10 Adaptive responses provide a ‘quantum leap’ in effective defence
Infectious agents frequently find ways around the innate defences
In Chapter 9, we discussed the many ways in which the primary or innate defences of the body may counteract microbial infection. However, infectious agents frequently find ways around these defences, as there is a huge number of different microorganisms surrounding us and they have a powerful ability to mutate, e.g.:
• The surface of some microbes fails to activate the alternative complement pathway.
• Other microbes can activate the alternative complement pathway, but do so at the end of flagella, so that the membrane attack complex builds up at a site distant from the body of the organism and therefore causes no damage.
• In other cases, microorganisms taken into the body of the macrophage develop subterfuges that prevent the development of the awesome battery of microbicidal mechanisms that the macrophage normally expresses (see Ch. 16).
• Cells infected with certain viruses may prove to be resistant to the cytotoxic action of natural killer cells, or the viruses may be only weak stimulators of interferon, so that cell-to-cell transmission of the virus proceeds unchecked.
• Yet another microbial subterfuge is the production of bacterial toxins that can kill the phagocyte if not neutralized.
Adaptive responses act against microorganisms that overcome the innate defences
The role of antibodies
The acute inflammatory response
Antibodies act as adaptors to focus acute inflammatory reactions
Antibodies are immunoglobulin molecules (Fig. 10.1, Table 10.1) which are synthesized by host B lymphocytes (so-called because they mature in the bone marrow; see Fig. 11.2) when they make contact with an infectious microbe, which acts as a foreign antigen (i.e. it generates antibodies). Each antibody has two identical recognition sites that are complementary in shape to the surface of the foreign antigen and which enable it to bind with varying degrees of strength to that antigen. The recognition site is hypervariable in that antibodies of different antigen specificities each have a unique amino acid sequence in this region. This hypervariability is confined to three loops on the heavy and three on the light peptide chains, which make up the antibody molecule (Fig. 10.1) and are referred to as complementarity determining regions (CDRs) because they make complementary contact with the antigen. Thus, the amino acid sequences of these CDRs determine which antigen is recognized by a given antibody. Other sites on the antibody molecule are specialized for functions such as activating the complement system and inducing phagocytosis by macrophages and polymorphs (Fig. 10.2). Therefore, when a microbial antigen is coated with several of these adaptor antibody molecules, they induce complement fixation and phagocytosis, processes that the microbe may well have evolved to try and avoid. In this way, the reluctant microorganism becomes drawn into the innate defence mechanism of the acute inflammatory response. We will now examine the ways in which antibody can mediate these different phenomena.

Figure 10.2 The antibody adaptor molecule. Antibodies (anti-foreign bodies) are produced by host lymphocytes on contact with invading microbes, which act as antigens (i.e. generate antibodies). Each antibody (see Fig. 10.1) has a recognition site (Fab) enabling it to bind antigen, and a backbone structure (Fc) capable of some secondary biologic action such as activating complement and phagocytosis. Thus, in the present case, antibody bound to the microbe activates complement and initiates an acute inflammatory reaction (cf. Fig. 9.14). The C3b generated fixes to the microbe and, together with the antibody molecules, facilitates adherence to Fc and C3b receptors on the phagocyte and thence microbial ingestion.
Antibody complexed with antigen activates complement through the ‘classical’ pathway
When antibody molecules bind an antigen, the resulting complex activates the first component of complement, C1, converting it into an esterase (). This initiates a second route of complement activation (Fig. 10.3) termed the ‘classical’ pathway, mainly because scientists discovered it before the ‘alternative’ pathway (see Ch. 9), although the evidence indicates that the alternative pathway is of greater antiquity in evolutionary terms. The activated first component splits off a small peptide from each of the succeeding components C4 and C2, the residual fragments forming a composite, the
, complex. The
complex has the enzymatic ability or property of a C3 convertase. Being an enzyme, the
protease creates large numbers of the
convertase which itself also having proteolytic activity, cleaves many C3 molecules, this so-called enzyme cascade providing a mechanism for the striking amplification of the relatively few initial complement activation events.
has a similar function to the alternative pathway C3 convertase,
, and the sequence of events following the splitting of C3 which generates an acute inflammatory response is indistinguishable from that occurring in the alternative pathway. C3a and C5a anaphylatoxins are formed, and C3b binds to the surface of the microbe–antibody complex (Fig. 10.4; compare Fig. 9.14). Subsequently, the later components are assembled into a membrane attack complex (MAC) (see Fig. 9.18), which may help to kill the microorganism if it has been focused onto a vulnerable site.

Figure 10.3 Comparison of the alternative classical and mannose binding lectin complement pathways. All converge with the formation of C3 convertase enzymes (in heavily outlined boxes) which split the dominant protein C3 into the C3b fragment, an event which is at the heart of the complement interactions. The complex of antibody with microbial antigen activates the first component of the ‘classical’ pathway (step 1) leading to cleavage of C3 through the , C3 convertase. Mannose binding lectin, when combined with microbial surface carbohydrate, associates with serine proteases MASP-1 and -2 which split C4 and C2, just like
. In contrast, the activation of the ‘alternative’ pathway depends upon stabilization of the C3 convertase (
) on the microbial surface produced by the feedback loop (cf. Fig. 9.13). The molecular units with protease activity are highlighted in green, the enzymatic domains showing considerable homology. Note that the acute phase protein, C-reactive protein, on binding to microbial phosphorylcholine, can trigger the classical pathway. An upper bar (—) indicates an active complex.
It is appropriate at this stage to recall the activation of complement by innate immune mechanisms involving the binding of mannose binding lectin (MBL) and C-reactive protein to carbohydrates on microbial surfaces (cf. Fig. 9.20), and it is noteworthy that both acute phase proteins activate the classical pathway albeit through different routes (Fig. 10.3).
The acute inflammatory reaction can also be initiated by antibody bound to mast cells
A specialized antibody, immunoglobulin E (IgE), has a backbone site with a high affinity for specific receptors on the surface of mast cells. When microbial antigen attaches to these cell-bound antibodies the surface receptors are cross-linked and transduce a signal to the interior of the cell. This signal leads to the release of mediators capable of increasing vascular permeability and inducing polymorph chemotaxis (Fig. 10.5).
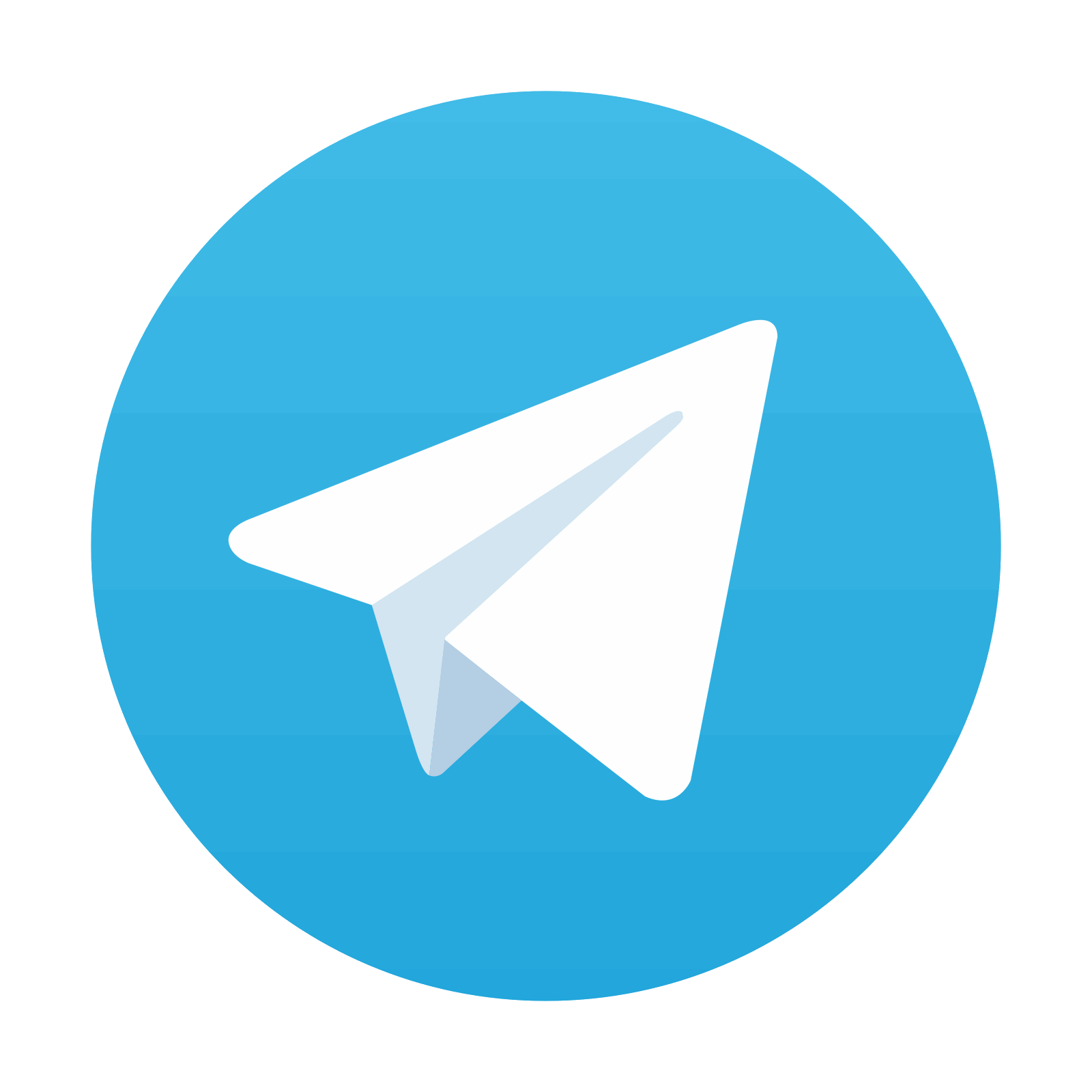
Stay updated, free articles. Join our Telegram channel

Full access? Get Clinical Tree
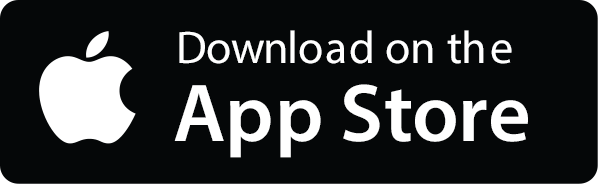
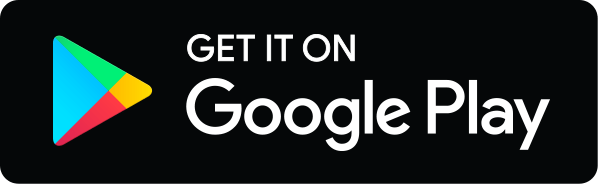