FIGURE 3–1. Kinetics of bacterial killing. A. Exponential killing is shown as a function of population size and time. B. Deviation from linearity, as with a mixed population, extends the time.
Bacterial killing follows exponential kinetics
Heterogeneous microbial subpopulations may extend the killing kinetics
STERILIZATION
The availability of reliable methods of sterilization has made possible the major developments in surgery and intrusive medical techniques that have helped to revolutionize medicine over the last century. Furthermore, sterilization procedures form the basis of many food preservation procedures, particularly in the canning industry. The various modes of sterilization described in the text are summarized in Table 3–1.
TABLE 3–1 Methods of Disinfection and Sterilization
Heat
The simplest method of sterilization is to expose the surface to be sterilized to a naked flame, as is done with the wire loop used in microbiology laboratories. It can be used equally effectively for emergency sterilization of a knife blade or a needle. Of course, disposable material is rapidly and effectively decontaminated by incineration. Carbonization of organic material and destruction of microorganisms, including spores, occur after exposure to dry heat of 160°C for 2 hours in a sterilizing oven. This method is applicable to metals, glassware, and some heat-resistant oils and waxes that are immiscible in water and, therefore, cannot be sterilized in the autoclave. A major use of the dry-heat sterilizing oven is in preparation of laboratory glassware.
Incineration is rapid and effective Dry heat requires 160°C for 2 hours to kill
Moist heat in the form of water or steam is far more rapid and effective in sterilization than dry heat because reactive water molecules denature protein irreversibly by disrupting hydrogen bonds between peptide groups at relatively low temperatures. Most vegetative bacteria are killed within a few minutes at 70°C or less, although many bacterial spores can resist boiling for prolonged periods.
Moisture allows for rapid denaturation of protein
Boiling water fails to kill bacterial spores
In effect, the autoclave is a sophisticated pressure cooker (Figure 3–2). In its simplest form, it consists of a chamber in which the air can be replaced with pure saturated steam under pressure. Air is removed either by evacuation of the chamber before filling it with steam or by displacement through a valve at the bottom of the autoclave, which remains open until all air has drained out. The latter, which is termed a downward displacement autoclave, capitalizes on the heaviness of air compared with saturated steam. When the air as been removed, the temperature in the chamber is proportional to the pressure of the steam; autoclaves are usually operated at 121°C. Under these conditions, spores directly exposed are killed in less than 5 minutes, although the normal sterilization time is 10 to 15 minutes to account for variation in the ability of steam to penetrate different materials and to allow a wide margin of safety.
FIGURE 3–2. Simple form of downward displacement autoclave. (Reproduced with permission from Willey JM: Prescott, Harley, & Klein’s Microbiology, 7th edition. McGraw-Hill, 2008.)
Autoclave creates increased temperature of steam under pressure
steam displaces air from the autoclave
The effectiveness of autoclaves depends on the absence of air, pure saturated steam, and access of steam to the material to be sterilized. Pressure per se plays no role in sterilization other than to ensure the increased temperature of the steam. “Flash” autoclaves, which are widely used in operating rooms, often use saturated steam at a temperature of 134°C for 3 minutes. Air and steam are removed mechanically before and after the sterilization cycle to ensure that metal instruments may be available rapidly.
Access of pure saturated steam is required for sterilization
Flash autoclaves use 134°C for 3 minutes
Gas
A number of articles, particularly certain plastics and lensed instruments that are damaged or destroyed by autoclaving, can be sterilized with gases. Ethylene oxide is an inflammable and potentially explosive gas. It is an alkylating agent that inactivates microorganisms by replacing labile hydrogen atoms on hydroxyl, carboxy, or sulfhydryl groups, particularly of guanine and adenine in DNA. Ethylene oxide sterilizers resemble autoclaves and expose the load to 10% ethylene oxide in carbon dioxide at 50 °C to 60 °C under controlled conditions of humidity. Exposure times, usually, are approximately 4 to 6 hours and must be followed by a prolonged period of aeration to allow the gas to diffuse out of substances that have absorbed it. Aeration is essential, because absorbed gas can cause damage to tissues or skin. Ethylene oxide is an effective sterilizing agent for heat-labile devices such as artificial heart valves that cannot be treated at the temperature of the autoclave. Other alkylating agents such as formaldehyde vapor can be used without pressure to decontaminate larger areas such as rooms.
Ethylene oxide sterilization is used for heat-labile materials
Aeration needed after ethylene oxide sterilization
Formaldehyde and oxidizing agents are useful in sterilization
Ultraviolet Light and Ionizing Radiation
Ultraviolet (UV) light in the wavelength range of 240 to 280 nm is absorbed by nucleic acids and causes genetic damage, including the formation of the thymine dimers discussed previously. The practical value of UV sterilization is limited by its poor ability to penetrate. Its main application has been in irradiation of air in the vicinity of critical hospital sites and as an aid in the decontamination of facilities used for handling particularly hazardous organisms.
UV light causes direct damage to DNA
Use of UV light is limited by penetration and safety
Ionizing radiation carries far greater energy than UV light. It, too, causes direct damage to DNA and produces toxic free radicals and hydrogen peroxide from water within the microbial cells. Cathode and gamma rays from cobalt-60 are widely used in industrial processes, including the sterilization of many disposable surgical spplies such as gloves, plastic syringes, specimen containers, some foodstuffs, and the like, because they can be packaged before exposure to the penetrating radiation.
Ionizing radiation damages DNA
Used for surgical spplies and food
DISINFECTION
Physical Methods
Filtration
Both live and dead microorganisms can be removed from liquids by positive- or negative-pressure filtration. Membrane filters, usually composed of cellulose esters (eg, cellulose acetate), are available commercially with variable pore sizes (0.005-1 μm). For removal of bacteria, a pore size of 0.2 μm is effective for disinfection of large volumes of fluid, especially fluid containing heat-labile components such as serum. Filtration is not considered effective for removing viruses.
Membrane filters remove bacteria
Pasteurization
Pasteurization involves exposure of liquids to temperatures in the range 55°C to 75°C to remove all vegetative bacteria. Spores are unaffected by the pasteurization process. Pasteurization is used commercially to render milk safe and to extend its storage quality. With the outbreaks of infection due to contamination with enterohemorrhagic E coli (see Chapter 33), this has been extended (reluctantly) to fruit drinks. To the dismay of some of his compatriots, Pasteur proposed application of the process to wine-making to prevent microbial spoilage and vinegarization. Pasteurization in water at 70°C for 30 minutes has been effective and inexpensive when used to render plastics, such as those used in inhalation therapy equipment, free of organisms that may, otherwise, multiply in mucus and humidifying water.
Kills vegetative bacteria but not spores
Used for foods and fragile medical equipment
Microwaves
The use of microwaves in the form of microwave ovens or specially designed units is another method of disinfection. These systems are not under pressure, but they but can achieve temperatures near boiling if moisture is present. In some situations, they are being used as a practical alternative to incineration for disinfection of hospital waste. These procedures cannot be considered sterilization only because heat-resistant spores may survive the process.
Microwaves kill by generating heat
Chemical Methods
Given access and sufficient time, chemical disinfectants cause the death of pathogenic vegetative bacteria. Most of these substances are general protoplasmic poisons and are not used in the treatment of infections other than very sperficial lesions, having been replaced by antimicrobial agents. Some disinfectants such as the quaternary ammonium compounds, alcohol, and the iodophors reduce the sperficial flora and can eliminate contaminating pathogenic bacteria from the skin surface. Other agents such as the phenolics are valuable only for treating inanimate surfaces or for rendering contaminated materials safe. All are bound and inactivated to varying degrees by protein and dirt, and they lose considerable activity when applied to other than clean surfaces.
Most agents are general protoplasmic poisons
Disinfectants are variably inactivated by organic material
Chemical disinfectants are classified on the basis of their ability to sterilize. High-level disinfectants kill all agents, except the most resistant of bacterial spores. Intermediate-level disinfectants kill all agents, but not spores. Low-level disinfectants are active against most vegetative bacteria and lipid-enveloped viruses.
Activity against spores and viruses varies
Alcohol
The alcohols are protein denaturants that rapidly kill vegetative bacteria when applied as aqueous solutions in the range of 70% to 95% alcohol. They are inactive against bacterial spores and many viruses. Solutions of 100% alcohol dehydrate organisms rapidly but fail to kill, because the lethal process requires water molecules. Ethanol (70-90%) and isopropyl alcohol (90-95%) are widely used as skin decontaminants before simple invasive procedures such as venipuncture. Their effect is not instantaneous, and the traditional alcohol wipe, particularly when followed by a vein-probing finger, is more symbolic than effective because insufficient time is given for significant killing. Isopropyl alcohol has largely replaced ethanol in hospital use because it is somewhat more active and is not subject to diversion to house/staff parties.
Alcohols require water for maximum effectiveness
Action of alcohol is slow
Halogens
Iodine is an effective disinfectant that acts by iodinating or oxidizing essential components of the microbial cell. Its original use was as a tincture of 2% iodine in 50% alcohol, which kills more rapidly and effectively than alcohol alone. Tincture of iodine has now been largely replaced by preparations in which iodine is combined with carriers (povidone) or nonionic detergents. These agents, termed iodophors, gradually release small amounts of iodine. They cause less skin staining and dehydration than tinctures, and are widely used in preparation of skin before surgery.
Tincture of iodine in alcohol is effective
Iodophors combine iodine with detergents
Chlorine exists as hypochlorous acid in aqueous solutions that dissociate to yield free chlorine over a wide pH range, particularly under slightly acidic conditions. In concentrations of less than one part per million, chlorine is lethal within seconds to most vegetative bacteria, and inactivates most viruses; this efficacy accounts for its use in rendering spplies of drinking water safe and in chlorination of water in swimming pools. Chlorine is the agent of choice for decontaminating surfaces and glassware that have been contaminated with viruses or spores of pathogenic bacteria. For these purposes, it is usually applied as a 5% solution called hypochlorite.
Chlorine oxidative action is rapid
Good for water and glassware
Hydrogen Peroxide
Hydrogen peroxide is a powerful oxidizing agent that attacks membrane lipids and other cell components. Although it acts rapidly against many bacteria and viruses, it kills bacteria that produce catalase and spores less rapidly. Hydrogen peroxide has been useful in disinfecting items such as contact lenses, which are not susceptible to its corrosive effect.
Hydrogen peroxide oxidizes cell components
Surface-Active Compounds
Surfactants are compounds with hydrophobic and hydrophilic groups that attach to and solubilize various compounds or alter their properties. Anionic detergents such as soaps are highly effective cleansers, but have little direct antibacterial effect, probably because their charge is similar to that of most microorganisms. Cationic detergents, particularly the quaternary ammonium compounds (“quats”) such as benzalkonium chloride, are highly bactericidal in the absence of contaminating organic matter. Their hydrophobic and lipophilic groups react with the lipid of the cell membrane of the bacteria, alter the membrane’s surface properties and its permeability, and lead to loss of essential cell components and death. These compounds have little toxicity to skin and mucous membranes and, thus, have been used widely for their antibacterial effects in a concentration of 0.1%. They are inactive against spores and most viruses. Care is needed in the use of quats because they adsorb to most surfaces as well as cotton, cork, and even dust. As a result, their concentration may be lowered to a point at which certain bacteria, particularly Pseudomonas aeruginosa, can grow in the quat solutions and serve as a source of infection.
Hydrophobic and hydrophilic groups of surfactants act on lipids of bacterial membranes
Little activity against viruses
Quats adsorb to surfaces and cotton
Phenolics
Phenol is a potent protein denaturant and bactericidal agent. Substitutions in the ring structure of phenol have substantially improved activity and have provided a range of phenols and cresols that are the most effective environmental decontaminants available for use in hospital hygiene. Concern about their release into the environment in hospital waste and sewage has created some pressure to limit their use. Phenolics are less “quenched” by protein than are most other disinfectants, have a detergent-like effect on the cell membrane, and are often formulated with soaps to increase their cleansing property. They are too toxic to skin and tissues to be used as antiseptics, although brief exposures can be tolerated. They are the active ingredient in many mouthwash and sore throat preparations.
Relatively stable to protein
Environmental contamination limits use
Chlorhexidine is used as a routine hand and skin disinfectant and for other topical applications. It has the ability to bind to the skin and produce a persistent antibacterial effect. It acts by altering membrane permeability of both Gram-positive and Gram-negative bacteria. It is cationic and, thus, its action is neutralized by soaps and anionic detergents.
Chlorhexidine persists in skin
Glutaraldehyde and Formaldehyde
Glutaraldehyde and formaldehyde are alkylating agents highly lethal to essentially all microorganisms (Figure 3–3). Formaldehyde gas is irritative, allergenic, and unpleasant—properties that limit its use as a solution or gas. Glutaraldehyde is an effective high-level disinfecting agent for apparatus that cannot be heat-treated, such as some lensed instruments and equipment for respiratory therapy. Formaldehyde vapor, an effective environmental decontaminant under conditions of high humidity, is sometimes used to decontaminate laboratory rooms that have been accidentally and extensively contaminated with pathogenic bacteria.
FIGURE 3–3. Action of glutaraldehyde. Glutaraldehyde polymerizes and then interacts with amino acids in proteins (left) or in bacterial peptidoglycan (right). As a result, they are alkylated and inactivated. (Reproduced with permission from Willey JM: Prescott, Harley, & Klein’s Microbiology, 7th edition. McGraw-Hill, 2008.)
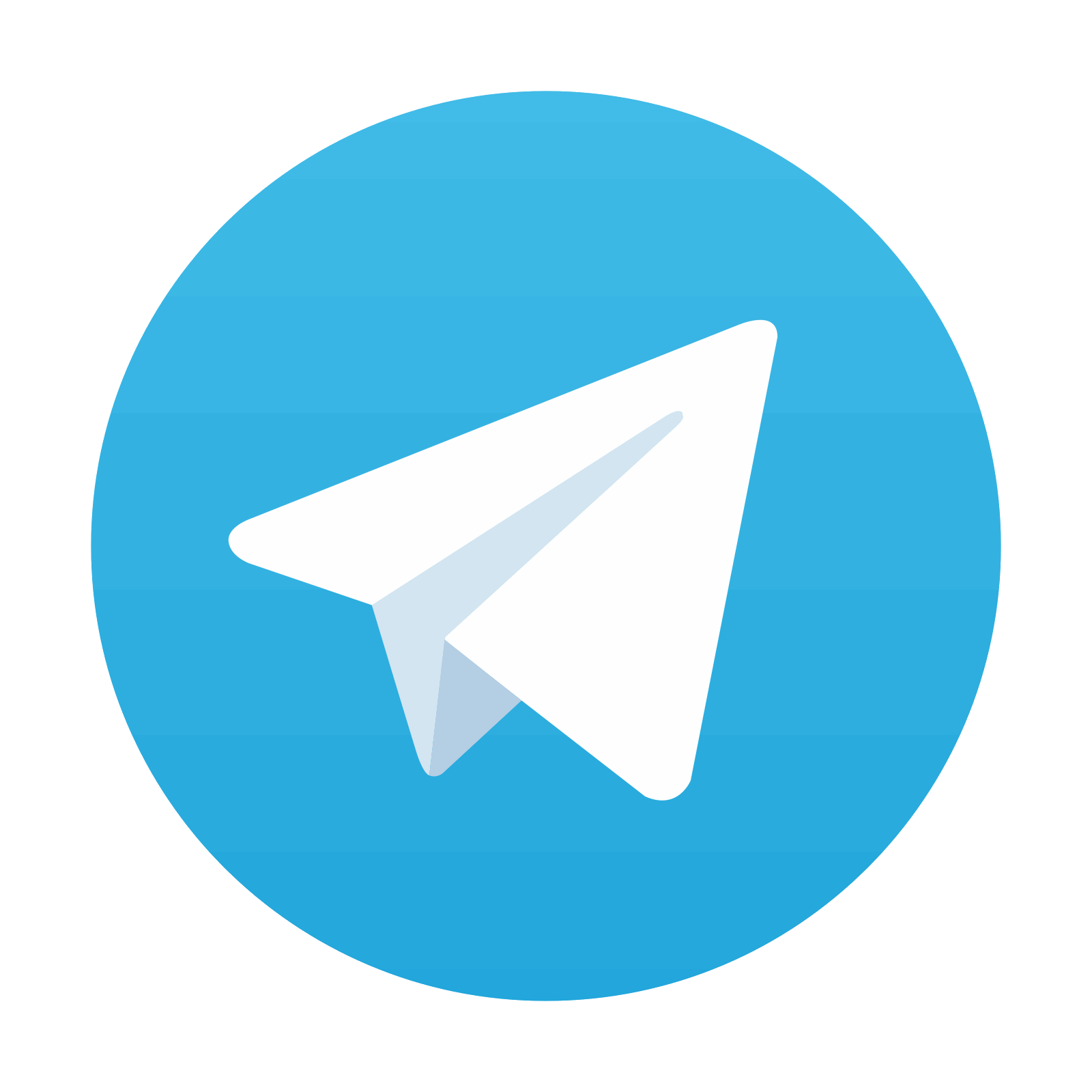
Stay updated, free articles. Join our Telegram channel

Full access? Get Clinical Tree
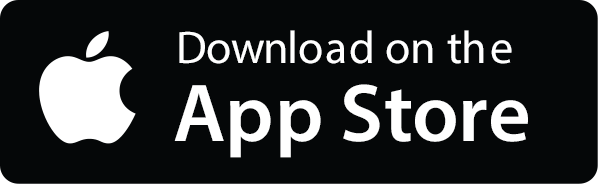
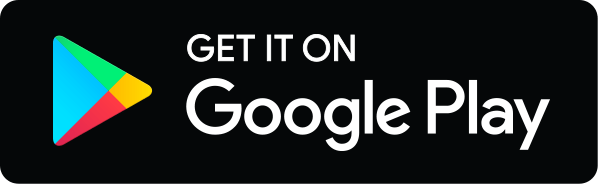