ARTHROPOD-BORNE ZOONOTIC ARBOVIRUSES
VIROLOGY
In most cases, the zoonotic viruses were first named after the place of initial isolation (eg, St. Louis encephalitis) or after the disease produced (eg, yellow fever). More recent studies have assigned the majority to families and genera on the basis of properties indicated in Table 16–1. The major characteristics of these arbovirus families, including togaviruses, flaviviruses, bunyaviruses, and reoviruses are summarized below.
Often named after place of initial isolation
TOGAVIRUSES
Togaviruses are from Togaviridae family and Alphavirus genus includes arboviruses within this family that infect humans. The other genus, Rubivirus that includes rubella virus is discussed in Chapter 10. Alphaviruses have enveloped virions containing single-stranded, positive-sense RNA genome measuring 70 nm in external diameter. The RNA genome is encapsidated in an icosahedral capsid that measures approximately 40 nm. The lipid bilayer envelope contains viral-encoded glycoproteins, E1 and E2. Alphaviruses have the ability to hemagglutinate via fusion of E1 glycoprotein to lipids in erythrocyte membrane and E2 also participates in this process. The structure of an alphavirus virion is shown in Figure 16–1. Replication occurs in the cytoplasm of the cells of infected arthropods and in vertebrate hosts. Virus enters via receptor-mediated endocytosis by interacting with a variety of cellular receptors, depending on the host and the cell type. The genomic RNA serves as the mRNA for the translation of nonstructural proteins, including RNA-dependent RNA polymerase. The RNA-dependent RNA polymerase synthesized negative-sense RNA intermediates, which is used for the synthesis of both subgenomic RNA (mRNA for synthesis of structural proteins) and new positive-sense, full-length genomic RNA. Virus assembly takes place in the cytoplasm. Virions mature by budding from cellular membranes. The effect of viral replication on invertebrate and vertebrate hosts is variable, with usually a persistent infection in invertebrate (arthropod) hosts. Viruses within the Alphavirus genus are frequently serologically related to one another but not to others. Representatives are listed in Table 16–1.
FIGURE 16–1. Virion structure of alphavirus. The single-stranded, positive-sense RNA genome is encapsidated into an icosahedral capsid (C protein) wrapped by a lipid bilayer envelope (viral membrane) containing viral-encoded glycoproteins (spikes), E1 and E2 with an external diameter of 70 nm. E1 has the ability to hemagglutinate via fusion to lipids on erythrocyte membrane and E2 also participates in this process.
Alphavirus genus of Togaviruses includes most arboviruses
Positive-sense RNA viruses that have icosahedral capsid and lipid bilayer envelope
Envelope contains glycoproteins that are hemagglutinin and lipoproteins
Replicates in the cytoplasm of the infected cells
Full-length RNA encodes nonstructural proteins and subgenomic RNA encodes structural proteins
Usually causes persistent infection in arthropods but acute infection in humans
FLAVIVIRUSES
Flaviviruses come from Flaviviridae family and Flavivirus genus that includes arboviruses transmitted through mosquitoes to humans. The other genus of Flaviviridae is Hepacivirus (hepatitis C virus) that is a blood-borne virus and causes hepatitis C (discussed in Chapter 13). Flaviviruses are similar to togaviruses in several respects such that they are positive-sense, single-stranded RNA, icosahedral capsid, enveloped viruses. However, the virions of flaviviruses are smaller than those of togaviruses, ranging from 40 to 50 nm in diameter. The RNA genome is surrounded by multiple copies of small basic proteins; the capsid (C) protein that covers the core and makes it icosahedral. The lipid bilayer envelope membrane contains the membrane (M) protein and envelope (E) protein, which is glycosylated in many flaviviruses. An example of a flavivirus virion is shown in Figure 16–2. The Flavivirus genus comprises most arboviruses within this family. Flavivirus members are serologically related, and there is cross-reactivity among members. The virus enters target cells via receptor-mediated endocytosis; flaviviruses can also bind to Fc receptors on macrophages, monocytes, and other cells coated with antibody. The enhancing antibody enhances viral adsorption and infectivity. The virus replicates like positive-sense RNA viruses, and the whole positive-sense RNA genome is translated into a polyprotein (like picornaviruses), which is cleaved into individual mature proteins, including a protease, an RNA-dependent RNA polymerase, a capsid and envelope proteins. Virus assembly takes place in the cytoplasm and the envelope is acquired by budding into intracellular vesicles and released upon cell lysis. Like alphaviruses, flaviviruses also cause a lytic response in vertebrate hosts and a persistent infection in invertebrate hosts.
FIGURE 16–2. Virion structure of flavivirus. Two types of virions, intracellular and extracellular virions, are shown. The positive-sense, single-stranded RNA genome is packaged into an icosahedral capsid wrapped into a lipid bilayer envelope containing membrane (M) protein and spike glycoprotein (E). The prM is the precursor to M protein. The size of flavivirus virion ranges from 40 to 50 nm in diameter There are two major differences between intracellular and extracellular virions; intracellular virions have only prM and E as monomer, whereas extracellular virions have prM and M and E as dimer
Flavivirus genus comprises arboviruses
Enveloped, positive-sense RNA, icosahedral capsid viruses
Replicates in the cytoplasm
Genomic RNA translated into a polyprotein that is cleaved into individual proteins
Lytic infection in vertebrates and persistent infection in invertebrates
BUNYAVIRUSES
There are four genera of Bunyaviridae family: Bunyavirus (–) RNA, Phlebovirus (–) RNA, Nairovirus (+/–) ambisense RNA, and Hantavirus (–) RNA. All bunyaviruses are arboviruses, except Hantavirus, which is a nonarthropod zoonotic virus and discussed in the next section. Bunyaviruses are spherical, enveloped, single-stranded, negative-sense RNA viruses approximately 90 to 100 nm in external diameter. The envelope contains two glycoproteins, G1 and G2, and encloses three helical nucleocapsids containing RNA, namely, large (L), medium (M), and small (S), associated with an RNA-dependent RNA polymerase (L) and nonstructural proteins (N) (Figure 16–3). Unlike enveloped RNA viruses, bunyaviruses are devoid of a matrix protein. The viral attachment protein (G1) interacts with cellular receptors, and the virus enters the cell via receptor-mediated endocytosis. After lysis of endosomal vesicles and release of the nucleocapsids in the cytoplasm, the negative RNA strands (L, M, S) transcribe to synthesize mRNA using virion-associated RNA-dependent RNA polymerase. The M strand encodes G1 and G2 envelope, a nonstructural protein; L strand encodes the L protein (RNA-dependent RNA polymerase); and the S strand encodes the nucleocapsid protein (NP) and a nonstructural protein. They mature by budding into smooth-surfaced vesicles in or near the Golgi region of the infected cell. The major disease-causing bunyaviruses in North America are California virus (arbovirus) and hantavirus (nonarthropod zoonotic virus).
FIGURE 16–3. Bunyavirus virion structure. The virions of bunyaviruses contain single-stranded, negative-sense RNA viruses that are spherical and enveloped with an external diameter of 90 to 100 nm. The envelope contains two glycoproteins, G1 and G2, and encloses three helical nucleocapsids containing RNA, namely large (L), medium (M), and small (S), associated with an RNA-dependent RNA polymerase (L) and nonstructural proteins (N).
Four genera, three arboviruses and one nonarthropod zoonotic virus
Spherical enveloped RNA viruses
Three helical nucleocapsids containing RNA: large (L), medium (M), and small (S)
Replicates in the cytoplasm; ambisense RNA uses negative-sense RNA strategies for replication
M segment encodes the envelope glycoproteins (G1 and G2), L segment encodes the viral RNA polymerase, and S segment encodes the nucleocapsid (N) protein
REOVIRUSES
Reoviruses are spherical, naked capsid icosahedral, double-stranded RNA viruses that measure about 80 nm in diameter with a segmented genome. The details about virus structure and replication of another member of the Reoviridae family, Rotavirus, are described in Chapter 15. The double-stranded segmented genome of reoviruses replicate in the cytoplasm by utilizing the negative-stranded RNA of the double strand for transcription and replication using their own virion-associated RNA-dependent RNA polymerase. However, the reoviruses described here are arboviruses that are transmitted through insect bites. The most important North American arbovirus of this family, which is a member of the genus Coltivirus, causes Colorado tick fever in humans. The other arboviruses from the Reoviridae family are Orbivirus which includes African horse sickness and bluetongue viruses, mainly causing disease in animals.
Colorado tick fever is prominent in North America
Colorado tick fever is the only reovirus transmitted by ticks to humans
Naked capsid, double-stranded RNA viruses replicate in the cytoplasm
ARBOVIRUS DISEASE
EPIDEMIOLOGY
Arboviruses of major importance in human disease are listed in Table 16–1 with summaries of their geographic distribution, the arthropod vectors that transmit them, and the usual disease syndromes that can result from infection.
With the exception of urban dengue and urban yellow fever, in which the virus may simply be transmitted between humans and mosquitoes, other arboviral diseases involve nonhuman vertebrates. These are usually small mammals, birds, or, in the case of jungle yellow fever, monkeys. Infection is transmitted within the host species by arthropods (eg, mosquitoes or ticks) that become infected. In some cases, the infection can be maintained from generation to generation in the arthropod by transovarial transmission. Infection in the arthropod usually does not appear to harm the insect; however, a period of virus multiplication (termed extrinsic incubation period) is required to enhance the capacity to transmit infection to vertebrates by bite.
The consequences of infection transmitted from the arthropod to susceptible vertebrate hosts are variable; some develop illness of varying severity with viremia, whereas others have long-term viremia without clinical disease. Vertebrate hosts are then a source of further spread of the virus by amplification, in which noninfected arthropods feeding on viremic hosts acquire the virus, thereby increasing the risk of transmission. The general features of this overall transmission cycle are illustrated in Figure 16–4.
FIGURE 16–4. General features of arbovirus transmission cycles, including urban (mosquito–human–mosquito), sylvatic (birds/monkey/small mammals–mosquito–human), and arthropod sustained (small mammals–tick–tick human). (reprinted from the Centers for Disease Control and prevention.)
Sometimes maintained by vertical transmission in vector
Multiplication in vector is required
Transient viremia is a feature of many of these infections in hosts other than their reservoir; those affected, including humans and higher vertebrates (eg, horses and cattle), are often referred to as blind-end hosts. In contrast, if viremia is sustained for longer periods (eg, weeks to months in a variety of togavirus, flavivirus, and bunyavirus infections of lower vertebrates), the vertebrate host becomes highly important as a reservoir for continuing transmission. Viremia may last a week or more in human dengue and yellow fever infections, and humans may then serve as a reservoir in urban disease.
Sustained viremia required for vertebrate host to be significant reservoir
Obviously, the typical arthropod vectors are rarely present during all seasons. The question then arises as to how the arboviruses survive between the time the vector disappears and the time it reappears in subsequent years. Several mechanisms can operate to sustain the virus between transmission periods (often referred to as overwintering): (1) sustained viremia in lower vertebrates such as small mammals, birds, and snakes, from which newly mature arthropods can be infected when taking a blood meal; (2) hibernation of infected adult arthropods that survive from one season to the next; and (3) transovarial transmission, whereby the infected female arthropod can transmit virus to its progeny.
Season-to-season survival has multiple mechanisms
The three basic specific cycles of arbovirus transmission are urban, sylvatic, and arthropod sustained.
Urban
As the term suggests, the urban cycle is favored by the presence of relatively large numbers of humans living in close proximity to arthropod (usually mosquito) species capable of virus transmission. The cycle is:
Examples of the urban cycle include urban dengue, urban yellow fever, and occasional urban outbreaks of St. Louis encephalitis.
Urban cycle exists with dengue and yellow fever
Sylvatic
In the sylvatic cycle, a single nonhuman vertebrate reservoir may be involved.
In this situation, the human, who becomes a tangential host through accidental intrusion into a zoonotic transmission cycle, is not important in maintaining the infection cycle. An example of this cycle is jungle yellow fever.
In other sylvatic cycles, multiple vertebrate reservoirs may be involved:
Examples include western equine encephalitis, eastern equine encephalitis, and California viruses. In some situations, such as St. Louis encephalitis and yellow fever, the urban and sylvatic cycles may operate concurrently.
Sylvatic cycle occurs with many viruses
Humans are tangential hosts
Arthropod Sustained
Arthropods, especially ticks, may sustain the reservoir by transovarial transmission of virus to their progeny, with amplification of the cycle by spread to and from small mammals:
Tick-borne encephalitis in Russia is transmitted by the arthropod-sustained cycle. In temperate climates such as the United States, arboviruses are major causes of disease during the summer and early fall months, the seasons of greatest activity of arthropod vectors (usually mosquitoes or ticks). When climatic conditions and ecologic circumstances (eg, swamps and ponds) are optimal for arthropod breeding and egg hatching, arbovirus amplification may begin.
Arthropod sustained by tick transovarial transmission
Weather, swamps, and ponds alter conditions
An example of amplification is provided by western equine encephalitis. When the mosquito vectors become abundant, the level of transmission among the basic reservoir hosts (birds and small mammals) increases, and the mosquitoes also turn to other susceptible species such as the domestic fowl. These hosts experience a rapidly developing asymptomatic viremia, which permits still more arthropods to become infected on biting. At this point, spread to blind-end hosts such as humans or horses and the development of clinical disease become likely. This occurrence depends on the accessibility of the host to the infected mosquito and on mosquito feeding preferences which, for unknown reasons, vary from one season to another.
Mosquito increases create risk for blind-end human infection
PATHOGENESIS
There are three major manifestations of arbovirus diseases in humans associated with different tropisms of various viruses for human organs, although overlap can occur. In some, the central nervous system (CNS) is primarily affected, leading to aseptic meningitis or meningoencephalitis. A second syndrome involves many major organ systems, with particular damage to the liver, as in yellow fever. The third syndrome is manifested by hemorrhagic fever, in which damage is particularly severe to the small blood vessels, with skin petechiae and intestinal and other hemorrhages.
CNS, visceral, and hemorrhagic fever are major syndromes
Infection of the human by a biting, infected arthropod is followed by viremia, which is apparently amplified by extensive virus replication in the reticuloendothelial system and vascular endothelium. After replication, the virus becomes localized in various target organs, depending on its tropism, and illness results. The viruses produce cell necrosis with resultant inflammation, which leads to fever in nearly all infections. If the major viral tropism is for the CNS, virus reaching this site by crossing the blood–brain barrier or along neural pathways can cause meningeal inflammation (aseptic meningitis) or neuronal dysfunction (encephalitis). The CNS pathology consists of meningeal and perivascular mononuclear cell infiltrates, degeneration of neurons with neuronophagia, and occasionally destruction of the supporting structure of neurons.
After bite, viremia and viral tissue tropism define disease
In CNS, aseptic meningitis and encephalitis follow cell injury
In some infections, especially yellow fever, the liver is the primary target organ. Pathologic findings include hyaline necrosis of hepatocytes, which produces cytoplasmic eosinophilic masses called Councilman bodies. Degenerative changes in the renal tubules and myocardium may also be seen, as may microscopic hemorrhages throughout the brain. Hemorrhage is a major feature of yellow fever, largely because of the lack of liver-produced clotting factors as a result of liver necrosis.
Liver often the target, with necrosis of hepatocytes
Hemorrhagic fevers other than those related to primary hepatic destruction have a somewhat different pathogenesis, which has been studied most extensively in dengue infections. In uncomplicated dengue fever, which is associated with a rash and influenza-like symptoms, there are changes in the small dermal blood vessels. These alterations include endothelial cell swelling and perivascular edema with mononuclear cell infiltration. More severe infection, as in dengue hemorrhagic fever, often complicated by shock, is characterized by perivascular edema and widespread effusions into serous cavities such as the pleura and by hemorrhages. The spleen and lymph nodes show hyperplasia of lymphoid and plasma cell elements, and there is focal necrosis in the liver. The pathophysiology seems related to increased vascular permeability and disseminated intravascular coagulation, which is further complicated by liver and bone marrow dysfunction (eg, decreased platelet production and decreased production of liver-dependent clotting factors). The major vascular abnormalities may be provoked by circulating virus–antibody complexes (immune complexes), which mediate activation of complement and subsequent release of vasoactive amines. The precise reason for this phenomenon is not clear; it may be related to intrinsic virulence of the virus strains involved and to host susceptibility factors.
Dengue hemorrhagic fevers involve perivascular and endothelial injury
May progress to shock
Lymphoid hyperplasia seen
Virus–antibody complexes may trigger complement activation
Two hypotheses are based on the existence of four distinct but antigenically related serotypes of dengue virus, any of which can generate group-specific cross-reacting antibodies that are not necessarily protective against other serotypes. One possibility is that preexisting group-specific antibody at a critical concentration serves as “enhancing” rather than neutralizing antibody. In the presence of enhancing antibody, virus-antibody complexes are more efficiently adsorbed to and engulfed by monocytes and macrophages. Subsequent replication leads to extensive spread throughout the host. Alternatively, or in concert with this, activation of previously sensitized T cells by viral antigen present on the surfaces of macrophages may result in release of cytokines, which mediate the development of shock and hemorrhage.
Cross-reacting antibodies may enhance infection
IMMUNITY
The usual humoral responses (hemagglutination inhibition, IgM, neutralization) in relation to onset of illness are illustrated in Figure 16–5. The rise in antibody titer generally correlates with recovery from infection. Neutralizing antibodies, which are the most sero-type-specific, generally persist many years after infection. The presence of IgM-specific antibodies indicates that primary infection likely occurred within the previous 2 months. Cellular immunity and humoral immunity to reinfection are serotype specific and appear to be permanent.
FIGURE 16–5. Typical patterns of antibody response after arbovirus infection. These patterns begin to appear about 3 days after onset and decline after about 6 weeks. HI, hemagglutination inhibition antibodies; IgM, immunoglobulin M antibodies.
Neutralizing antibodies protective and last for years
Immunity is serotype specific
SPECIFIC ARBOVIRUSES
Western Equine Encephalitis
Western equine encephalitis virus (Alphavirus/Togavirus) causes western equine encephalitis that is prevalent in the central valley of California, eastern Washington (Yakima valley), Colorado, and Texas. It has also been responsible for outbreaks in midwestern states (Minnesota, Wisconsin, Illinois, Missouri, and Kansas) and as far east as New Jersey. The virus is transmitted through mosquito (Culex tarsalis) bites. Horses and humans represent blind-end hosts; both are susceptible to infection and illness, commonly manifested as encephalitis. Although human infection in endemic areas is commonplace, overall only 1 of 1000 infections causes clinical symptoms. However, in young infants, 1 of every 25 infections may produce severe illness. The attack rates are therefore far higher in young infants than in other groups. The disease spectrum may range from mild, nonspecific febrile illness to aseptic meningitis or severe, overwhelming encephalitis. Mortality rate is estimated at 5% for cases of encephalitis. It is a very serious disease in infants less than 1 year of age; as many as 60% of survivors have permanent neurologic impairment.
Human and equine illness
Prevalent in the Western United States
Outbreaks in the Midwestern United States
Encephalitis is more likely in young infants
Eastern Equine Encephalitis
The eastern equine encephalitis virus (Alphavirus/Togavirus) is largely confined to the Atlantic Seaboard states from New England down the coasts of Central America and South America. The mosquito vector (principally Culiseta melanura) generally restricts its feeding to horses and birds, although occasional outbreaks among humans have occurred. Increasing numbers of human infections have been observed in 2012, which is a cause of concern. The virus can cause severe encephalitis in horses and also in wild birds. The mortality rate for eastern equine encephalitis among humans is estimated at 33% to 50% for individuals of all ages, and the incidence of severe sequelae among survivors is high.
New England to South America
Mosquito vector feeds on horses and birds
Occasional outbreaks in humans with encephalitis in all ages
St. Louis Encephalitis
The St. Louis encephalitis virus (Flavivirus) is a major cause of arbovirus encephalitis in the United States. Its major mosquito vector is C tarsalis similar to those of western equine encephalitis, but St. Louis encephalitis has been much more prevalent in eastern states and in Texas, Mississippi, and Florida. It infects but causes no disease in horses. The disease spectrum in humans is similar to that of western equine encephalitis, but the major morbidity and mortality, as well as the highest attack rates, are among adults more than 40 years of age. Infants and young children are relatively spared.
Major cause of arboviral encephalitis in the United States
Prevalent in eastern and southern United States
Highest attack rates among adults above the age of 40 years
West Nile Virus
West Nile virus (WNV), a member of flaviviruses, was first detected in 1937 in Uganda, Africa. During the summer of 1999 in the Northeastern United States, human WNV infections appeared for the first time in the Western Hemisphere. A subsequent outbreak occurred again in 2000. Together, these outbreaks resulted in 78 hospitalized patients and 9 deaths, mostly among the elderly. More widespread activity was observed in 2001 (66 human cases); then in 2002, a dramatic increase in virus spread was seen across the United States, with activity in 46 states and 4 Canadian provinces. Now, the virus has been detected in all states in the continental United States except Alaska (Figure 16–6). It is currently the most widespread arbovirus in North America. Before 1999, outbreaks of human WNV infections were primarily confined to eastern Africa, the Middle East, eastern Europe, west Asia, and Australia. Now it is distributed throughout Africa, the Middle East, parts of Europe, the former USSR, India, and Indonesia.
FIGURE 16–6. West Nile virus activity in the United States.
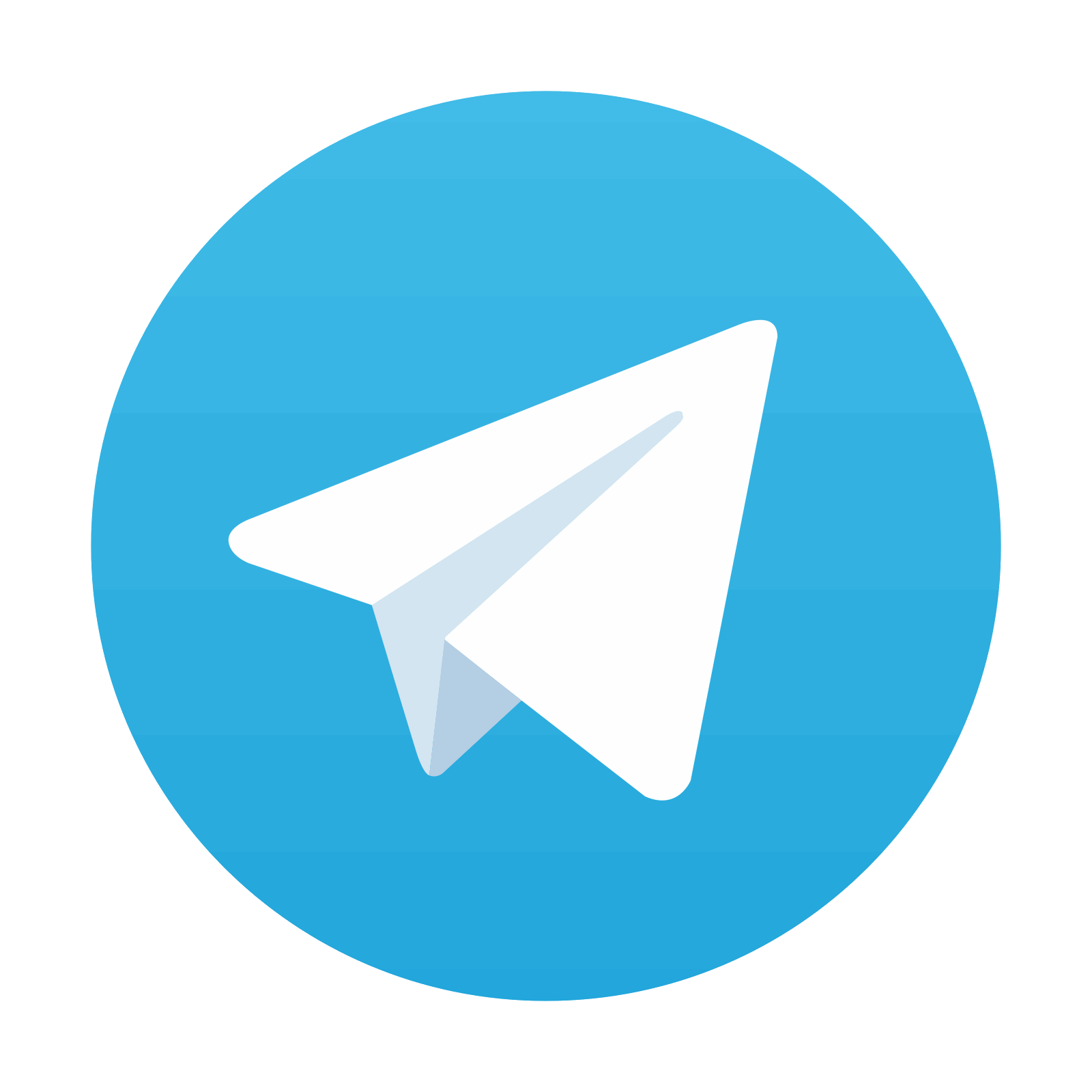
Stay updated, free articles. Join our Telegram channel

Full access? Get Clinical Tree
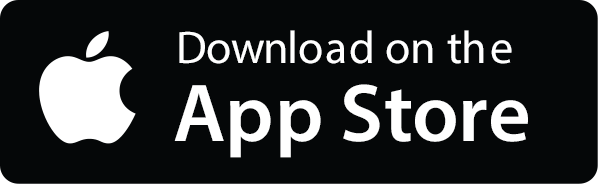
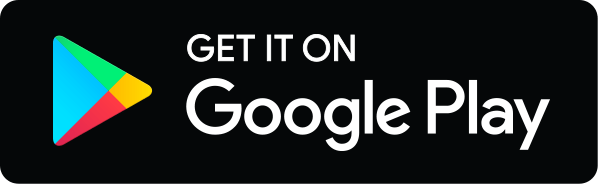