heart is like wax; it is melted in the midst of my bowels.
—The Bible: Psalms 22:14
This group of curved Gram-negative rods includes Vibrio cholerae, the cause of cholera, one of the first proven infectious diseases, along with Helicobacter pylori and Campylobacter jejuni, newcomers incriminated as pathogens late in the 20th century (Table 32–1). The peptic ulcer disease now known to be caused by Hpylori had been long accepted to be due to stress and disturbed gastric acid secretion. Campylobacter jejuni is one of the most common causes of diarrhea in virtually every country of the world. Cholera has undergone a resurgence in recent decades, spreading from its historic Asiatic locale to the Americas, including the coastline of the United States.
TABLE 32–1 Features of Vibrio, Campylobacter, and Helicobacter
VIBRIO
Vibrios are curved, Gram-negative rods (Figure 32–1) commonly found in saltwater. Cells may be linked end to end, forming S shapes and spirals. They are highly motile with a single polar flagellum, non–spore-forming, and oxidase-positive, and they can grow under aerobic or anaerobic conditions. The cell envelope structure is similar to that of other Gram-negative bacteria. Vibrio cholerae is the prototype cause of a water-loss diarrhea called cholera. Other species causing diarrhea, wound infections, and, rarely, systemic infection are listed in Table 32–2.
FIGURE 32–1. Vibrio cholerae (scanning electron micrograph). Note the curved rods and polar flagella. (Reproduced with permission from Willey JM: Prescott, Harley, & Klein’s Microbiology, 7th edition. McGraw-Hill, 2008.)
TABLE 32–2 Features of Less Common Vibrio and Campylobacter Species
Rapidly motile curved rods are found in seawater
VIBRIO CHOLERAE
BACTERIOLOGY
GROWTH AND STRUCTURE
Vibrio cholerae has a low tolerance for acid, but grows under alkaline (pH 8.0-9.5) conditions that inhibit many other Gram-negative bacteria. It is distinguished from other vibrios by biochemical reactions, lipopolysaccharide (LPS) O antigenic structure, and production of cholera toxin (CT). There are over 200 O antigen serotypes, only two of which (O1 and O139) cause cholera. Vibrio cholerae biogroup El Tor, an O1 variant, is a biotype of the classic strain. The O139 strains phenotypically resemble O1 El Tor strains but also produce a polysaccharide capsule. Vibrio cholerae possess long filamentous pili that form bundles on the bacterial surface and belong to a family of pili whose chemical structure is similar to those of the gonococcus and a number of other bacterial pathogens. All strains capable of causing cholera produce a colonizing factor known as the toxin-coregulated pilus (TCP) because its expression is regulated together with CT. In aquatic environments V cholerae produces polysaccharide biofilms, which contain carbohydrate moieties mediating cell–cell adhesion and attachment to surfaces.
Growth prefers alkaline over acid conditions
Cholera is limited to O1 and O139 serotypes
Biofilm produced in environment
CHOLERA TOXIN
The structure and mechanism of action of CT have been studied extensively (Figure 32–2). CT is an A–B type ADP-ribosylating toxin. Its molecule is an aggregate of multiple polypeptide chains organized into two toxic subunits (A1, A2) and five binding (B) units.
FIGURE 32–2. The action of cholera toxin. The complete toxin is shown binding to the GMI-ganglioside receptor on the cell membrane via the binding (B) subunits. The active portion (A1) of the A subunit catalyzes the ADP-ribosylation (ADPR) of the GS (stimulatory) regulatory protein, “locking” it in the active state. Because the GS protein acts to return adenylate cyclase from its inactive to active form, the net effect is persistent activation of adenylate cyclase. The increased adenylate cyclase (AC) activity results in accumulation of cyclic adenosine 3′, 5′-monophosphate (cAMP) along the cell membrane. The cAMP causes the active secretion of sodium (Na+), chloride (Cl–), potassium (K+), bicarbonate (HCO3–), and water out of the cell into the intestinal lumen.
B subunit receptor is a ganglioside on cell surface
A1 enters cytoplasm and ADP ribosylates regulatory G protein
The B units bind to a GM1-ganglioside receptor found on the surface of many types of cells. Once bound, the A1 subunit is released from the toxin molecule by reduction of the disulfide bond that binds it to the A2 subunit, and it enters the cell by translocation. In the cell, it exerts its effect on the membrane-associated adenylate cyclase system at the basolateral membrane surface. The target of the toxic A1 subunit is a guanine nucleotide (G) protein, Gsa, which regulates activation of the adenylate cyclase system. CT catalyzes the ADP ribosylation (Figure 21–16) of the G protein, rendering it unable to dissociate from the active adenylate cyclase complex. This causes persistent activation of intracellular adenylate cyclase, which in turn stimulates the conversion of adenosine triphosphate to cyclic adenosine 3′,5′-monophosphate (cAMP). The net effect is excessive accumulation of cAMP at the cell membrane, which causes hypersecretion of chloride, potassium, bicarbonate, and associated water molecules out of the cell. Strains of V cholerae other than the two epidemic serotypes may or may not produce CT.
Adenylate cyclase becomes locked in active state
Hyperproduction of cAMP causes hypersecretion of water and electrolytes
CHOLERA
EPIDEMIOLOGY
Epidemic cholera is spread primarily by contaminated water under conditions of poor sanitation, particularly where sewage treatment is absent or defective. Even though convalescent human carriage is brief, if the numerous vibrios purged from the intestines of those infected with cholera are able to reach the primary water supply, the conditions for spread are established. The short incubation period (2 days) ensures that organisms ingested by others quickly enter the epidemic cycle. Even so, modern travel makes imported cases of cholera possible. For instance, one man developed diarrhea in Florida after eating ceviche (marinated uncooked fish) just before departure from an airport in Ecuador.
Transmission is through untreated water supply
Incubation period is 2 days
Cholera is endemic in the Indian subcontinent and Africa. Over the last two centuries, its spread beyond this historic locale to other parts of Asia, Indonesia, and Europe has been described in eight great pandemics, each lasting 5 to 25 years. The current pandemic has brought cholera to the Western Hemisphere for the first time since 1911. Sporadic cases of cholera in the United States first appeared in the early 1970s and were traced to inadequately cooked crabs and shrimp caught off the Gulf Coast of Louisiana and Texas. In 1991, Latin America was hit with epidemic cholera with cases reported from 21 countries from Peru to northern Mexico. In Peru alone, over 500 000 cases of cholera and 4500 deaths occurred in 2 years. A massive epidemic of cholera followed the devastating earthquake of 2010 in Haiti. The disease is now endemic, claiming thousands of lives every year. Virulent V cholerae now lurks in coastal waters throughout the hemisphere and in the drinking water of locales with poor sanitation.
Cholera is endemic in India and Africa
Pandemics span decades
Gulf Coast cases result from undercooked shellfish
Latin American epidemic is widespread
The dominant strain of the 20th century was the El Tor biotype, first isolated from Mecca pilgrims at the El Tor quarantine camp in 1905. This strain survives slightly longer in nature and is more likely to produce subclinical cases of cholera, both of which facilitated its spread. In 1992, the first cases of cholera due to a serotype other than O1 were detected in India and Bangladesh. The new serotype (O139 Bengal) is fully virulent with the additional threat of enhanced ability to produce disease in persons whose immunity is due to exposure to the old serotype. Genomic analysis of the clonal Haitian epidemic strains showed them to be nearly identical to variant V cholera El Tor O1 strains isolated from Bangladesh in 2002 and 2008, likely introduced into Haiti by asymptomatic UN peacekeepers from South Asia, and quite different from earlier Peruvian isolates. These realities illustrate the potential for global spread of cholera and the challenges for the vaccine strategies designed to prevent it.
El Tor biotype dominated 20th century
New O139 serotype is spreading
The epidemic potential of V cholerae depends on its ability to survive in both aquatic environments and human hosts. In the environment, it persists in a dormant state in association with shellfish and plankton by attaching to their chitinous exoskeleton in the biofilms formed in the dormant seawater state but not during infection. This dual life is facilitated by a surface protein able to bind a constituent of chitin as well as glycoproteins and lipids on the intestinal epithelium. Satellite tracking has linked periodic climate changes (warming seawater), plankton blooms, and cholera epidemics along the coast of South America. Otherwise, the organism is fragile, surviving only a few days in the environment outside its human or crustacean hosts.
Survival in shellfish and plankton facilitates epidemics
PATHOGENESIS
To produce cholera, V cholerae must reach the small intestine, swim to the intestinal crypts, multiply, and produce virulence factors. In healthy people, ingestion of large numbers of bacteria is required to offset the acid barrier of the stomach. Colonization of the entire intestinal tract from the jejunum to the colon by V cholerae requires adherence to the epithelial surface by the abovementioned protein and surface pili. Bacteria recently passed from cholera cases are hyperinfectious by virtue of chemotactic motility facilitating colonization of the small intestine. The outstanding feature of V cholerae pathogenicity is the ability of virulent strains to secrete CT, which is responsible for the disease cholera. The water and electrolyte shift from the cell to the intestinal lumen is the fundamental cause of the watery diarrhea of cholera. Non-O1, non-O139 strains have been sporadically isolated from cases of gastroenteritis but do not produce CT, and thus not the disease cholera.
Large doses required to pass stomach acid barrier
Pili and proteins mediate epithelial adherence
CT-stimulated intestinal hypersecretion causes diarrhea
Fluid Loss
The fluid loss that results from the adenylate cyclase stimulation of cells depends on the balance between the amount of bacterial growth, toxin production, fluid secretion, and fluid absorption in the entire gastrointestinal tract. The outpouring of fluid and electrolytes is greatest in the small intestine, where the secretory capacity is high and absorptive capacity low. The diarrheal fluid can amount to many liters per day, with approximately the same NaCl content as plasma, but also significant potassium and bicarbonate. The result is dehydration (isotonic fluid loss), hypokalemia (potassium loss), and metabolic acidosis (bicarbonate loss). The intestinal mucosa remains unaltered except for some hyperemia, because V cholerae does not invade or otherwise injure the enterocyte.
Small intestine loses liters of fluid
K+ and bicarbonate losses cause hypokalemia and acidosis
Intestinal mucosa is structurally unaffected; no invasion
Genetic Regulation of Virulence
The expression of the multiple virulence factors of V cholerae is controlled in a coordinated 2-component systems involving environmental sensors and as many as 20 chromosomal genes divided between a pathogenicity island (PAI) containing CT and one containing TCP. The chief regulator is a transmembrane protein (ToxR) that “senses” environmental changes in pH, osmolarity, and temperature, which convert it to an active form. In the active state, ToxR can directly turn on CT genes as well as activate transcription of a second regulatory protein, ToxT. ToxT, whose natural effector may be bile, then activates transcription of virulence genes in both PAIs, including TCP and CT. Another set of environmental sensors switch V cholerae from free-swimming forms to the sessile, biofilm-forming state associated with environmental persistence in crustaceans. Quorum-sensing systems deploy expression of these virulence genes at a time when a critical mass of V cholerae is present to sustain it.
ToxR controls CT and TCP genes
Biofilm formation expressed in environmental crustaceans
IMMUNITY
Nonspecific defenses such as gastric acidity, gut motility, and intestinal mucus are important in preventing colonization with V cholerae. For example, in persons who lack gastric acidity (gastrectomy or achlorhydria from malnutrition), the attack rate of clinical cholera is higher. The immune state has been most strongly associated with sIgA directed against O-antigen LPS, CT (B subunit), and TCP. The precise protective mechanisms remain to be established.
Attack rate is higher with achlorhydria
Immunity is associated with sIgA
MANIFESTATIONS
Typical cholera has a rapid onset, beginning with abdominal fullness and discomfort, rushes of peristalsis, and loose stools. Vomiting may also occur. The stools quickly become watery, voluminous, almost odorless, and contain mucus flecks, giving it an appearance called rice-water stools. Neither white blood cells nor blood are in the stools, and the patient is afebrile. Clinical features of cholera result from the extensive fluid loss and electrolyte imbalance, which can lead to extreme dehydration, hypotension, and death within hours if untreated. No other disease produces dehydration as rapidly as cholera.
Extreme watery diarrhea causes large fluid loss
Dehydration and electrolyte imbalance are the major problems
DIAGNOSIS
The initial suspicion of cholera depends on recognition of the typical clinical features in an appropriate epidemiologic setting. A bacteriologic diagnosis is accomplished by isolation of V cholerae from the stool. The organism grows on common clinical laboratory media such as blood agar and MacConkey agar, but its isolation is enhanced by a selective medium (thio-sulfate–citrate–bile salt–sucrose agar). Once isolated, the organism is readily identified by biochemical reactions. Outside cholera-endemic areas, the selective medium is not routinely used for stool cultures, so clinical laboratories must be alerted to the suspicion of cholera.
Stool culture using selective media is required
TREATMENT
The outcome of cholera depends on balancing the diarrheal fluid and ionic losses with adequate fluid and electrolyte replacement. This is accomplished by oral and/or intravenous administration of solutions of glucose with near physiologic concentrations of sodium and chloride and higher than physiologic concentrations of potassium and bicarbonate. Exact formulas are available as dried packets to which a given volume of water is added. Oral replacement, particularly if begun early, is sufficient for all but the most severe cases and has substantially reduced the mortality from cholera. Antimicrobial therapy plays a secondary role to fluid replacement by shortening the duration of diarrhea and magnitude of fluid loss. A single dose of azithromycin provides optimal antimicrobial therapy, but doxycycline, a fluoroquinolone, or trimethoprim-sulfamethoxazole are also effective agents.
Oral or intravenous fluid and electrolyte replacement is crucial
Antimicrobial therapy can reduce duration and severity
PREVENTION
Epidemic cholera, a disease of poor sanitation, does not persist where treatment and disposal of human waste are adequate. Because good sanitary conditions do not exist in much of the world, secondary local measures such as boiling and chlorination of water during epidemics are required. Cholera associated with ingestion of crabs and shrimp can be prevented by adequate cooking (10 minutes) and avoidance of recontamination from containers and surfaces. Vaccines prepared from whole cells, lipopolysaccharide, and CT B subunit have been disappointing, providing protection that is not longlasting. Current interest includes live attenuated vaccine strains because of their potential to stimulate the local sIgA immune response.
Water sanitation and cooking shellfish prevent infection
Vaccines are disappointing
Other Vibrios
Species of Vibrio other than V cholerae may still produce disease, but are uncommon and typically restricted to seacoast locales. Vibrio parahaemolyticus produces a diarrheal illness after ingestion of raw or inadequately cooked seafood due to the production of a pair of its own enterotoxins. For virulence V vulnificus stands out because it can produce a rapidly progressive cellulitis in wounds sustained in seawater as well as a fatal bacteremic infection after ingestion of raw seafood. The latter has been common enough in Florida to threaten the local oyster trade. Cases were also seen in the area devastated by hurricane Katrina. Vibrio vulnificus is also a spectacular scavenger of host iron stores and produces particularly fulminant disease in persons with iron-overload states (eg, thalassemia, hemochromatosis). Features of these and other less common vibrios are shown in Table 32–2.
V parahaemolyticus in undercooked or raw seafood causes diarrhea
V vulnificus sepsis and wound infections linked to raw oysters and iron overload
CAMPYLOBACTER
Campylobacters are motile, curved, oxidase-positive, Gram-negative rods similar in morphology to vibrios. The cells have polar flagella and are often attached at their ends giving pairs “S” shapes or a “seagull” appearance. More than a dozen Campylobacter species have been associated with human disease. Of these, C jejuni is by far the most common and is discussed here as the prototype for intestinal disease. The features of other species are summarized in Table 32–2.
BACTERIOLOGY: CAMPYLOBACTER JEJUNI
Before 1973, C jejuni was not recognized as a cause of human disease. Not until selective methods for its isolation were developed was it recognized as one of the most common causes of infectious diarrhea. Like other campylobacters, C jejuni grows well only on enriched media under microaerophilic conditions. That is, it requires oxygen at reduced tension (5%-10%), presumably because of the vulnerability of some of its enzyme systems to super-oxides. Growth usually requires 2 to 4 days, sometimes as much as 1 week. Campylobacter jejuni has the structural components found in other Gram-negative bacteria (eg, outer membrane, LPS and LOS). The cells are actively motile through the action of a polar flagellum. In contrast to the vibrios, C jejuni does not break down carbohydrates, but uses amino acids and metabolic intermediates for energy. It is one of a number of pathogens that produce a membrane-bound protein called cytolethal distending toxin (CDT). CDT has an A/B toxin structure in which the A subunit is able to cause cell cycle arrest.
Microaerophilic atmosphere is required for growth
CDT is cytotoxin
CAMPYLOBACTER ENTERITIS
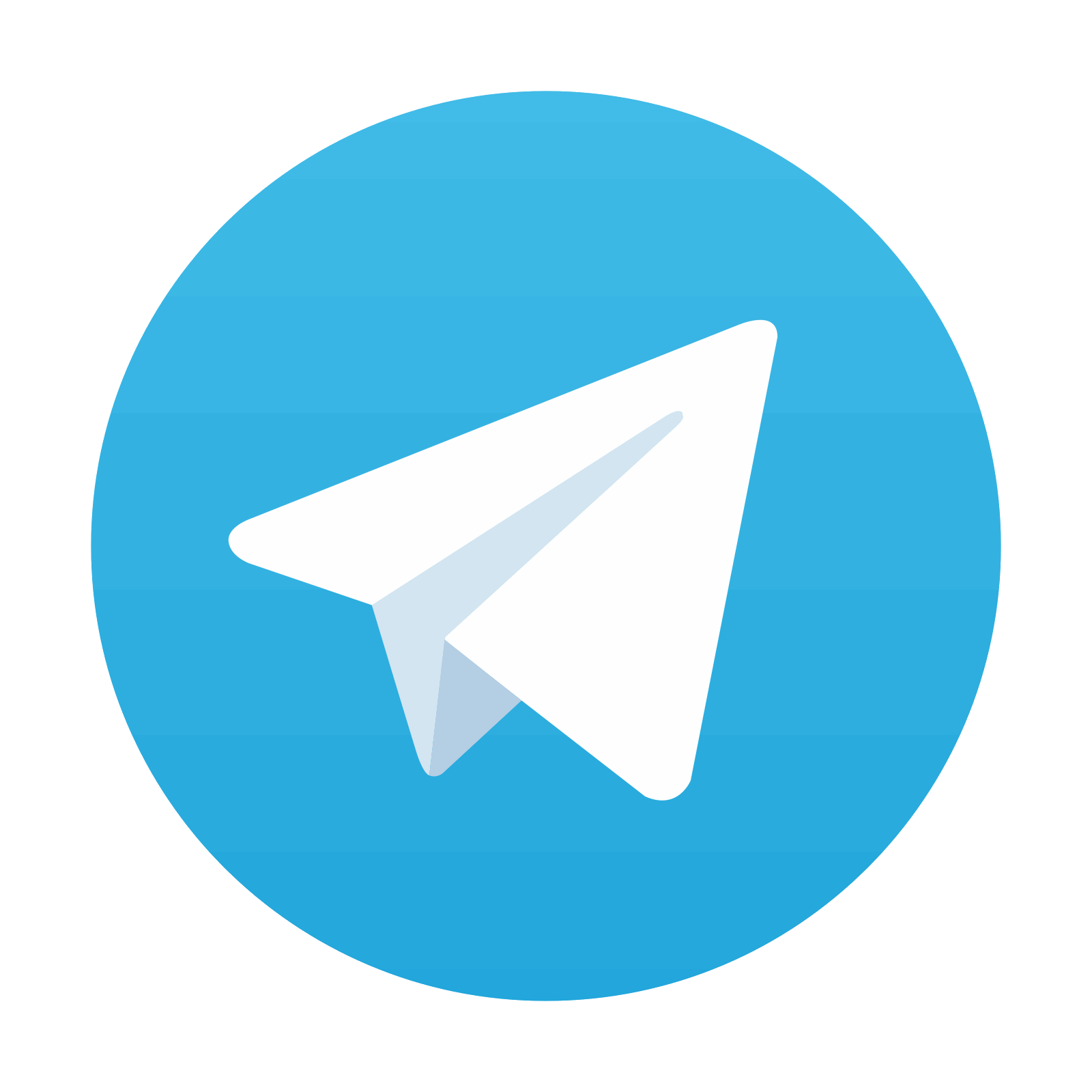
Stay updated, free articles. Join our Telegram channel

Full access? Get Clinical Tree
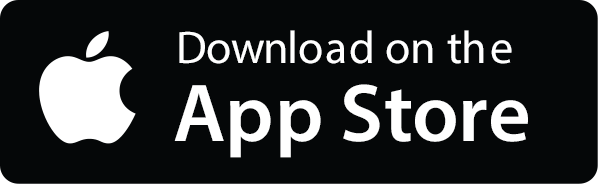
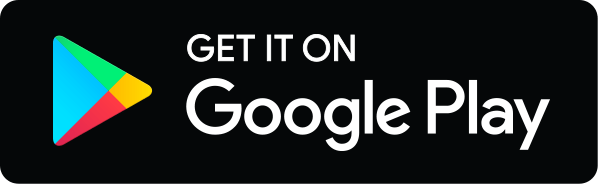