FIGURE 1–1. Death rates for infectious disease in the United States in the 20th century. Note the steady decline in death rates related to the introduction of public health, immunization, and antimicrobial interventions.
A new uneasiness that is part evolutionary, part discovery, and part diabolic has taken hold. Infectious agents once conquered have shown resistance to established therapy, such as multiresistant Mycobacterium tuberculosis, and diseases, such as acquired immunodeficiency syndrome (AIDS), have emerged. The spectrum of infection has widened, with discoveries that organisms earlier thought to be harmless can cause disease under certain circumstances. Who could have guessed that Helicobacter pylori, not even mentioned in the first edition of this book (1984), would be the major cause of gastric and duodenal ulcers and an officially declared carcinogen? Finally, bioterrorist forces have unearthed two previously controlled infectious diseases—anthrax and smallpox—and threatened their distribution as agents of biological warfare. For students of medicine, understanding the fundamental basis of infectious diseases has more relevance than ever.
BACKGROUND
The science of medical microbiology dates back to the pioneering studies of Pasteur and Koch, who isolated specific agents and proved that they could cause disease by introducing the experimental method. The methods they developed lead to the first golden age of microbiology (1875-1910), when many bacterial diseases and the organisms responsible for them were defined. These efforts, combined with work begun by Semmelweis and Lister, which showed how these diseases spread, led to the great advances in public health that initiated the decline in disease and death. In the first half of the 20th century, scientists studied the structure, physiology, and genetics of microbes in detail and began to answer questions relating to the links between specific microbial properties and disease. By the end of the 20th century, the sciences of molecular biology, genetics, genomics, and proteomics extended these insights to the molecular level. Genetic advances have reached the point at which it is possible to know not only the genes involved but also to understand how they are regulated. The discoveries of penicillin by Fleming in 1929 and of sulfonamides by Domagk in 1935 opened the way to great developments in chemotherapy. These gradually extended from bacterial diseases to fungal, parasitic, and finally viral infections. Almost as quickly, virtually all categories of infectious agents developed resistance to all categories of antimicrobial agents to counter these chemotherapeutic agents.
INFECTIOUS AGENTS: THE MICROBIAL WORLD
Microbiology is a science defined by smallness. Its creation was made possible by the invention of the microscope (Gr. micro, small + skop, to look, see), which allowed visualization of structures too small to see with the naked eye. This definition of microbiology as the study of microscopic living forms still holds if one can accept that some organisms can live only in other cells (eg, all viruses and some bacteria) and that others include macroscopic forms in their life cycle (eg, fungal molds, parasitic worms). The relative sizes of some microorganisms are shown in Figure 1–2.
FIGURE 1–2. Relative size of microorganisms.
Microbes are small
Microorganisms are responsible for much of the breakdown and natural recycling of organic material in the environment. Some synthesize nitrogen-containing compounds that contribute to the nutrition of living things that lack this ability; others (oceanic algae) contribute to the atmosphere by producing oxygen through photosynthesis. Because micro-organisms have an astounding range of metabolic and energy-yielding abilities, some can exist under conditions that are lethal to other life forms. For example, some bacteria can oxidize inorganic compounds such as sulfur and ammonium ions to generate energy. Others can survive and multiply in hot springs at temperatures higher than 75°C.
Most play benign roles in the environment
Some microbial species have adapted to a symbiotic relationship with higher forms of life. For example, bacteria that can fix atmospheric nitrogen colonize root systems of legumes and of a few trees, such as alders, and provide the plants with their nitrogen requirements. When these plants die or are plowed under, the fertility of the soil is enhanced by nitrogenous compounds originally derived from the metabolism of the bacteria. Ruminants can use grasses as their prime source of nutrition, because the abundant flora of anaerobic bacteria in the rumen break down cellulose and other plant compounds to usable carbohydrates and amino acids and synthesize essential nutrients including some amino acids and vitamins. These few examples illustrate the protean nature of microbial life and their essential place in our ecosystem.
Products of microbes contribute to the atmosphere
The major classes of microorganisms in terms of ascending size and complexity are viruses, bacteria, fungi, and parasites. Parasites exist as single or multicellular structures with the same compartmentalized eukaryotic cell plan of our own cells including a nucleus and cytoplasmic organelles like mitochondria. Fungi are also eukaryotic, but have a rigid external wall that makes them seem more like plants than animals. Bacteria also have a cell wall, but with a cell plan called “prokaryotic” that lacks the organelles of eukaryotic cells. Viruses are not cells at all. They have a genome and some structural elements, but must take over the machinery of another living cell (eukaryotic or prokaryotic) to replicate. The four classes of infectious agents are summarized in Table 1–1, and generic examples of each are shown in Figure 1–3.
TABLE 1–1 Features of Infectious Agents
FIGURE 1–3. Infectious agents. A. Virus. B. Bacterium. C. Fungus. D. Parasite. (Reproduced with permission from Willey JM: Prescott, Harley, & Klein’s Microbiology, 7th edition. McGraw-hill, 2008.)
Increasing complexity: viruses → bacteria → fungi → parasites
VIRUSES
Viruses are strict intracellular parasites of other living cells, not only of mammalian and plant cells, but also of simple unicellular organisms, including bacteria (the bacteriophages). Viruses are simple forms of replicating, biologically active particles that carry genetic information in either DNA or RNA molecules. Most mature viruses have a protein coat over their nucleic acid and, sometimes, a lipid surface membrane derived from the cell they infect. Because viruses lack the protein-synthesizing enzymes and structural apparatus necessary for their own replication, they bear essentially no resemblance to a true eukaryotic or prokaryotic cell.
Viruses contain little more than DNA or RNA
Viruses replicate by using their own genes to direct the metabolic activities of the cell they infect to bring about the synthesis and reassembly of their component parts. A cell infected with a single viral particle may, thus, yield thousands of viral particles, which can be assembled almost simultaneously under the direction of the viral nucleic acid. Infection of other cells by the newly formed viruses occurs either by seeding from or lysis of the infected cells. Sometimes, viral and cell reproduction proceed simultaneously without cell death, although cell physiology may be affected. The close association of the virus with the cell sometimes results in the integration of viral nucleic acid into the functional nucleic acid of the cell, producing a latent infection that can be transmitted intact to the progeny of the cell.
Replication is by control of the host cell metabolic machinery
Some integrate into the genome
BACTERIA
Bacteria are the smallest (0.1–10 μm) independently living cells. They have a cytoplasmic membrane surrounded by a cell wall; a unique interwoven polymer called peptidoglycan makes the wall rigid. The simple prokaryotic cell plan includes no mitochondria, lysosomes, endoplasmic reticulum, or other organelles (Table 1–2). In fact, most bacteria are approximately the size of mitochondria. Their cytoplasm contains only ribosomes and a single, double-stranded DNA chromosome. Bacteria have no nucleus, but all the chemical elements of nucleic acid and protein synthesis are present. Although their nutritional requirements vary greatly, most bacteria are free living if given an appropriate energy source. Tiny metabolic factories, they divide by binary fission and can be grown in artificial culture, often in less than 1 day. The Archaea are similar to bacteria but evolutionarily distinct. They are prokaryotic, but differ in the chemical structure of their cell walls and other features. The Archaea (archebacteria) can live in environments humans consider hostile (eg, hot springs, high salt areas) but are not associated with disease.
TABLE 1–2 Distinctive Features of Prokaryotic and Eukaryotic Cells
Smallest living cells
Prokaryotic cell plan lacks nucleus and organelles
FUNGI
Fungi exist in either yeast or mold forms. The smallest of yeasts are similar in size to bacteria, but most are larger (2–12 μm) and multiply by budding. Molds form tubular extensions called hyphae, which, when linked together in a branched network, form the fuzzy structure seen on neglected bread slices. Fungi are eukaryotic, and both yeasts and molds have a rigid external cell wall composed of their own unique polymers, called glucan, mannan, and chitin. Their genome may exist in a diploid or haploid state and replicate by meiosis or simple mitosis. Most fungi are free living and widely distributed in nature. Generally, fungi grow more slowly than bacteria, although their growth rates sometimes overlap.
Yeasts and molds are surrounded by cell wall
PARASITES
Parasites are the most diverse of all microorganisms. They range from unicellular amoebas of 10 to 12 μm to multicellular tapeworms 1 m long. The individual cell plan is eukaryotic, but organisms such as worms are highly differentiated and have their own organ systems. Most worms have a microscopic egg or larval stage, and part of their life cycle may involve multiple vertebrate and invertebrate hosts. Most parasites are free living, but some depend on combinations of animal, arthropod, or crustacean hosts for their survival.
Range from tiny amoebas to meter-long worms
THE HUMAN MICROBIOTA
Before moving on to discuss how, when, and where the previously mentioned agents cause human disease, we should note that the presence of microbes on or in humans is not, by itself, abnormal. In fact, from shortly after birth on, it is universal; we harbor 10 times the number of microbial cells as we do human cells. This population formerly called the normal flora is now referred to as our microbiota. These microorganisms, which are overwhelmingly bacteria, are frequently found colonizing various body sites in, healthy individuals. The constituents and numbers of the microbiota vary in different areas of the body and, sometimes, at different ages and physiologic states. They comprise microorganisms whose morphologic, physiologic, and genetic properties allow them to colonize and multiply under the conditions that exist in particular sites, to coexist with other colonizing organisms, and to inhibit competing intruders. Thus, each accessible area of the body presents a particular ecologic niche, colonization of which requires a particular set of properties of the colonizing microbe.
Organisms of the microbiota may have a symbiotic relationship that benefits the host or may simply live as commensals with a neutral relationship to the host. A parasitic relationship that injures the host would not be considered “normal,” but, in most instances, not enough is known about the organism–host interactions to make such distinctions. Like houseguests, the members of the normal flora may stay for highly variable periods. Residents are strains that have an established niche at one of the many body sites, which they occupy indefinitely. Transients are acquired from the environment and establish themselves briefly, but tend to be excluded by competition from residents or by the host’s innate or immune defense mechanisms. The term carrier state is used when potentially pathogenic organisms are involved, although its implication of risk is not always justified. For example, Streptococcus pneumoniae, a cause of pneumonia, and Neisseria meningitidis, a cause of meningitis, may be isolated from the throat of 5% to 40% of healthy people. Whether these bacteria represent transient flora, resident flora, or carrier state is largely semantic. The possibility that their presence could be the prelude to disease is impossible to determine in advance.
Flora may stay for short or extended periods
It is important for students of medical microbiology and infectious disease to understand the role of the microbiota because of its significance both as a defense mechanism against infection and as a source of potentially pathogenic organisms. In addition, it is important for physicians to know the typical composition of the microbiota at various sites to avoid confusion when interpreting laboratory culture results. The following excerpt indicates that the English poet W.H. Auden understood the need for balance between the microbiota and its host. He was influenced by an article in Scientific American about the flora of the skin.
If pathogens are involved, the relationship is called the carrier state
On this day tradition allots to taking stock of our lives, my greetings to all of you, Yeasts, Bacteria, Viruses, Aerobics and Anaerobics: A Very Happy New Year to all for whom my ectoderm is as middle earth to me.
For creatures your size I offer a free choice of habitat, so settle yourselves in the zone that suits you best, in the pools of my pores or the tropical forests of arm-pit and crotch, in the deserts of my fore-arms, or the cool woods of my scalp.
Build colonies: I will supply adequate warmth and moisture, the sebum and lipids you need, on condition you never do me annoy with your presence, but behave as good guests should, not rioting into acne or athlete’s-foot or a boil.
—W.H. Auden, Epistle to a Godson
ORIGIN AND NATURE
The healthy fetus is sterile until the birth membranes rupture. During and after birth, the infant is exposed to the flora of the mother’s vagina and to other organisms in the environment. During the infant’s first few days of life, the microbiota reflects chance exposure to organisms that can colonize particular sites in the absence of competitors. Subsequently, as the infant is exposed to a broader range of organisms, those best adapted to colonize particular sites become predominant. Thereafter, the flora generally resembles that of other individuals in the same age group and cultural milieu.
Initial flora is acquired during and immediately after birth
Local physiologic and ecologic conditions determine the microbial makeup of the flora. These conditions are sometimes highly complex, differing from site to site, and sometimes with age. Conditions include the amounts and types of nutrients available, pH, oxidation–reduction potentials, and resistance to local antibacterial substances such as bile and lysozyme. Many bacteria have adhesin-mediated affinity for receptors on specific types of epithelial cells; this facilitates colonization and multiplication and prevents removal by the flushing effects of surface fluids and peristalsis. Various microbial interactions also determine their relative prevalence in the flora. These interactions include competition for nutrients and inhibition by the metabolic products of other organisms.
Physiologic conditions such as local pH influence colonization
Adherence factors counteract mechanical flushing
Ability to compete for nutrients is an advantage
MICROBIOTA AT DIFFERENT SITES
At any one time, the microbiota of a single person contains hundreds if not thousands of species of microorganisms, mostly bacteria. The major members known to be important in preventing or causing disease, as well as those that may be confused with etiologic agents of local infections, are summarized in Table 1–3 and are described in greater detail in subsequent chapters.
TABLE 1–3 Predominant and Potentially Pathogenic Microbiota of Various Body Sites
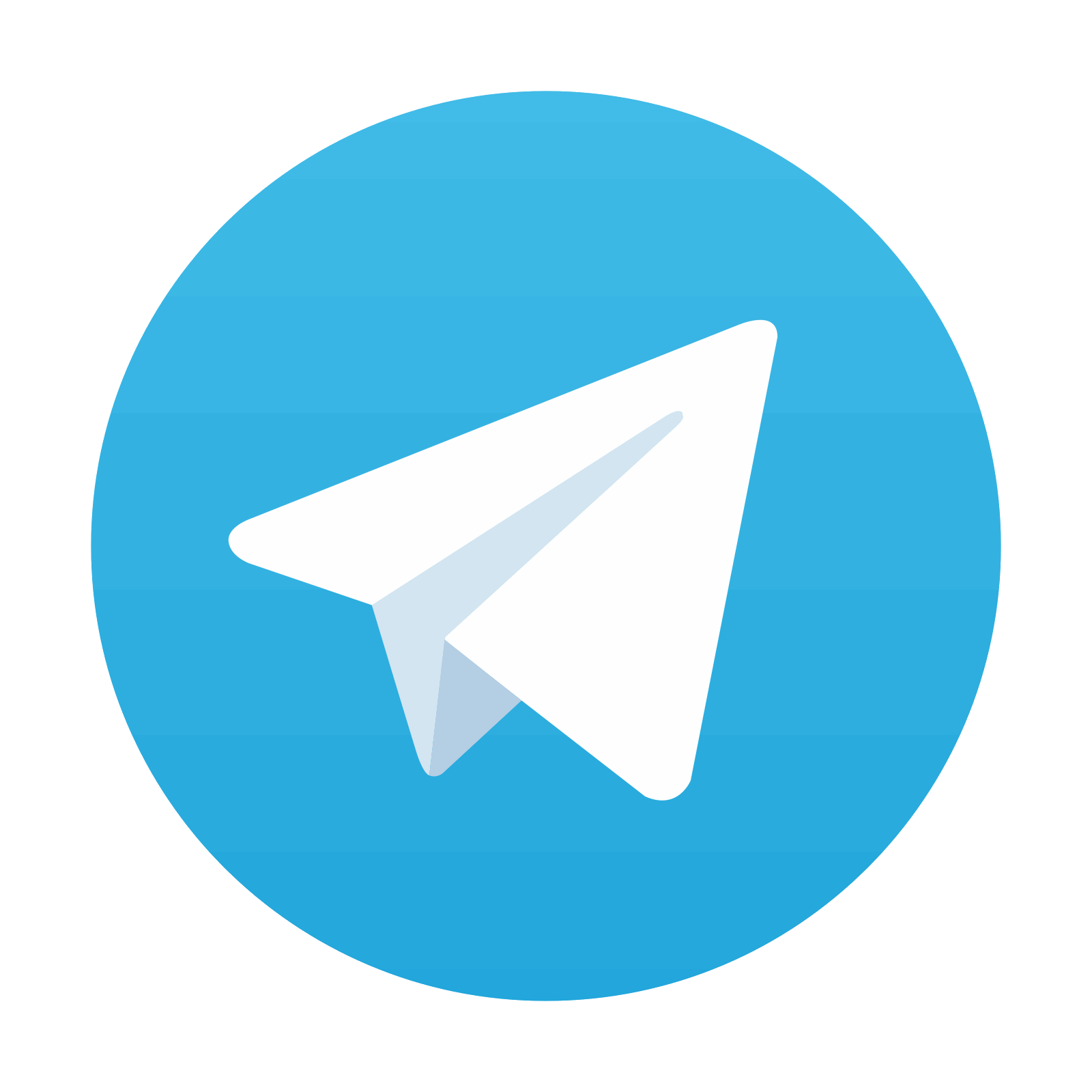
Stay updated, free articles. Join our Telegram channel

Full access? Get Clinical Tree
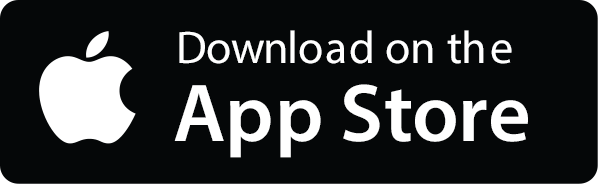
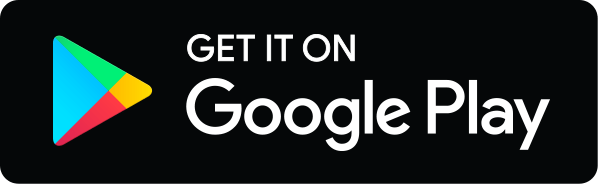