The actions of two enzymes—monoamine oxidase and catechol-O-methyltransferase (COMT)—explain why the catecholamines have short half-lives and cannot be used orally. MAO and COMT are located in the liver and in the intestinal wall. Both enzymes are very active and quickly destroy catecholamines administered by any route. Because these enzymes are located in the liver and intestinal wall, catecholamines that are administered orally become inactivated before they can reach the systemic circulation. Hence catecholamines are ineffective if given by mouth. Because of rapid inactivation by MAO and COMT, three catecholamines—NE, dopamine, and dobutamine—are effective only if administered by continuous infusion. Administration by other parenteral routes (e.g., subcutaneously, intramuscularly) will not yield adequate blood levels, owing to rapid hepatic inactivation.
Catecholamines are polar molecules, so they cannot cross the blood-brain barrier. (Recall from Chapter 4 that polar compounds penetrate membranes poorly.) The polar nature of the catecholamines is due to the hydroxyl groups on the catechol portion of the molecule. Because they cannot cross the blood-brain barrier, catecholamines have minimal effects on the CNS.
Be aware that catecholamine-containing solutions, which are colorless when first prepared, turn pink or brown over time. This pigmentation is caused by oxidation of the catecholamine molecule. Catecholamine solutions should be discarded as soon as discoloration develops. The only exception is dobutamine, which can be used up to 24 hours after the solution was made, even if discoloration appears.
Noncatecholamines
The noncatecholamines have ethylamine in their structure (see Fig. 13.1), but do not contain the catechol moiety that characterizes the catecholamines. Here we discuss three noncatecholamines: ephedrine, albuterol, and phenylephrine.
The noncatecholamines differ from the catecholamines in three important respects. First, because they lack a catechol group, noncatecholamines are not substrates for COMT and are metabolized slowly by MAO. As a result, the half-lives of noncatecholamines are much longer than those of catecholamines. Second, because they do not undergo rapid degradation by MAO and COMT, noncatecholamines can be given orally, whereas catecholamines cannot. Third, noncatecholamines are considerably less polar than catecholamines and hence are more able to cross the blood-brain barrier.
Receptor Specificity
To understand the actions of individual adrenergic agonists, we need to know their receptor specificity. Variability in receptor specificity among the adrenergic agonists can be illustrated with three drugs: albuterol, isoproterenol, and epinephrine. Albuterol is highly selective, acting at beta2 receptors only. Isoproterenol is less selective, acting at beta1 receptors and beta2 receptors. Epinephrine is even less selective, acting at all four adrenergic receptor subtypes: alpha1, alpha2, beta1, and beta2.
The receptor specificities of the major adrenergic agonists are shown in Table 13.2. In the upper part of the table, receptor specificity is presented in tabular form. In the lower part, the same information is presented schematically. By learning this content, you will be well on your way to understanding the pharmacology of the sympathomimetic drugs.
TABLE 13.2
Receptor Specificity of Representative Adrenergic Agonists
α, alpha; β, beta.
*Ephedrine is a mixed-acting agent that causes NE release and also activates alpha and beta receptors directly.
†Receptor activation by dopamine is dose dependent.
‡This chart presents in graphic form the same information on receptor specificity given above. Arrows indicate the range of receptors that the drugs can activate (at usual therapeutic doses).
Note that the concept of receptor specificity is relative, not absolute. The ability of a drug to selectively activate certain receptors to the exclusion of others depends on the dosage: at low doses, selectivity is maximal; as dosage increases, selectivity declines. For example, when albuterol is administered in low to moderate doses, the drug is highly selective for beta2-adrenergic receptors. However, if the dosage is high, albuterol will activate beta1 receptors as well. The information on receptor specificity in Table 13.2 refers to usual therapeutic doses. So-called selective agents will activate additional adrenergic receptors if the dosage is abnormally high.
Therapeutic Applications and Adverse Effects of Adrenergic Receptor Activation
In this section we discuss the responses—both therapeutic and adverse—that can be elicited with sympathomimetic drugs. Because many adrenergic agonists activate more than one type of receptor (see Table 13.2), it could be quite confusing if we were to talk about the effects of the sympathomimetics while employing specific drugs as examples. Consequently, rather than attempting to structure this presentation around representative drugs, we discuss the actions of the adrenergic agonists one receptor at a time. Our discussion begins with alpha1 receptors, and then moves to alpha2 receptors, beta1 receptors, beta2 receptors, and finally dopamine receptors. For each receptor type, we discuss both the therapeutic and adverse responses that can result from receptor activation.
To understand the effects of any specific adrenergic agonist, all you need are two types of information: (1) the identity of the receptors at which the drug acts and (2) the effects produced by activating those receptors. Combining these two types of information will reveal a profile of drug action. This is the same approach to understanding neuropharmacologic agents that we discussed in Chapter 10.
Before you continue, I encourage you to review Table 11.3 in Chapter 11. We are about to discuss the clinical consequences of adrenergic receptor activation, and Table 11.3 shows the responses to activation of those receptors. If you choose not to memorize Table 11.3 now, be prepared to refer back to it as we discuss the consequences of receptor activation.
Clinical Consequences of Alpha1 Activation
In this section we discuss the therapeutic and adverse effects that can result from activation of alpha1-adrenergic receptors. As shown in Table 13.2, drugs capable of activating alpha1 receptors include epinephrine, NE, phenylephrine, ephedrine, and dopamine.
Therapeutic Applications of Alpha1 Activation
Activation of alpha1 receptors elicits two responses that can be of therapeutic use: (1) vasoconstriction (in blood vessels of the skin, viscera, and mucous membranes); and (2) mydriasis. Of the two, vasoconstriction is the one for which alpha1 agonists are used most often. Using these drugs for mydriasis is rare.
Hemostasis
Hemostasis is defined as the arrest of bleeding, which alpha1 agonists support through vasoconstriction. Alpha1 agonists are given to stop bleeding primarily in the skin and mucous membranes. Epinephrine, applied topically, is the alpha1 agonist used most for this purpose.
Nasal Decongestion
Nasal congestion results from dilation and engorgement of blood vessels in the nasal mucosa. Drugs can relieve congestion by causing alpha1-mediated vasoconstriction. Specific alpha1-activating agents employed as nasal decongestants include phenylephrine (administered topically) and pseudoephedrine (administered orally).
Adjunct to Local Anesthesia
Alpha1 agonists are frequently combined with local anesthetics to delay systemic absorption. The mechanism is alpha1-mediated vasoconstriction, which reduces blood flow to the site of anesthetic administration. Why delay anesthetic absorption? Because keeping the drug at the local site of action prolongs anesthesia, allows a reduction in anesthetic dosage, and reduces the systemic effects that a local anesthetic might produce. The drug used most frequently to delay anesthetic absorption is epinephrine.
Elevation of Blood Pressure
Because of their ability to cause vasoconstriction, alpha1 agonists can elevate blood pressure in hypotensive patients. Please note, however, that alpha1 agonists are not the primary therapy for hypotension. Rather, they are reserved for situations in which fluid replacement and other measures either are contraindicated or have failed to restore blood pressure to a satisfactory level.
Mydriasis
Activation of alpha1 receptors on the radial muscle of the iris causes mydriasis (dilation of the pupil), which can facilitate eye examinations and ocular surgery. Note that producing mydriasis is the only clinical use of alpha1 activation that is not based on vasoconstriction.
Adverse Effects of Alpha1 Activation
All of the adverse effects caused by alpha1 activation result directly or indirectly from vasoconstriction.
Hypertension
Alpha1 agonists can produce hypertension by causing widespread vasoconstriction. Severe hypertension is most likely with parenteral dosing. Accordingly, when alpha1 agonists are given intravenously, the patient’s cardiac rhythm must be monitored continuously, and other indicators of cardiovascular status and perfusion (e.g., blood pressure, peripheral pulses, urine output) should be assessed frequently.
Necrosis
If the IV line used to administer an alpha1 agonist becomes extravasated, seepage of the drug into the surrounding tissues may result in necrosis (tissue death). The cause is lack of blood flow to the affected area secondary to intense local vasoconstriction. If extravasation occurs, the area should be infiltrated with an alpha1-blocking agent (e.g., phentolamine), which will counteract alpha1-mediated vasoconstriction and thereby help minimize injury.
Bradycardia
Alpha1 agonists can cause reflex slowing of the heart. The mechanism is this: alpha1-mediated vasoconstriction elevates blood pressure, which triggers the baroreceptor reflex, causing heart rate to decline. In patients with marginal cardiac reserve, the decrease in cardiac output may compromise tissue perfusion.
Clinical Consequences of Alpha2 Activation
Alpha2 receptors in the periphery are located presynaptically, and their activation inhibits NE release. Several adrenergic agonists (e.g., epinephrine, NE) are capable of causing alpha2 activation. However, their ability to activate alpha2 receptors in the periphery has little clinical significance because there are no therapeutic applications related to activation of peripheral alpha2 receptors. Furthermore, activation of these receptors rarely causes significant adverse effects.
In contrast to alpha2 receptors in the periphery, alpha2 receptors in the CNS are of great clinical significance. By activating central alpha2 receptors, we can produce two useful effects: (1) reduction of sympathetic outflow to the heart and blood vessels and (2) relief of severe pain. The central alpha2 agonists used for effects on the heart and blood vessels, and the agents used to relieve pain, are discussed in Chapters 15 and 22, respectively.
Clinical Consequences of Beta1 Activation
All of the clinically relevant responses to activation of beta1 receptors result from activating beta1 receptors in the heart; activation of renal beta1 receptors is not associated with either beneficial or adverse effects. As indicated in Table 13.2, beta1 receptors can be activated by epinephrine, NE, isoproterenol, dopamine, dobutamine, and ephedrine.
Therapeutic Applications of Beta1 Activation
Heart Failure
Heart failure is characterized by a reduction in the force of myocardial contraction, resulting in insufficient cardiac output. Because activation of beta1 receptors in the heart has a positive inotropic effect (i.e., increases the force of contraction), drugs that activate these receptors can improve cardiac performance.
Shock
This condition is characterized by profound hypotension and greatly reduced tissue perfusion. The primary goal of treatment is to maintain blood flow to vital organs. By increasing heart rate and force of contraction, beta1 stimulants can increase cardiac output and can thereby improve tissue perfusion.
Atrioventricular Heart Block
Atrioventricular (AV) heart block is a condition in which impulse conduction from the atria to the ventricles is either impeded or blocked entirely. As a consequence, the ventricles are no longer driven at an appropriate rate. Because activation of cardiac beta1 receptors can enhance impulse conduction through the AV node, beta1 stimulants can help overcome AV block. It should be noted, however, that drugs are only a temporary form of treatment. For long-term management, a pacemaker is implanted.
Cardiac Arrest
By activating cardiac beta1 receptors, drugs have a role in initiating contraction in asystole or pulseless ventricular rhythms. It should be noted, however, that drugs are not the preferred treatment. Initial management focuses on cardiopulmonary resuscitation, external pacing, or defibrillation (whichever is applicable), and identification and treatment of the underlying cause (e.g., hypoxia, severe acidosis, drug overdose). When a beta1 agonist is indicated, epinephrine, administered intravenously, is the preferred drug. If IV access is not possible, epinephrine can be injected directly into the heart or endotracheally.
Adverse Effects of Beta1 Activation
All of the adverse effects of beta1 activation result from activating beta1 receptors in the heart. Activating renal beta1 receptors is not associated with untoward effects.
Altered Heart Rate or Rhythm
Overstimulation of cardiac beta1 receptors can produce tachycardia (excessive heart rate) and dysrhythmias (irregular heartbeat).
Angina Pectoris
In some patients, drugs that activate beta1 receptors can precipitate an attack of angina pectoris, a condition characterized by substernal pain in the region of the heart. Anginal pain occurs when cardiac oxygen supply (blood flow) is insufficient to meet cardiac oxygen needs. The most common cause of angina is coronary atherosclerosis (accumulation of lipids and other substances in coronary arteries). Because beta1 agonists increase cardiac oxygen demand (by increasing heart rate and force of contraction), patients with compromised coronary circulation are at risk for an anginal attack.
Clinical Consequences of Beta2 Activation
Applications of beta2 activation are limited to the lungs and the uterus. Drugs used for their beta2-activating ability include epinephrine, isoproterenol, and albuterol.
Therapeutic Applications of Beta2 Activation
Asthma
Asthma is a chronic condition characterized by inflammation and bronchoconstriction occurring in response to a variety of stimuli. During a severe attack, the airflow reduction can be life threatening. Because drugs that activate beta2 receptors in the lungs promote bronchodilation, these drugs can help relieve or prevent asthma attacks.
For therapy of asthma, adrenergic agonists that are selective for beta2 receptors (e.g., albuterol) are preferred to less selective agents (e.g., isoproterenol). This is especially true for patients who also suffer from angina pectoris or tachycardia because drugs that can activate beta1 receptors would aggravate these cardiac disorders.
Most beta2 agonists used to treat asthma are administered by inhalation. This route is desirable in that it helps minimize adverse systemic effects. It should be noted, however, that inhalation does not guarantee safety: Serious systemic toxicity can result from overdosing with inhaled sympathomimetics, so patients must be warned against inhaling too much drug.
Delay of Preterm Labor
Activation of beta2 receptors in the uterus relaxes uterine smooth muscle. This action can be employed to delay preterm labor.
Adverse Effects of Beta2 Activation
Hyperglycemia
The most important adverse response to beta2 activation is hyperglycemia (elevation of blood glucose). The mechanism is activation of beta2 receptors in the liver and skeletal muscles, which promotes breakdown of glycogen into glucose. As a rule, beta2 agonists cause hyperglycemia only in patients with diabetes; in patients with normal pancreatic function, insulin release will maintain blood glucose at an appropriate level. If hyperglycemia develops in the patient with diabetes, medications used for glucose control will need to be adjusted.
Tremor
Tremor is the most common side effect of beta2 agonists. It occurs because activation of beta2 receptors in skeletal muscle enhances contraction. This effect can be confounding for patients with diabetes because tremor is a common symptom of hypoglycemia; however, when due to beta2 activation, it may be accompanied by hyperglycemia. Fortunately, the tremor generally fades over time and can be minimized by initiating therapy at low doses.
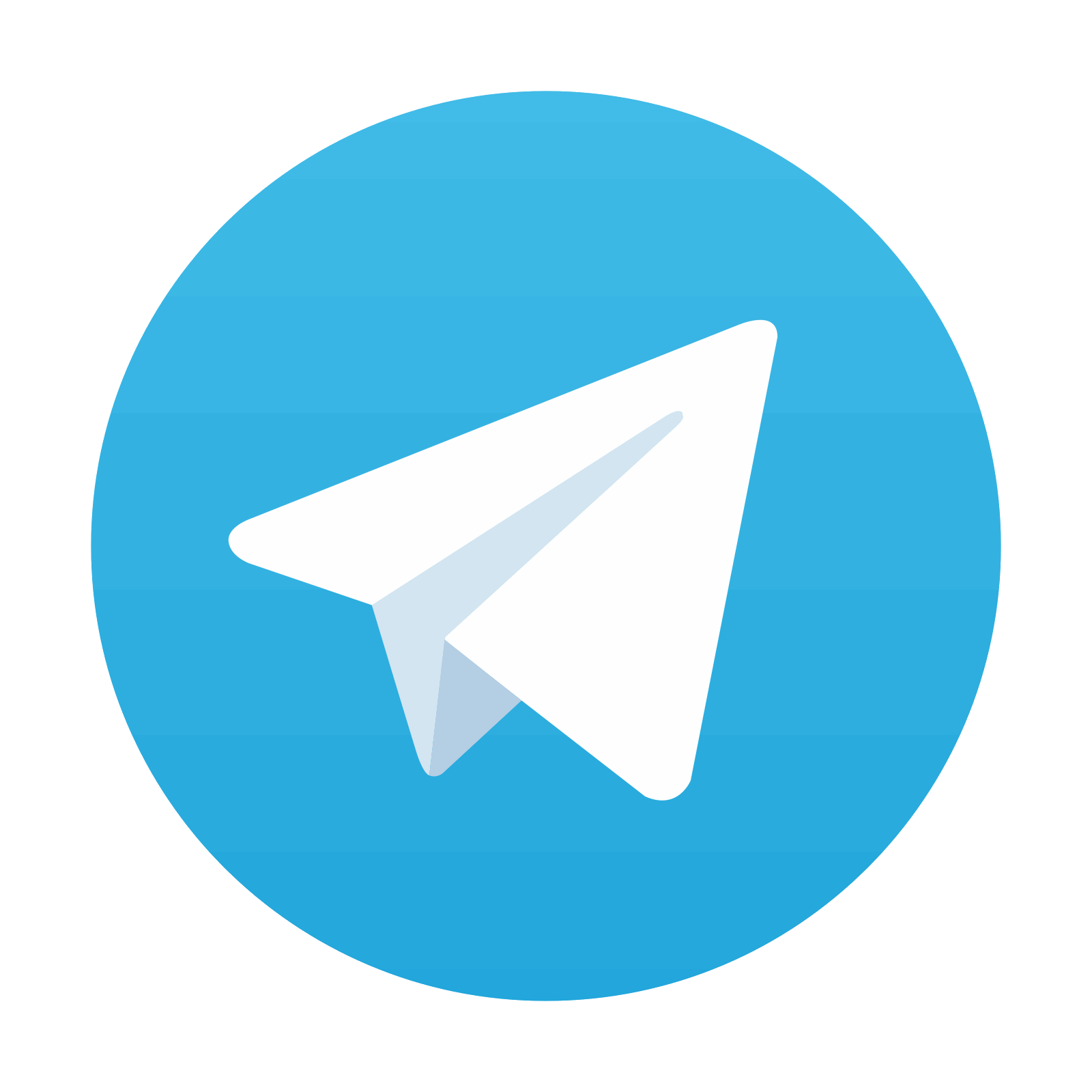
Stay updated, free articles. Join our Telegram channel

Full access? Get Clinical Tree
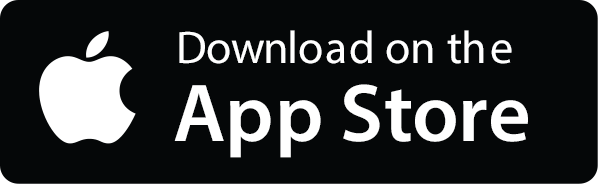
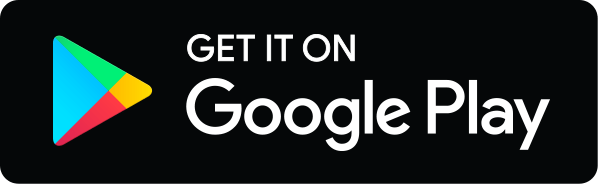