VIROLOGY
MORPHOLOGY AND BIOLOGIC FEATURES
As a group, the enteroviruses are picornaviruses that are extremely small (22-30 nm in diameter), naked capsid virions with icosahedral symmetry. They possess single-stranded, positive-sense RNA with a covalently bound small virus-encoded protein (VPg) and a capsid formed from 60 copies of four nonglycosylated proteins (VP1, VP2, VP3, and VP4). The basic building block of the capsid is the protomer containing one copy each of VP1, VP2, VP3, and VP4. Five protomers (pentamers) are placed at each of the 12 vertices of the icosahedron to form a capsomere of 60 protomers. The shell is composed of VP1, VP2, and VP3, whereas VP4 is attached on the inner surface. On the surface of the virus, there is a deep depression or canyon around each pentameric vertex. The receptor binding site is located at the floor of the canyon. The virion structure of a picornavirus member is shown in Chapter 13 (Figure 13–1). Replication and assembly occurs exclusively in the cellular cytoplasm; one infectious cycle can occur within 6 to 7 hours. This results in cessation of host cell protein synthesis and cell lysis with release of new infectious progeny. The replication cycle is shown in Figure 12–1. Picornaviruses enter the host cell via receptor-mediated endocytosis (viropexis) following interaction of a viral surface protein with a specific receptor on the host cell. Picornaviruses use a wide variety of host cell receptors, including PVR or CD155 (poliovirus), CD55 (coxsackieviruses, echoviruses, enterovirus 70), and ICM1 (some coxsackieviruses A). After the removal of capsid protein, uncoating takes place followed by removal of VPg, and the positive-sense RNA viral genome is released into the cytoplasm, which acts as an mRNA. This genomic viral mRNA is translated in a cap-independent manner using internal ribosomal entry site (IRES) into a polyprotein, which is processed into mature proteins, including an RNA-dependent RNA polymerase. RNA-dependent RNA polymerase directs both transcription of mRNA and synthesis of genomic RNA via negative-sense RNA intermediates. After the synthesis of viral proteins, the genomic RNA (+) is packaged into progeny virions that are assembled in the cytoplasm and released upon cell death.
FIGURE 12–1. Replication cycle of picornaviruses. Picornaviruses interact with a specific receptor on the host cell for entry via receptor-mediated endocytosis (viropexis). Following uncoating, VPg (viral protein genome) is removed and the positive-sense genomic RNA is released in the cytoplasm. The genomic RNA (+) is translated in a cap into a polyprotein, which is processed into mature proteins, including an RNA-dependent RNA polymerase. RNA-dependent RNA polymerase directs both transcription of mRNA and synthesis of genomic RNA via negative-sense RNA intermediates. After the synthesis of viral proteins, the genomic RNA (+) is packaged into progeny virions that are assembled in the cytoplasm and released upon cell death.
Small, naked capsid, single-stranded positive-sense RNA viruses
Replication and assembly take place in cytoplasm
Unlike rhinoviruses, which are also members of the picornavirus family, enteroviruses are resistant to an acidic pH (as low as 3.0). This feature undoubtedly helps ensure their survival during passage through the stomach to the intestines. Enteroviruses are also resistant to many common disinfectants such as 70% alcohol, substituted phenolics, ether, and various detergents that readily inactivate most enveloped viruses. Chemical agents, such as 0.3% formaldehyde or free residual chlorine at 0.3 to 0.5 ppm, are effective. However, if sufficient extraneous organic debris is present, the virus can be protected and survive long periods. Glutaraldehyde (2%, pH 7.4, temperature 25°C) can reduce the infectivity of the virus by 2log10 in less than 1 minute and was not negatively affected by the presence of high concentration of organic matters.
Resistant to acid, detergents, and many disinfectants
Formaldehyde and hypochlorite are active against enteroviruses
Some of the enterovirus serotypes share common antigens, but there are no significant serologic relationships between the currently recognized major classes listed in Table 12–1. Genetic variation within specific strains occurs, and mutants that exhibit antigenic drift and altered tropism for specific cell types are now recognized. Polioviruses, which have been most extensively studied as enterovirus prototypes, are known to have epitopes on three surface structural proteins (VP1, VP2, and VP3) that induce type-specific neutralizing antibodies. This appears to be generally the case for all enteroviruses; definitive identification of isolates usually requires neutralization or molecular analysis tests.
TABLE 12–1 Human Enteroviruses
Antigenic mutations and drifts occur
Antibody to surface proteins neutralize infectivity
GROWTH IN THE LABORATORY
Most enteroviruses can be isolated in primate (human or simian) cell cultures and show characteristic cytopathic effects. Some strains, particularly several coxsackievirus A serotypes, are more readily detected by inoculation of newborn mice. In fact, the newborn mouse is one basis for originally classifying group A and B coxsackieviruses. Group A coxsackieviruses cause primarily a widespread, inflammatory, necrotic effect on skeletal muscle, leading to flaccid paralysis and death. Similar inoculation of group B coxsackieviruses causes encephalitis, resulting in spasticity and occasionally convulsions. Other enteroviruses rarely have an adverse effect on mice unless special adaptation procedures are first used. The higher-numbered enteroviruses (types 68-71), which have overlapping variable growth and host characteristics, have been classified separately.
Growth of some in primate cell cultures
Coxsackie A and B viruses have different effects on newborn mice
ENTEROVIRUS DISEASE
EPIDEMIOLOGY
Humans are the major natural host for the polioviruses, coxsackieviruses, and echoviruses. There are enteroviruses of other animals with limited host ranges that do not appear to extend to humans. Conversely, viruses thought to be identical or related to human entero-viruses have been isolated from dogs and cats. Whether these agents cause disease in such animals is debatable, and there is no evidence of disease spreading from animals to humans.
Animals are not involved in human disease
The enteroviruses have a worldwide distribution, and asymptomatic infection is common. The proportion of infected persons who develop illness varies from 2% to 100%, depending on the serotype or strain involved and the age of the patient. Secondary infections in households are common and range as high as 40% to 70%, depending on factors such as family size, crowding, and sanitary conditions.
Proportion of asymptomatic infections varies with strain
In some years, certain serotypes emerge as dominant epidemic strains; they then may wane, only to reappear in epidemic fashion years later. For example, echovirus 16 was a major cause of outbreaks in the eastern United States in 1951 and 1974. Coxsackievirus B1 was common in 1963; echovirus 9 in 1962, 1965, 1968, and 1969; echovirus 13 and 18 in 2001; and echovirus 30 in 1968, 1969, between 1989 and 1992, and in 2003. The emergence of dominant serotypes is unpredictable from year to year. All enteroviruses show a seasonal predilection in temperate climates; epidemics are usually observed during the summer and fall months. In subtropical and tropical climates, the transmission may occur year-round.
Dominant epidemic strains come and go
Greater prevalence during summer and fall in temperate climates
Direct or indirect fecal–oral transmission is considered the most common mode of spread. After infection, the virus persists in the oropharynx for 1 to 4 weeks, and it can be shed in the feces for 1 to 18 weeks. The virus is present in respiratory secretions, including saliva, sputum, and nasal mucous as well as stool of infected people. Thus, sewage-contaminated water, fecally contaminated foods, or passive transmission by insect vectors (flies, cockroaches) may occasionally be the source of infection. More commonly, however, spread is directly from person-to-person. This mode of transmission is suggested by the high infection rates seen among young children, whose hygienic practices tend to be less than optimal, and in crowded households. Approximately two-thirds of all isolates are from children 9 years of age or younger. The risk of transmission is higher in those who do not have antibodies from previous infections, including during pregnancy. Mothers infected around the time of delivery can pass the virus to the infants.
Person-to-person fecal–oral transmission correlates with predominance in children
Incubation periods vary, but relatively short intervals (2-10 days) are common. Often, illness is seen concurrently in more than one family member, and the clinical features vary within the household.
Incubation periods are typically short
PATHOGENESIS
Initial binding of an enterovirus to the cell surface is commonly between an attachment protein in a “canyon” configuration on the virion surface and cell receptors belonging to the immunoglobulin gene superfamily. These receptors map to chromosome 19. A different receptor, belonging to the integrin group of adhesion molecules, has been identified for at least one echovirus serotype. After attachment, the virion is enveloped by the cell membrane, and its RNA is released into the cellular cytoplasm, where it binds to ribosomes and commences protein synthesis. Newly synthesized virions are released by lysis to spread to the other cells. Picornaviruses shut off host cell synthesis by destroying the cellular initiation factor complex that is required for protein synthesis and still favor its own protein synthesis by allowing ribosomes to bind onto IRES on viral RNA.
Initial attachment binds viral surface protein to cell surface receptors
Host receptor may relate to immunoglobulin or integrin families
After primary replication in epithelial cells and lymphoid tissues in the upper respiratory and gastrointestinal tracts, viremic spread to other sites can occur. Potential target organs vary according to the virus strain and its tropism, but may include the central nervous system (CNS), heart, vascular endothelium, liver, pancreas, lungs, gonads, skeletal muscles, synovial tissues, skin, and mucous membranes. Histopathologic findings include cell necrosis and mononuclear cell inflammatory infiltrates; in the CNS, the inflammatory cells are localized most prominently in perivascular sites. The initial tissue damage is thought to result from the lytic cycle of virus replication; secondary spread to other sites may ensue. Viremia is usually undetectable by the time symptoms appear, and termination of virus replication appears to correlate with the appearance of circulating neutralizing antibody, interferon, and mononuclear cell infiltration of infected tissue. The early dominant antibody response is with immunoglobulin M (IgM), which usually wanes 6 to 12 weeks after onset to be replaced progressively by increased IgG-specific antibodies. The important role of antibodies in termination of infection, demonstrated in mouse models of group B coxsackievirus infections, is supported by the observation of persistent echovirus and poliovirus replication in patients with antibody deficiency diseases.
Initial replication in epithelial and lymphoid cells is followed by viremic spread
Injury by cell lysis is localized in perivascular sites
Antibody response terminates replication
Although initial acute tissue damage may be caused by the lytic effects of the virus on the cell, the secondary sequelae may be immunologically mediated. Enterovirus-caused poliomyelitis, disseminated disease of the newborn, aseptic meningitis, encephalitis, and acute respiratory illnesses, thought to represent primary lytic infections, can usually be identified through routine methods of virus isolation and determination of specific antibody titer changes. On the other hand, syndromes such as myopericarditis, nephritis, and myositis have been associated with enteroviruses primarily because of serologic and epidemiologic evidence. In many of these cases, viral isolation is the exception rather than the rule. The pathogenesis of these latter infections is not clear; however, observations suggest that the acute infectious phase of the virus may be mild or subclinical and often subsides by the time clinical illness becomes evident. Illness may represent a host immunologic response to tissue injury by the virus or to viral or virus-induced antigens that persist in the affected tissues.
In experimental group B coxsackievirus myocarditis, mononuclear inflammatory cells (monocytes, natural killer lymphocytes) seem to play a greater role than antibody in termination of infection, and the persistence of inflammation after disappearance of detectable infectious virus or viral antigen appears to be mediated by cytotoxic T lymphocytes. Experimental findings have led to another hypothesis regarding pathogenic mechanisms, called molecular mimicry. This is best conceptualized as a form of virus-induced autoimmune response. It is known that small peptide sequences on viral epitopes can sometimes be shared by host tissues. Thus, an immune response produced by the virus may also generate antibodies or cytotoxic cross-reactive effector T lymphocytes that recognize shared determinants located on host cells. For example, a monoclonal antibody directed against a neutralizing site of a group B coxsackievirus has also been shown to react strongly with normal myocardial cells.
In addition to lytic effects of virus, there are probable immunopathologic manifestations
Disease may follow the acute infection
Coxsackie B myocarditis may involve virus-induced cross-reacting antibody
IMMUNITY
Infection by a specific serotype in an immunologically normal host is followed by a humoral antibody response, which can often be detected by neutralization methods for many years thereafter (Figure 12–2). There is relative immunity to reinfection by the same serotype; however, reinfection has been reported, usually resulting in subclinical infection or mild illness.
FIGURE 12–2. Antibody response and viral isolation from various sites in a typical case of enteroviral infection.
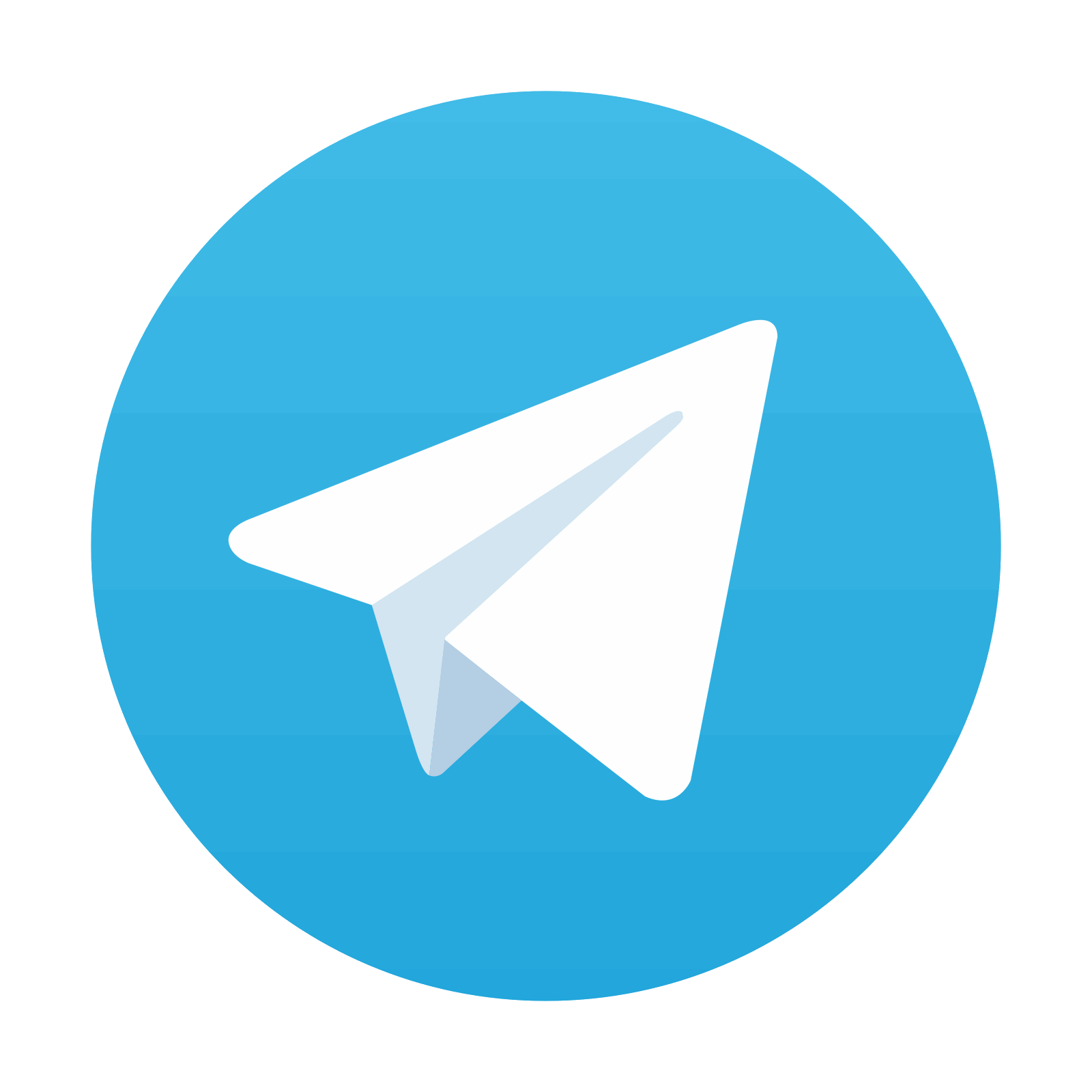
Stay updated, free articles. Join our Telegram channel

Full access? Get Clinical Tree
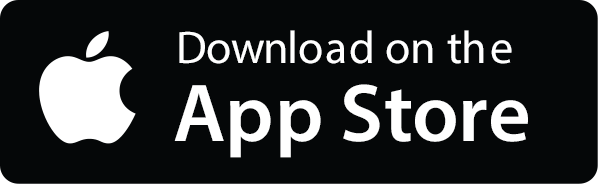
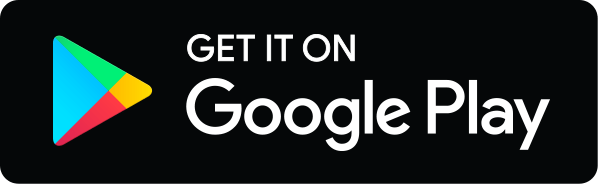