12 Alexander E. Ivanov Protista Biotechnology AB Sweden Polymeric materials and macromolecular constructs with chemically attached phenylboronic acids (PBAs) have gained an increasing attention during the last decade. These materials and constructs range from bulky hydrogels and water-soluble synthetic polymers to the finest nanoparticles, micelles and nanoscopic polymer brushes capable of reversible binding to polyols, sugars, polysaccharides, glycoproteins and biological cells via formation of phenylboronate esters [1]. The reaction takes place in aqueous media, often close to physiology conditions. Boronate-containing polymers (BCPs) have, therefore, been widely studied and used for sugar sensing [2–5] and sugar-responsive drug delivery [6–10], assays of pharmaceuticals [11,12], separation of glycoproteins [13–15] and cells [16], encapsulation of animal cells [17,18] and, recently, for gene transfection [19] and detection of metastatic cell phenotypes [20]. Biomedical applications of BCPs have previously been reviewed [21]. BCPs and other complex PBAs are sometimes called ‘boronolectins’ [22] due to their ability to bind to mono- and oligosaccharides. In some cases, such as isolation of glycoproteins from human serum, a similarity between the compositions of glycan mixtures obtained using BCPs and lectins as immobilized affinity ligands was quite evident [14]. Since lectins were successfully used to enhance the mucoadhesivity of microparticles, liposomes and microdevices [23–25], employment of BCPs as sugar-specific mucoadhesive agents seems also promising. Both the mucus gel and epithelial glycocalix contain many mucins or mucin-like glycoproteins exhibiting numerous oligosaccharides [26] that can be targeted by the boronate groups of adhering polymers. Incorporation of BCPs into macrodosages and tissue sealants may have both advantages and disadvantages compared to lectins. Firstly, lectins exhibit much higher selectivity to the particular end-group saccharides compared to BCPs. At the same time, this selectivity cannot be fully realized because the mucosal surfaces contain different cell types, which, as well as the insoluble layer of mucus, exhibit many different O-glycans besides those complementary to the particular lectin. Since the contact area of a macrodosage with mucosa is much larger than the surface of a particular cell, some of the lectin molecules will not be involved in the interaction with their carbohydrate counterparts. Secondly, BCPs may have several tens of phenylboronic acid groups per polymer chain, whereas the lectins have a lower number of binding sites, for example four in concanavalin A [27]. On the other hand, lectins recognize the pyranose forms of monosaccharides, including the end groups of oligosaccharides typical of mucosal surfaces, whereas boric and boronic acids preferably interact with the furanose forms of free monosaccharides [28,29]. Despite these differences, spontaneous binding of BCPs to polysaccharides [1] and mucin [30] in aqueous solution has been proven experimentally and some mucoadhesive materials have been developed (Section 12.6). Lastly, but not least, the high cost of lectins is a limiting factor for their applications at macrodosage scales, whereas synthetic BCPs may happen to be less expensive. Until now, BCPs and low molecular weight borate were rarely studied and used as mucoadhesive reagents. This might be a consequence of limitations imposed by the optimal conditions for boronate–sugar interactions (the pH higher than the physiological pH, Section 12.2) and difficulties with the synthesis of biocompatible water-soluble BCP. Some of these limitations can be partially overcome by using phenylboronic acids with lower pKa [7,19] and the development of new synthetic approaches for incorporation of boronic acids into biocompatible and biodegradable polymers [31]. To understand better the possibilities offered by BCPs to the molecular design of mucoadhesive materials, the fundamentals of boronate interactions with sugars, including oligosaccharides of mucins, are briefly considered. It is generally accepted that the interactive forms of boric and phenylboronic acids (PBA) are their anions, which have a tetrahedral configuration of the hydroxyl groups around the boron atom (Figure 12.1) [32]. The formation of cyclic esters in an aqueous medium is accompanied by a rise in the acidity because the Figure 12.1 Equilibrium of the pendent PBA groups between an uncharged trigonal form and a charged tetrahedral form. The charged form interacts with a monosaccharide adopting a furanose configuration with vicinal cis-diols. The other pendent groups of the copolymer are not shown. Due to the different configurations of diols and triols, monosaccharides vary strongly in their binding strength to boronic acids and, respectively, to BCPs. The association constants of some sugars and polyos with borate and phenylboronate are listed in Table 12.1. Table 12.1 Increase in phase transition temperature of NIPAM-co-NAAPBA in the presence of 0.56 mM sugars and polyols (ΔTP) and the association constants of borate and phenylboronate with sugars and polyols (Log Kass). Reprinted with permission from [36], Copyright (2006) American Chemical Society. Obviously, BCPs with many PBA groups can bind several sugar molecules simultaneously. The reagents convenient for evaluation of the binding affinity of sugars and glycoproteins to immobilized PBAs are thermally responsive copolymers of N-isopropylacrylamide and N-acryloyl-m-aminophenylboronic acid (NIPAM-co-NAAPBA) [36]. The phase transition temperature of the copolymer shifts to the higher values due to the formation of charged PBA–sugar esters, whereas the shift grows proportionally to the fraction of sugar-associated PBA groups [37]. The temperature shifts obtained with various sugars are listed in Table 12.1. The interactions of PBA with oligosaccharides of glycoproteins and with alkyl glycosides are essentially different from the interactions with monosaccharides capable of interconverting between their α- and β-forms as well as pyranose and furanose forms, due to reversible opening and closure of the saccharide ring. The most stable cyclic boronic acid esters are formed between the boron atom and vicinal cis-diols on furanose rings. The saccharides able to adopt the furanose configuration (D-fructose, D-ribose and its derivatives, D-galactose, [Figure 12.1] and others) form stable complexes with both borate and boronates [28,29]. Alternatively, the sugar moieties connected via glycosidic bonds to the neighbouring sugars, amino acids or alkyl radicals are unable to undergo the ring opening. Moreover, they exist in pyranose forms, where configuration of hydroxyl groups is less favourable for binding to borate or boronate. This is the reason why disaccharides, such as maltose consisting of two glucose molecules or lactose consisting of glucose and galactose molecules, form weaker complexes with borate than the individual sugars [38]. However, in some special cases, such as ‘addition of galactose to saccharose, to form raffinose’, the complex stability increases [38]. Similarly, the disaccharide N-acetyllactosamine, consisting of the units of galactose and N-acetylglucosamine, affected the thermoprecipitation of NIPAM-co-NAAPBA in a manner similar to free galactose, whereas N-acetylglucosamine itself provided a very weak effect (Table 12.1, [36]). The residues of galactose located on the nonreducing ends of oligosaccharides may, therefore, be considered as probable binding sites for BCPs. Additional evidence for this was given by the reversible interaction between galactomannan and borate [39]. The saccharide most often considered as a target for boronate reagents in glycoproteins [19,20,35] is Neu5Ac. It interacts with PBAs via 8- and 9-hydroxylic groups in the glycerol chain at pH > 8, and via α–hydroxycarboxylate moiety at pH 2–8 [35]. Since Neu5Ac is bound to oligosaccharides via its 2-hydroxy group, the interaction of sialylated oligosaccharides with PBA taking place at weakly alkaline pH may be expected. The association binding constant of phenylboronate to Neu5Ac was found to be 11 M−1 at pH 7.4 [35], log Kass = 1.04, whereas the binding of N-propionyl-m-aminoPBA [34] was somewhat stronger, log Kass = 1.2–1.3 (Table 12.1). The local association binding constants of borate to 3,4-diols of α-methyl and β–methyl galactopyranosides were found to have values of about 10 M−1 at pH 7 [40]. Obviously, these values are much lower compared to those of sugars and polyols strongly interacting with borate and boronate (Table 12.1). Fructose or mannitol is, therefore, able to displace the end-group glycosides from their complexes with PBAs. The other end-group sugars typical of O-oligosaccharides in mucins are N-acetylgalactosamine, N-acetylglucosamine and L-fucose. The first two compounds form very weak complexes with NIPAM-co-NAAPBA, even in the monosaccharide forms (Table 12.1, [36]), whereas L-fucose, a sugar without a 6-hydroxyl group, interacts with NIPAM-co-NAAPBA somewhat weaker than D-galactose (Table 12.1). In spite of the low values of equilibrium association constants typical for boronate ester formation with end-group or in-chain glycosides, the binding of BCPs containing several tens of PBA groups per macromolecule to agarose carrier was virtually irreversible under conditions where monomeric NAAPBA could bind only in a reversible manner (Figure 12.2) with the equilibrium association constant of about 50 M−1 [41]. Figure 12.2 Reversible binding of monomeric NAAPBA (left) and irreversible binding of NAAPBA-containing polymer (right) to agarose gel. Elution profiles of NAAPBA ( Notice that this association constant was markedly higher than the constants of PBA with Neu5Ac or of borate with methyl glycosides (Section 12.2 and Table 12.1). This could be due to secondary interactions of NAAPBA with agarose, such as hydrogen bonds. Furthermore, if the reactive pendent groups of a macromolecule bind to the complement molecular receptors, the binding constant grows exponentially as a function of number of the binding sites [42]: where K1 and ΔF1 are the equilibrium binding constant and the free energy change for the reaction of one pendent group and n is the number of reacting groups. Therefore, a simultaneous binding of three or four pendent PBA groups to the polysaccharide carrier would result in a binding strength similar or higher than the strengths of lectin–oligosaccharide interactions: Kass = 103–104 M−1 [43]. Interestingly, the BCPs were found to associate with polysaccharides at a pH even below the pKa of the boronate groups: the copolymer of NAAPBA with acrylamide (4 : 96) was able to cross-link galactomannan at pH 7.4 [44], whereas the copolymer of NAAPBA with N,N-dimethylacrylamide (DMAA-co-NAAPBA) (10 : 90) has adsorbed at significant quantities (≥6 mg/ml) on agarose gel at pH 7.9 or higher [41]. The effective pKa of the copolymerized NAAPBA estimated by potentiometric titration was 8.8 in 0.15 M sodium chloride [41]. As followed from the titration curve, the ionization degree α ≈ 0.2 might be expected for the latter copolymer at pH 7.9, and therefore the average quantity of the charged phenylboronates could be estimated as three of about 15 groups existing per macromolecule, for the average molecular weight of 1.9 × 104 g/mol [41]. The charged PBA groups were probably involved in the copolymer binding to agarose gel. A series of BCPs called PLL-g-(PEG;PBA) was prepared by simultaneous attachment of the end-group activated poly(ethylene glycol), PEG (Mw = 5 kDa), and 4-formyl-PBA to polylysine (PLL, Mw = 24 kDa) [45]. The interaction of PLL-g-(PEG;PBA) with the polysaccharide mannan immobilized on agarose beads was studied. Mannose residues in mannans are most often connected by 1–2 or 1–6 bonds. The 2,3- or 4,6-diols of the mannose residues were, therefore, available for interaction with PBA, though at low binding strengths. The chemisorbed amount of the PLL-g-(PEG;PBA) increased with decreasing PEG/lysine ratio. This was ascribed to the steric repulsion between the densely grafted PEG chains and the gel. The BCPs with the low PEG/lysine ratio (1 : 21) and the high number of PBA groups (41 per polylysine chain) adsorbed to the gel at significant quantities (up to 5 mg/ml gel) from 1 mg/ml copolymer solution in 10 mM sodium phosphate buffer, containing 0.14 M NaCl, pH 7.4, [45,46]. The multipoint binding of BCPs to polysaccharides via several weakly interacting pendent groups suggests a possibility of the polymer adhesion to mucosal surfaces containing a large number of cell-surface bound mucin-like glycoproteins as well as the glycoproteins and proteoglycans of extracellular matrix. To investigate this possibility and to find out the optimal conditions for the adhesion, the interaction between pig gastric mucin and several BCPs was studied [30]. DMAA-co-NAAPBA copolymers with the content of boronic monomer from 2.5 to 8.8 mol% were found to form polycomplexes with mucin appearing as fine coacervates in the aqueous solution at pH 8–10 and ionic strengths from 0.01 to 0.2 M [30]. The insoluble particles with hydrodynamic diameter of about 150 nm could be registered as soon as five minutes after the mixing of the reagents and slowly grew in size to 500 nm in 19 h and further to about 750 nm in the next 23 h. The increase in turbidity of the suspension was strongly dependent on the weight proportion of the interacting counterparts. The highest rate of coacervation was registered at the intermediate copolymer:mucin weight ratio of two (Figure 12.3
Boronate-Containing Polymers
12.1 Introduction
12.2 Fundamentals of Borate and Boronate Interactions with Mono- and Oligosaccharides
of the ester is lower than the pKa of the boronic acid itself. Since the pKa of various ring-substituted PBAs range from seven to nine [33], the sugars form esters with PBAs mostly in alkaline and weakly alkaline media. The important exception from this rule is N-acetylneuraminic acid (Neu5Ac, sialic acid), which is able to bind to neutral phenylboronic acids [34,35] in the wide pH range down to pH 4 [34], where other sugars and polyols are not reactive.
Compound
ΔTP
Log Kass
Borate [38]
Phenylboronate [32]
D-Fructose
4.5
2.82
3.6
Lactulose
3.6
2.91
Mannitol
4.0
3.3*
3.4
D-Glucose
4.2
1.80
2.0
L-Arabinose
0.4
2.14
2.6
D-Xylose
0.4
2.2**
D-Galactose
0.4
1.99
2.4
D-Mannose
0.3
2.01
2.2
L-Fucose
0.3
N-Acetylneuraminic acid
2.0
1.04#, 1.3–1.5***
N-Acetylgalactosamine
<0.2
N-Acetylglucosamine
<0.2
Sucrose
<0.2
0.86
Raffinose
<0.2
1.35
Glycerol
<0.2
1.2*
1.3
* taken from Ref. 32; ** taken from Ref. 40; *** calculated from data in Ref. 34, # taken from Ref. 35.
12.3 Multipoint Association of BCPs with Polysaccharides
) and acetone (
) obtained on a Sepharose CL-6B column (1 × 7 cm) in 0.1 M sodium bicarbonate buffer (pH 9.2, 22 °C) (left). The absorbance in the fractions (1 ml) was measured at 265 nm and 280 nm for NAAPBA and acetone, respectively. Elution profile of AA-NAAPBA copolymer (
) under the same conditions (right). The nonboronate pendent groups of the copolymer are not shown. Chromatograms reproduced with permission from [41]. Copyright (2006) WILEY-VCH verlag GmbH & Co., KGaA, Weinheim.
12.4 Formation of Interpolymer Complexes of BCPs with Mucin Glycoprotein
Stay updated, free articles. Join our Telegram channel

Full access? Get Clinical Tree
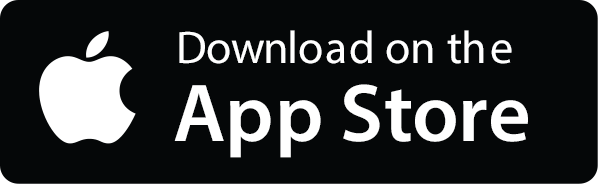
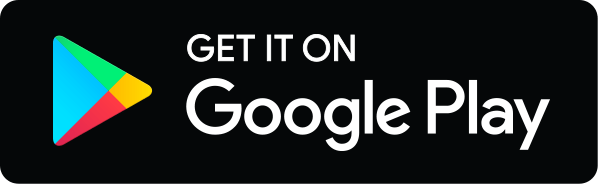