local anesthetic into the skin and underlying tissue around the operative site. Local infiltration of anesthetic is sufficient for limited superficial procedures that involve skin, subcutaneous tissue, muscle, and fascia. It is difficult to desensitize bone and periosteum with local infiltration. Preemptive analgesia by presurgical infiltration of the incision area reduces postoperative pain and suffering. The same contraindications as for nerve blocks apply.
Local anesthetics may be administered by several routes: topically (directly on the skin, cornea, or mucous membrane), by infiltration around a peripheral nerve or plexus, in the subarachnoid space, in the epidural space, or even intravenously. To provide a drier surgical field and to prolong the block, epinephrine is added to the local anesthetic. Because virtually every practitioner uses local anesthetics to some extent, a working knowledge of their pharmacology and toxicity is important. All local anesthetics except cocaine consist of a tertiary amine and an unsaturated aromatic ring separated by either an ester or an amide link. The benzene ring imparts lipid solubility that is necessary to permeate nerve sheaths and coverings. The amine imparts water solubility that is necessary to diffuse and bind to the axonal site of action. The amide or ester link determines the route and speed of drug metabolism: those with an ester linkage are metabolized rapidly by serum cholinesterase and acetylcholinesterase; those with an amide linkage are metabolized more slowly by the hepatic microsomal system. Table 1-15 lists local anesthetics that are currently in use, the ways in which they are used, and their important characteristics.
Local anesthetics are classified according to their duration of action. The drugs in each category have similar toxicities and potencies. Adding epinephrine or another vasoconstrictor to the injected solution usually prolongs the duration of action by one third to one half and reduces the toxicity of the drug secondary to retarding its rapid vascular uptake. Because epinephrine causes vasoconstriction, it is not used in areas that are prone to ischemia (e.g., nostril, external ear, digits, penis).
True allergic reactions to local anesthetic agents, especially those with an amide linkage, are rare but life threatening. A patient who has a history of allergy to local anesthesia should undergo skin testing with several agents to determine whether they are truly contraindicated. Although it is not 100% reliable, skin testing, which involves applying an intradermal patch and looking for wheal and flare, is accepted as adequate screening for local anesthetic allergy. True allergy to lidocaine and other amides has been reported only a few times in millions of administrations. Distinguishing an allergic reaction from systemic toxicity is difficult because most reactions to local anesthetics are the result of accidental intravascular injections or overdoses rather than true allergies. The maximum recommended doses of commonly used local anesthetic agents are shown in Table 1-15. Systemic toxicity is the greatest potential risk associated with their use. Symptoms include ringing in the ears, perioral numbness, and a metallic taste in the mouth. These symptoms signal the onset of CNS excitation that may then progress to jitteriness, shivering, convulsions, and coma. Treatment of local anesthetic toxicity consists of oxygenation and injection of anticonvulsants, preferably short-acting intravenous agents (e.g., thiopental, diazepam, midazolam). Prevention is easily accomplished by calculating the dose to be administered, ensuring that the agent is not injected into a vessel by aspirating frequently during injection, and premedicating with a benzodiazepine to reduce CNS toxicity whenever it is anticipated that large doses of local anesthetics will be used.
All local anesthetics (with the exception of cocaine) cause myocardial depression, peripheral vasodilation, and depression of autonomic responses in a dose-related fashion. Lidocaine is associated with a short-lived blockade of the myocardial conduction system. Bupivacaine causes a more prolonged blockade associated with malignant reentrant dysrhythmia, which is difficult to reverse and occurs at blood levels only slightly above those that cause CNS excitement. Emergency lifesaving support may be necessary, including prolonged cardiopulmonary resuscitation, bretylium rather than lidocaine for ventricular ectopy, and, often, large doses of epinephrine. If convulsions also occur after bupivacaine injection, they are controlled with a short-acting muscle relaxant (e.g., succinylcholine). Anti-convulsants are contraindicated because they may cause further cardiac depression.
Cocaine is different from the other local anesthetics because it blocks the presynaptic uptake of norepinephrine into sympathetic nerve terminals. For this reason, it has local vasoconstrictive effects that make it useful as topical anesthesia for surgery of the nose and throat. Cocaine also has profound CNS effects, similar to those of antidepressants or amphetamines, accompanied by cardiovascular stimulation. It is associated with coronary arterial vasospasm. The drug fell out of favor with many anesthesiologists because of the difficulty in keeping it “off the street,” and because the same effects can be achieved with a combination of other topical anesthetics and vasoconstrictors (e.g., phenylephrine).
Anesthetic Supplements
Opioids
Opioids are used to supplement local or regional anesthesia, to supplement inhalational anesthetics so that lower concentrations may be used, and with other drugs as a part of total intravenous anesthesia. Fentanyl, sufentanil, and alfentanil are often given as a continuous intravenous infusion or in small, frequent doses after an initial bolus dose. Remifentanil is usually given as a continuous infusion. The longer-acting opioids are given as incremental small doses during the anesthetic to establish a baseline narcosis that continues through the immediate postoperative period. With the exception of remifentanil, which is metabolized in plasma by esterases, opioids are all metabolized by the liver and the metabolites are excreted through the kidneys. Table 1-16 characterizes the opioid receptors and identifies their commonly used agonists and antagonists. Table 1-17 shows the opioids that are used intravenously in the operating room and their relative potencies, onset times, and elimination half-lives.
The CNS effects of opioids include analgesia, euphoria, drowsiness, miosis, depression of the cough reflex, nausea, and vomiting. Analgesia is caused by a reduction in the transmission of noxious stimuli at the peripheral, spinal, and central levels. Nausea and vomiting are caused by stimulation of both the vestibular system and the central chemoreceptor trigger zone in the medulla. High doses of opioids cause drowsiness and sedation, but do not reliably cause unconsciousness, so anesthesia must include other agents.
Cardiovascular effects include bradycardia that is caused by stimulation of vagal nuclei in the medulla and is easily treated with atropine. Meperidine may cause tachycardia in large intravenous doses, presumably because its chemical structure is similar to that of atropine. Only meperidine causes myocardial depression in clinically useful doses, which can be as low as 2 to 2.5 mg/kg. Morphine and meperidine stimulate the release of histamine in clinical doses, resulting in arteriolar and venous dilation and hypotension. Because fentanyl, sufentanil, alfentanil, and remifentanil do not show this effect, these narcotics are most often used in high doses during anesthesia.
All opioid agonists produce a dose-dependent respiratory depression that causes an equivalent right shift of the CO2 response curve, but does not alter its slope (Fig. 1-6). The effect is equal for all drugs at equianalgesic doses. Lower doses decrease the respiratory rate without changing the tidal volume, but at higher doses, the tidal volume is also smaller. Pontine and medullary centers are depressed at still higher doses and breathing becomes irregular, with eventual apnea ensuing in the unstimulated patient.
Figure 1-6 The CO2 response curve is shifted to the right after opioid administration. (Modified from Bailey PR, Stanley TH. Narcotic intravenous anesthetics. In Miller RD, ed. Anesthesia. 3rd ed. New York: Churchill Livingstone; 1990.)
Contrary to previous belief, opioids alone do not affect renal function or antidiuretic hormone secretion. Their effects on the liver are limited to contraction of the sphincter of Oddi and an increase in pressure in the biliary tree. Opioids decrease the motility of the gastrointestinal tract, causing ileus, delayed gastric emptying, and constipation.
All agonists can cause skeletal muscle rigidity when given in high intravenous doses, but only fentanyl, sufentanil, and alfentanil are given in sufficiently large boluses for this effect to be clinically significant. Large individual variation in this effect is seen; it can occur with doses as small as 1 mg/kg fentanyl. Rigidity is not caused by an action at the neuromuscular junction, but rather by a central effect. Nonetheless, sometimes a patient who has truncal rigidity after receiving intravenous narcotics cannot be ventilated until a muscle relaxant is administered. Muscle rigidity rarely occurs when opioids are given after an inhalation anesthetic is started.
In general, the narcotics used in the perioperative period are safe. The most common problems are respiratory depression and resultant hypoxemia. Hypotension is seen mainly with morphine because of its histamine-releasing effects or in severely hypovolemic patients. There is no evidence of teratogenicity, organ toxicity, or other delayed effects. Overdose is easily reversed with antagonists, although these drugs have serious side effects of their own (see later). Allergic reactions are rare, and they usually cause urticaria rather than anaphylaxis. Because they stimulate histamine release, morphine and meperidine may cause local wheal and flare formation at and near the site of injection. Meperidine is contraindicated in patients who are taking monoamine oxidase (MAO) inhibitors; tachycardia and hypertension can lead to death with this combination of agents.
Opioid Antagonists
Naloxone and naltrexone are competitive antagonists at the m, k, and S receptors for all opioid effects, including analgesia. Naltrexone, a long-acting antagonist used only in an oral preparation, has no use in the perioperative period. Naloxone is used to reverse narcotic-induced ventilatory depression and sedation in the immediate postoperative period and to treat unwanted side effects of epidural, subarachnoid, or continuous-infusion narcotics. Unfortunately, doses that rapidly reverse ventilatory depression may lead to such rapid onset of pain that the accompanying sympathetic stimulation causes tachycardia, hypertension, and CNS excitement. Doses of 0.1 to 0.4 mg naloxone are associated with atrial and ventricular dysrhythmia, pulmonary edema, cardiac arrest, and convulsions. To balance the desired effect of analgesia with the undesirable effect of respiratory depression, ventilation is supported while reversal is titrated with small, incremental doses of naloxone. In this way, a balance can be achieved between analgesia and acceptable respiration. The effect of naloxone lasts only approximately 30 minutes, so repeated doses or continuous infusion may be necessary. The best course is to tailor narcotic administration to avoid respiratory depression.
Sedation
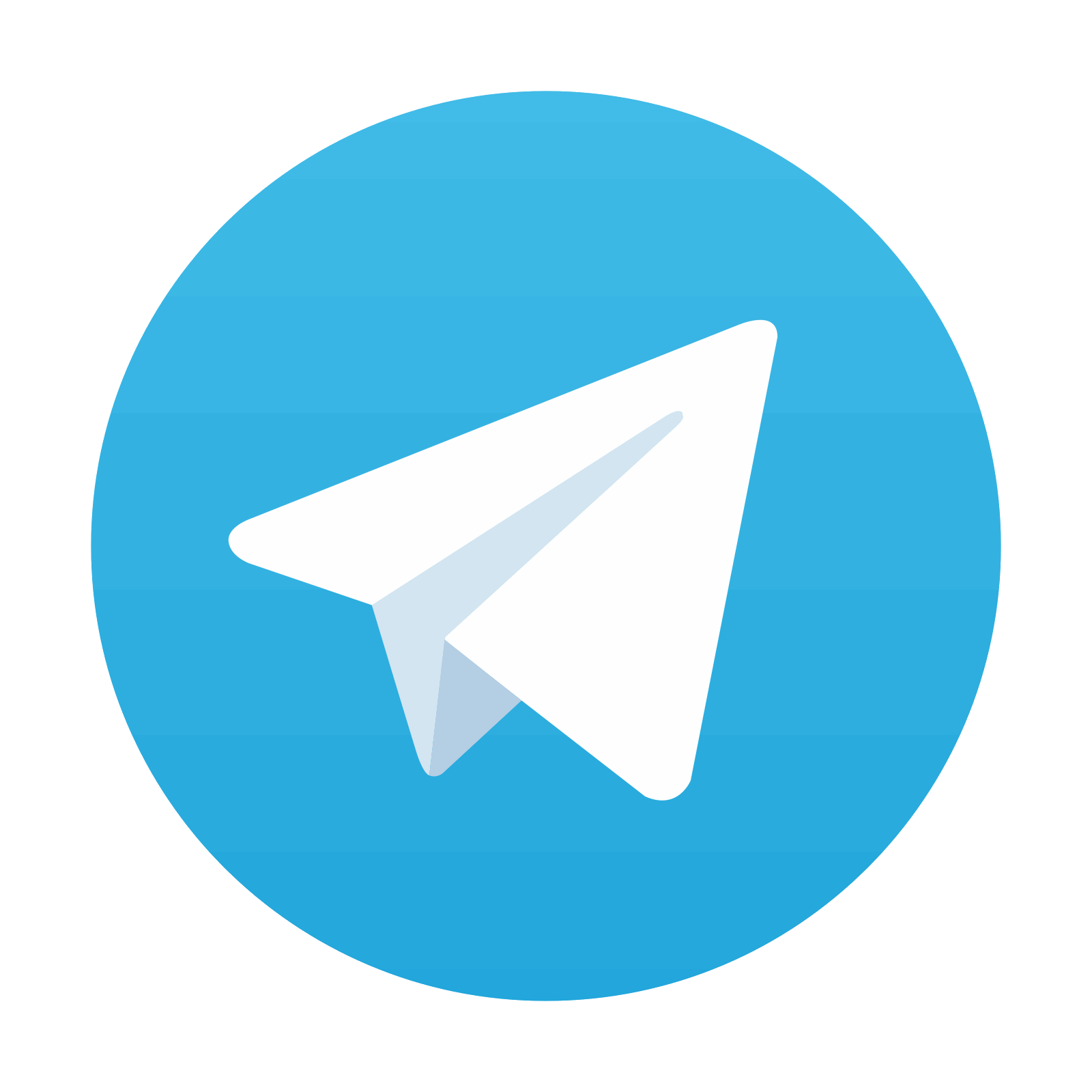
Stay updated, free articles. Join our Telegram channel

Full access? Get Clinical Tree
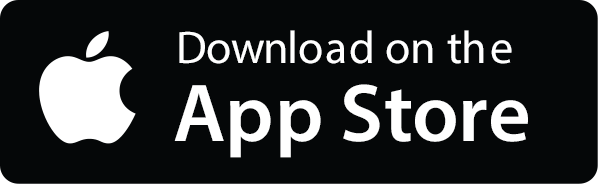
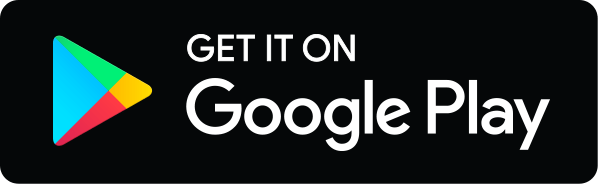