Proteins have many functions in the body. They serve as transporters of hydrophobic compounds in the blood, as cell adhesion molecules that attach cells to each other and to the extracellular matrix, as hormones that carry signals from one group of cells to another, as ion channels through lipid membranes, and as enzymes that increase the rate of biochemical reactions. The unique characteristics of a protein are dictated by its linear sequence of amino acids, termed its primary structure. The primary structure of a protein determines how it can fold and how it interacts with other molecules in the cell to perform its function. The primary structures of all of the diverse human proteins are synthesized from 20 amino acids arranged in a linear sequence determined by the genetic code.
General Properties of Amino Acids. Each of the amino acids used for protein synthesis has the same general structure (Fig. 6.1A). It contains a carboxylic acid group, an amino group attached to the α-carbon in an L-configuration, a hydrogen atom, and a chemical group called a side chain that is different for each amino acid. In solution, at physiologic pH, the free amino acids exist as zwitterions: ions in which the amino group is positively charged and the carboxylate group is negatively charged (see Fig. 6.1B). In proteins, these amino acids are joined into linear polymers called polypeptide chains through peptide bonds between the carboxylic acid group of one amino acid and the amino group of the next amino acid.
FIGURE 6.1 A. General structure of the amino acids found in proteins. B. Dissociation of the α-carboxyl and α-amino groups of amino acids. At physiologic pH (~7), a form in which both the α-carboxyl and α-amino groups are charged predominates. Some amino acids also have ionizable groups on their side chains. pKa, −log of the acid dissociation constant; R, side chain.
Classification of Amino Acids According to Chemical Properties of the Side Chains. The chemical properties of the side chain determine the types of bonds and interactions each amino acid in a polypeptide chain can make with other molecules. Thus, amino acids are often grouped by polarity of the side chain (charged, nonpolar hydrophobic, or uncharged polar) or by structural features (aliphatic, cyclic, or aromatic). The side chains of the nonpolar hydrophobic amino acids (alanine, valine, leucine, isoleucine, phenylalanine, and methionine) cluster together to exclude water in the hydrophobic effect. The uncharged polar amino acids (serine, threonine, tyrosine, asparagine, and glutamine) participate in hydrogen bonding.
Cysteine, which contains a sulfhydryl group, forms disulfide bonds. The negatively charged acidic amino acids (aspartate and glutamate) form ionic (electrostatic) bonds with positively charged molecules, such as the basic amino acids (lysine, arginine, and histidine). The charge on the amino acid at a particular pH is determined by the pKa (−log of the acid dissociation constant) of each group that has a dissociable proton.
Amino Acid Substitutions in the Primary Structure. Mutations in the genetic code result in proteins with an altered primary structure. Mutations resulting in single amino acid substitutions can affect the functioning of a protein or can confer an advantage specific to a tissue or a set of circumstances. Many proteins, such as hemoglobin, exist in the human population as polymorphisms (genetically determined variations in primary structure).
Within the same individual, the primary structure of many proteins varies with the stage of development and is present in fetal and adult isoforms, such as fetal and adult hemoglobin. The primary structure of some proteins, such as creatine kinase, can also vary between tissues (tissue-specific isozymes) or between intracellular locations in the same tissue. Electrophoretic separation of tissue-specific isozymes has been useful in medicine as a means of identifying the tissue site of injury.
THE WAITING ROOM 
Will S. is a 17-year-old boy who presented to the hospital emergency department with severe pain in his lower back, abdomen, and legs, which began after a 2-day history of nausea and vomiting caused by gastroenteritis. He was diagnosed as having sickle cell disease at age 3 years and has been admitted to the hospital on numerous occasions for similar vaso-occlusive sickle cell crises.
On admission, the patient’s hemoglobin level in peripheral venous blood was 7.8 g/dL (reference range, 12 to 16 g/dL). The hematocrit or packed cell volume (the percentage of the total volume of blood made up of red blood cells) was 23.4% (reference range, 41% to 53%). His serum total bilirubin level (a pigment derived from hemoglobin degradation) was 2.3 mg/dL (reference range, 0.2 to 1.0 mg/dL). A radiograph of his abdomen showed radiopaque stones in his gallbladder. With chronic hemolysis (red blood cell destruction), the amount of heme degraded to bilirubin is increased. These stones are the result of the chronic excretion of excessive amounts of bilirubin from the liver into the bile, leading to bilirubinate crystal deposition in the gallbladder lumen.
David K. is a 16-year-old boy who was brought to the hospital by his mother because of the sudden onset of severe pain in his left flank, radiating around his left side toward his pubic area. His urine was reddish-brown in color, and a urinalysis showed the presence of many red blood cells. When his urine was acidified with acetic acid, clusters of flat hexagonal transparent crystals of cystine were noted. There was no family history of kidney stone disease.
Dianne A., who has type 1 diabetes mellitus, has to give herself subcutaneous injections of insulin several times a day to try to mimic what her pancreas would do if she could produce insulin. Insulin was initially purified from animals, and then synthetic human insulin was developed. There is now modified synthetic insulin, which allows the onset of action to be adjusted. She takes long-acting insulin (glargine) once a day, and rapid-acting insulin (lispro) with meals. Her physician will adjust the doses to keep her blood glucose levels controlled.
Anne J. is a 54-year-old woman who is 68 in tall and weighs 198 lb. She has a history of high blood pressure and elevated serum cholesterol levels. Following a heated argument with a neighbor, she experienced a “tight pressure-like band of pain” across her chest, associated with shortness of breath, sweating, and a sense of light-headedness.
After 5 hours of intermittent chest pain, she went to the hospital emergency department, where her electrocardiogram showed changes consistent with acute cardiac ischemia and blood was sent to the laboratory for various tests, including a determination of cardiac troponin T (TnT) levels. She was given medications to treat her acute cardiac ischemia.
I. General Structure of the Amino Acids
Twenty different amino acids are commonly found in proteins. They are all α-amino acids—amino acids in which the amino group is attached to the α-carbon (the carbon atom next to the carboxylate group) (see Fig. 6.1A). The α-carbon has two additional substituents: a hydrogen atom and an additional chemical group called a side chain (–R). The side chain is different for each amino acid.
At a physiologic pH of 7.4, the amino group on these amino acids carries a positive charge and the carboxylic acid group is negatively charged (see Fig. 6.1B). The pKa of the primary carboxylic acid groups for all of the amino acids is approximately 2 (1.8 to 2.4). At pH values much lower than the pKa (higher hydrogen ion concentrations), all of the carboxylic acid groups are protonated. At the pKa, 50% of the molecules are dissociated into carboxylate anions and protons, and at a pH of 7.4, over 99% of the molecules are dissociated (see Chapter 4). The pKa for all of the α-amino groups is approximately 9.5 (8.8 to 11.0), so that at the lower pH of 7.4, most of the amino groups are fully protonated and carry a positive charge. The form of an amino acid that has both a positive and a negative charge is called a zwitterion. Because these charged chemical groups can form hydrogen bonds with water molecules, all of these amino acids are water-soluble at physiologic pH.
In all of the amino acids but glycine (where the side chain is a hydrogen), the α-carbon is an asymmetric carbon atom that has four different substituents and can exist in either the D- or L-configuration (Fig. 6.2). The amino acids in mammalian proteins are all L-amino acids represented with the amino group to the left if the carboxyl group is at the top of the structure. These same amino acids serve as precursors of nitrogen-containing compounds that are synthesized in the body, and thus human amino acid metabolism is also centered on L-amino acids. The amino acid glycine is neither D nor L because the α-carbon atom contains two hydrogen atoms and is not an asymmetric carbon.
FIGURE 6.2 L– and D-Amino acids. The L-forms are the only ones found in human proteins. Bonds coming out of the paper are shown by black arrows; those going in, by shaded arrows. The α-amino groups and H atoms come toward the reader, and the α-carboxyl and side chains go away from the reader. The L- and D-forms are mirror images that cannot be superimposed by rotating the molecule. The reference for the L-and D-forms is the stereoisomers of glyceraldehyde (see Fig. 5.10A). R, side chain.
The chemical properties of the amino acids give each protein its unique characteristics. Proteins are composed of one or more linear polypeptide chains and may contain hundreds of amino acids. The sequence of amino acids, termed the primary structure, is determined by the genetic code for the protein. In the polypeptide chains, amino acids are joined through peptide bonds between the carboxylic acid of one amino acid and the amino group of the adjacent amino acid (Fig. 6.3, see also Fig. 1.5). Thus, the amino group, the α-carbon, and the carboxyl groups form the peptide backbone, and the side chains of the amino acids extend outward from this backbone. The side chains interact with the peptide backbone of other regions of the chain or with the side chains of other amino acids in the protein to form hydrophobic regions, electrostatic bonds, hydrogen bonds, or disulfide bonds. These interactions dictate the folding pattern of the molecule. The three-dimensional folding of the protein forms distinct regions called binding sites that are lined with amino acid side chains that interact specifically with another molecule termed a ligand (such as the heme in hemoglobin). Thus, the chemical properties of the side chains determine how the protein folds, how it binds specific ligands, and how it interacts with its environment (such as the aqueous medium of the cytoplasm). Each chain will have a carboxyl terminal and an amino terminal. The amino terminal is the first amino acid in the chain, which contains a free amino group. The carboxyl terminal is the last amino acid in the chain, which contains a free carboxylate group.
FIGURE 6.3 Peptide bonds. Amino acids in a polypeptide chain are joined through peptide bonds between the carboxyl group of one amino acid and the amino group of the next amino acid in the sequence. R, side chain.
II. Classification of Amino Acid Side Chains
In Figure 6.4, the 20 amino acids used for protein synthesis are grouped into different classifications according to the polarity and structural features of the side chains. These groupings can be helpful in describing common functional roles or metabolic pathways of the amino acids. However, some amino acid side chains fit into a number of different classifications and are therefore grouped differently in different textbooks. Two of the characteristics of the side chain that are useful for classification are its pKa and its hydropathic index, listed in Table 6.1. The hydropathic index is a scale used to denote the hydrophobicity of the side chain; the more positive the hydropathic index, the greater is the tendency to cluster with other nonpolar molecules and exclude water in the hydrophobic effect. These hydrophobic side chains tend to occur in membranes or in the center of a folded protein, where water is excluded. The more negative the hydropathic index of an amino acid, the more hydrophilic is its side chain.
TABLE 6.1 Properties of the Common Amino Acids
aWhen these amino acids reside in proteins, the pKa for the side chains may vary to some extent from the value for the free amino acid, depending on the local environment of the amino acid in the three-dimensional structure of the protein.
bThe hydropathy index is a measure of the hydrophobicity of the amino acid (the higher the number, the more hydrophobic). Values based on Kyte J, Doolittle RF. A simple method for displaying the hydropathic character of a protein. J Mol Biol. 1982;157:105–132.
FIGURE 6.4 The side chains of the amino acids. The side chains are highlighted. The amino acids are grouped by the polarity and structural features of their side chains. These groupings are not absolute, however. Tyrosine and tryptophan, often listed with the nonpolar amino acids, are more polar than other aromatic amino acids because of their phenolic and indole rings, respectively. The single-letter and three-letter codes are also indicated for each amino acid.
The names of the different amino acids have been given three-letter and one-letter abbreviations (see Fig. 6.4). The three-letter abbreviations use the first two letters in the name plus the third letter of the name or the letter of a characteristic sound, such as “trp” for tryptophan. The one-letter abbreviations use the first letter of the name of the most frequent amino acid in proteins (such as an “A” for alanine). If the first letter has already been assigned, the letter of a characteristic sound is used (such as an “R” for arginine). Single-letter abbreviations are usually used to denote the amino acids in a polypeptide sequence.
A. Nonpolar, Aliphatic Amino Acids
Glycine is the simplest amino acid, and it does not fit well into any classification because its side chain is just a hydrogen atom. Because the side chain of glycine is so small compared with that of other amino acids, it causes the least amount of steric hindrance in a protein (i.e., it does not significantly impinge on the space occupied by other atoms or chemical groups). Therefore, glycine is often found in bends or in the tightly packed chains of fibrous proteins.
Alanine and the branched-chain amino acids (valine, leucine, and isoleucine) have bulky, nonpolar, aliphatic (open-chain hydrocarbons) side chains and exhibit a high degree of hydrophobicity (see Table 6.1). Electrons are shared equally between the carbon and hydrogen atoms in these side chains, so that they cannot hydrogen bond with water. Within proteins, these amino acid side chains will cluster together to form hydrophobic cores. Their association is also promoted by van der Waals forces between the positively charged nucleus of one atom and the electron cloud of another. This force is effective over short distances when many atoms pack closely together.
The role of proline in amino acid structure differs from those of the nonpolar amino acids. The amino acid proline contains a ring involving its α-carbon and its α-amino group, which are part of the peptide backbone. It is an imino acid. This rigid ring causes a kink in the peptide backbone that prevents it from forming its usual configuration and it will restrict the conformation of the protein at that point.
B. Aromatic Amino Acids
The aromatic amino acids have been grouped together because they all contain ring structures with similar properties, but their polarities differ a great deal. The aromatic ring is a six-membered carbon–hydrogen ring with three conjugated double bonds (the benzene ring or phenyl group). These hydrogen atoms do not participate in hydrogen bonding. The substituents on this ring determine whether the amino acid side chain engages in polar or hydrophobic interactions. In the amino acid phenylalanine, the ring contains no substituents, and the electrons are shared equally between the carbons in the ring, resulting in a very nonpolar hydrophobic structure in which the rings can stack on each other (Fig. 6.5). In tyrosine, a hydroxyl group on the phenyl ring engages in hydrogen bonds, and the side chain is therefore more polar and more hydrophilic. The more complex ring structure in tryptophan is an indole ring with a nitrogen that can engage in hydrogen bonds. Tryptophan is therefore also more polar than phenylalanine.
FIGURE 6.5 Hydrophobic and hydrogen bonds. A. Strong hydrophobic interactions occur with the stacking of aromatic groups in phenylalanine side chains. B. Examples of hydrogen bonds in which a hydrogen atom is shared by a nitrogen in the peptide backbone and an oxygen atom in an amino acid side chain or between an oxygen in the peptide backbone and an oxygen in an amino acid side chain. R, side chain.
C. Aliphatic, Polar, Uncharged Amino Acids
Amino acids with side chains that contain an amide group (asparagine and glutamine) or a hydroxyl group (serine and threonine) can be classified as aliphatic, polar, uncharged amino acids. Asparagine and glutamine are amides of the amino acids aspartate and glutamate. The hydroxyl groups and the amide groups in the side chains allow these amino acids to form hydrogen bonds with water, with each other and the peptide backbone, or with other polar compounds in the binding sites of the proteins (see Fig. 6.5). As a consequence of their hydrophilicity, these amino acids are frequently found on the surface of water-soluble globular proteins. Cysteine, which is sometimes included in this class of amino acids, has been separated into the class of sulfur-containing amino acids.
D. Sulfur-Containing Amino Acids
Both cysteine and methionine contain sulfur. The side chain of cysteine contains a sulfhydryl group that has a pKa of approximately 8.4 for dissociation of its hydrogen, so cysteine is predominantly undissociated and uncharged at the physiologic pH of 7.4. The free cysteine molecule in solution can form a covalent disulfide bond with another cysteine molecule through spontaneous (nonenzymatic) oxidation of their sulfhydryl groups. The resultant amino acid, cystine, is present in blood and tissues, and is not very water-soluble. In proteins, the formation of a cystine disulfide bond between two appropriately positioned cysteine sulfhydryl groups often plays an important role in holding two polypeptide chains or two different regions of a chain together (Fig. 6.6). Methionine, although it contains a sulfur group, is a nonpolar amino acid with a large bulky side chain that is hydrophobic. It does not contain a sulfhydryl group and cannot form disulfide bonds. Its important and central role in metabolism is related to its ability, when appropriately activated, to transfer the methyl group attached to the sulfur atom to other compounds.
FIGURE 6.6 A disulfide bond. Covalent disulfide bonds may be formed between two molecules of cysteine or between two cysteine residues in a protein. The disulfide compound is called cystine. The hydrogens of the cysteine sulfhydryl groups are removed during oxidation.
E. The Acidic and Basic Amino Acids
The amino acids aspartate and glutamate have carboxylic acid groups that carry a negative charge at physiologic pH (see Fig. 6.4). The basic amino acids histidine, lysine, and arginine have side chains containing nitrogen that can be protonated and positively charged at physiologic and lower pH values. Histidine has a nitrogen-containing imidazole ring for a side chain, lysine has a primary amino group on the ε-carbon (from the sequence α, β, γ, δ, ε), and arginine has a guanidinium group.
The positive charges on the basic amino acids enables them to form ionic bonds (electrostatic bonds) with negatively charged groups, such as the side chains of acidic amino acids or the phosphate groups of coenzymes (Fig. 6.7). In addition, lysine and arginine side chains often form ionic bonds with negatively charged compounds bound to the protein binding sites, such as the phosphate groups in adenosine triphosphate (ATP). The acidic and basic amino acid side chains also participate in hydrogen bonding and the formation of salt bridges (e.g., the binding of an inorganic ion such as Na+ between two partially or fully negatively charged groups).
FIGURE 6.7 Electrostatic interaction between the positively charged side chain of lysine and the negatively charged side chain of aspartate.
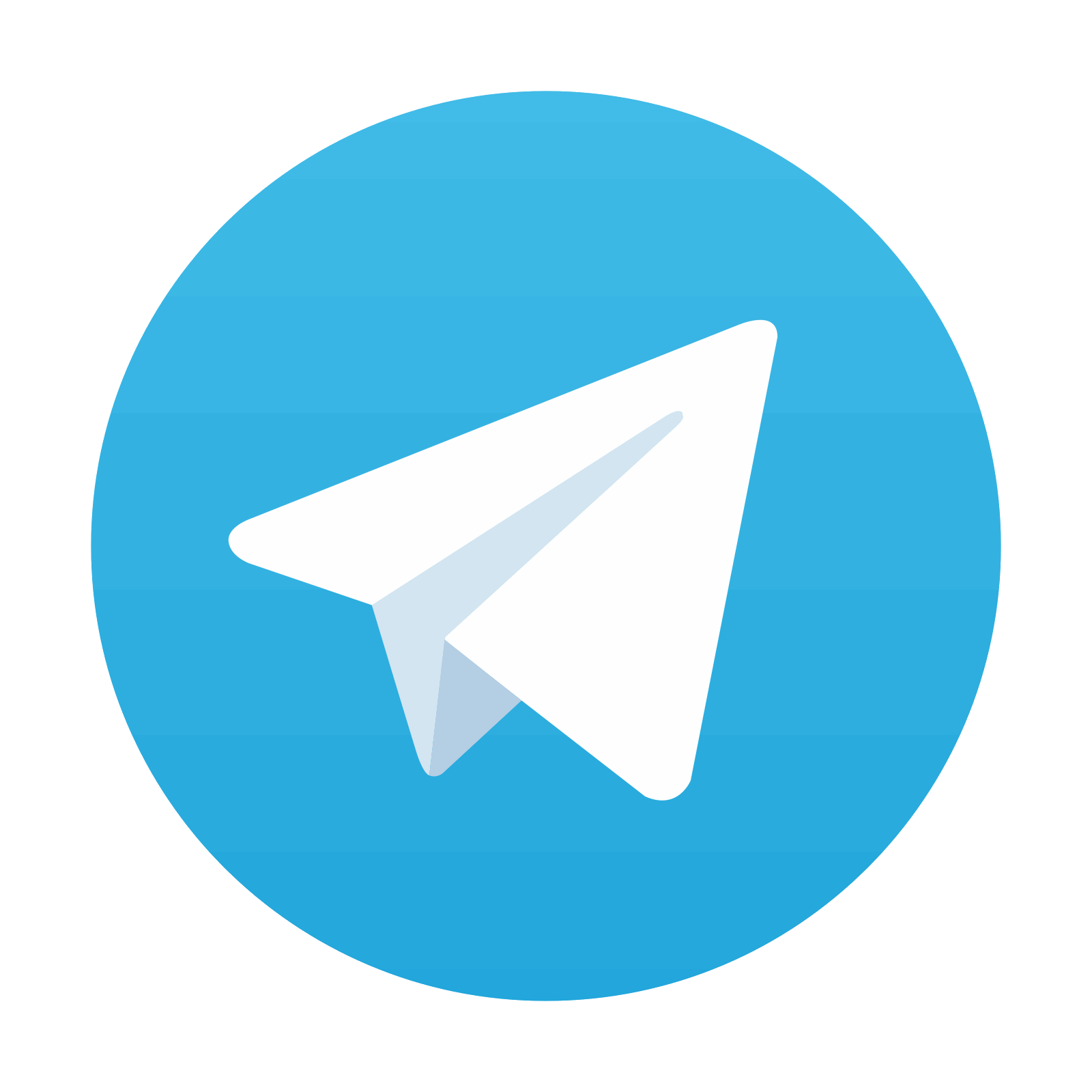
Stay updated, free articles. Join our Telegram channel

Full access? Get Clinical Tree
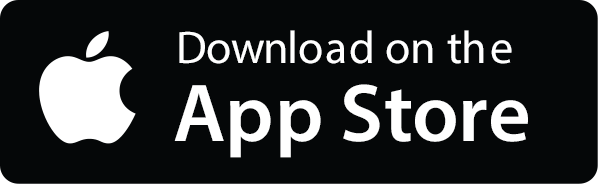
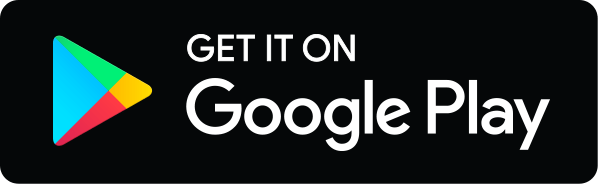