Figure 5-1. Four phases of wound healing: plotted against “Time” on the X axis, the four phases shown in different colors occur sequentially, and overlap. Overall the total time period for completion is 1 year.
Hemostasis
The first phase of hemostasis begins almost instantaneously after wounding. A scalpel drawn across intact skin injures cells in the epidermis and dermis and separates components of the extracellular matrix (ECM) that support the normal three-dimensional framework of the tissue and preserves the barrier function. The zone of injury is limited to the dimensions of the blade when using a conventional metal scalpel. With electrosurgery, the injury extends some distance into the surrounding tissues depending on the power settings on the device. As the surgeon deepens the incision, the blood vessels in the sub-dermal plexus and deeper in the subcutaneous planes, are cut, and bleeding occurs. The tissue response is mediated by factors from three sources: (1) blood leaking into the wound, (2) proteins stored in the ECM, and (3) locally resident surviving cells.
Table 5-1 Cellular and Biological Events that Frame the Normal Wound Healing Process
Circulating factors that initiate the inflammatory phase of healing are platelets, plasma proteins, and leukocytes. Platelets are unnucleated formed elements in the blood produced in the bone marrow by megakaryocytes. The plasma membrane of each platelet contains specific receptors for collagen known as the glycoprotein Ia/IIa complex. The platelet cytoplasm contains granules holding an array of factors important for hemostasis and inflammation. When plasma membrane receptors come into contact with collagen of the ECM (type I) and the basement membrane of the vascular endothelium (Type IV), platelets bind and anchor to the site. Simultaneously, platelet activation occurs and the platelet changes from a rounded amorphous shape to a flattened configuration and discharges the contents of stored cytoplasmic granules in an event known as the platelet release reaction. These bioactive factors serve a dual purpose in hemostasis and wound healing. Hemostasis is promoted by factors that cause strengthened force of platelet binding, accelerated platelet aggregation, vasoconstriction, and activation of the clotting cascade. Platelet anchoring is strengthened by von Willebrand factor (vWF) released by damaged endothelium and activated platelets. Platelet binding is also characterized by rapid vascular constriction and the formation of a stable fibrin clot. Platelets are the main cellular players of the first phase (Fig. 5-5A and Fig. 5-5B). By their collective function, they prevent hemorrhage. Platelet-derived functions that achieve this goal include: adhesion, aggregation, and formation of a procoagulant surface that facilitates the generation of thrombin and results in a fibrin plug. In addition, platelets express and release substances that promote tissue repair and influence processes such as angiogenesis, inflammation, and the immune response. They contain large secretable pools of biologically active proteins, including platelet-derived growth factor (PDGF), transforming growth factor-β (TGF-B), and vascular endothelial growth factor (VEGF) as well as cytokines while newly synthesized active metabolites including proteins such as PF4 and CD40L are also released. Although anucleate, activated platelets possess a spliceosome and can synthesize tissue factor and interleukin-1β. The binding of secreted proteins within a developing fibrin mesh or to the ECM can create chemotactic gradients favoring the recruitment of stem cells, stimulating cell migration and differentiation, and promoting repair.4 The therapeutic use of platelets in a fibrin clot has a positive influence in clinical situations requiring rapid healing. Dental implant surgery, orthopedic surgery, muscle and tendon repair, skin ulcers, hole repair in eye surgery and cardiac surgery are situations where the use of autologous platelets accelerates healing.
Figure 5-2. The dominant cells, physiologic process as well as the temporal distribution of the four phases of wound healing.
Figure 5-4. The patterns of cytokines and growth factors within each phase of wound healing.
Figure 5-5. A: Diagrammatic wound healing model. Platelets, the first cells to arrive at the wound are critical to create a clot and establish hemostasis B: Phase 1 – Hemostasis.
The growing platelet plug inside the vessel is stabilized by fibrin polymerized from circulating fibrinogen. The vascular response is mediated by local factors modulated by the systemic control of the sympathetic nervous system. Vasoconstriction, mediated by catecholamines and prostaglandins (thromboxane and PGF2a), normally is limited to the time required to achieve hemostasis. Vasodilation and alteration in capillary permeability soon follow mediated by histamine, prostaglandins (PGE2 and PGI2), and VEGF released from resident interstitial mast cells and damaged endothelium. This causes increased blood flow and controlled delivery of fluid, leukocytes, macrophages, and relevant plasma proteins to the wound environment. Platelets release two factors with particular importance for wound healing: PDGF and TGF-β. Which in turn stimulate chemotaxis and proliferation of inflammatory cells.
Inflammatory Phase
The second phase of healing involves acute inflammation that begins at the moment of tissue disruption. Inflammatory cells appearing during this phase of healing are polymorphonuclear leukocytes (PMNs) and macrophages. PMNs are first to appear (Fig. 5-6). Their primary role is to clear devitalized tissue, blood clot, foreign material, and bacteria from the wound. PMNs are part of natural host defenses that destroy bacteria by phagocytosis and secreting oxygen free radicals. Migration of PMNs from the intravascular compartment (the lumen) to the ECM and to the wound site is controlled by several biochemical agents including selectins, cytokines, and integrins that act in series to activate, tether, and facilitate extravascular escape (Fig. 5-7). PMNs work in concert with the immune system antibodies. Their numbers and persistence depend on the initial wound conditions. A clean surgical wound performed with atraumatic tissue handing will have low PMN activity, whereas a poorly performed surgical incision or a traumatic wound may be characterized by a large amount of debris and require prolonged participation of PMNs to set the stage for appearance of macrophages, the most important cellular mediator of the inflammatory phase of wound healing.
Figure 5-6. Phase 2 – Inflammation.
Macrophages appear in large numbers within 48 hours of wounding and play a central role in the inflammatory phase. They are derived from circulating monocytes or resident interstitial cells that migrate into the wound from adjacent tissues. Macrophages are multifunctional (Fig. 5-8) and complete the cleanup activities initiated by the PMNs by phagocytizing remaining wound debris. Most importantly, they secrete a vast array of cytokines and growth factors, which function in a rapidly amplifying process that affects all aspects of healing during the inflammatory phase. These factors induce recruitment and activation of additional macrophages, angiogenesis, proliferation of fibroblasts, and ECM production. Matrix production is accelerated as the inflammatory phase of healing transitions into the next phase of healing, the proliferative phase.
Proliferative Phase
The defining characteristic of the proliferative phase of healing is ECM production (Fig. 5-9). The phases of wound healing are not discreet. While the inflammatory phase is still most active, the proliferative phase begins with formation of a provisional ECM composed of fibrin and fibronectin precipitated from blood extravasated into the wound at the time of the initial injury. The provisional matrix is a protein scaffold that stabilizes the wound edges and provides a framework for migration of PMNs, macrophages, fibroblasts, and other cells into the wound from surrounding tissues. As the inflammatory phase slows the proliferative phase begins and becomes dominant. Fibroblasts replace macrophages as the most numerous cell type. Like macrophages, fibroblasts are multifunctional. They are responsible for new tissue formation, collagen production, and the laying down of the ECM (Fig. 5-9). Angiogenesis occurs simultaneously as new capillaries form and blood vessels penetrate the provisional matrix sprouting from vessels of the surrounding uninjured tissues. The processes that actually coordinate formation of ECM and new tissue with angiogenesis are not well defined. Signaling pathways that stimulate new tissue formation involve the role of low oxygen tension that increases the expression of “hypoxia-inducible factor” (HIF) by vascular endothelial cells. HIF in turn binds to specific sequences of DNA that regulate the expression of VEGF thus stimulating angiogenesis. This also has a negative feedback loop – increased formation of new blood vessels normalizes the oxygen tension. In response, oxygen binds to HIF and blocks its activity resulting in decreased synthesis of VEGF. Epidermal growth factor and TGFa produced by activated wound macrophages, platelets, and keratinocytes play an important role at the creation of a robust scaffold.
Figure 5-7. The biochemical factors that elicit migration of cells from intravascular compartment into the wound site.
Figure 5-8. Macrophage functions.
Epidermal cells migrate over the scaffold and only after the epithelial bridge is completed, enzymes are released to dissolve the attachment at the base of the overlying scab that falls off. In response to the growing need for oxygen and nutrients at the site of healing, the wound microenvironment stimulates the release of factors needed to bring in a new blood supply (low pH, reduced oxygen tension, and increased lactate). This process – angiogenesis or neovascularization is stimulated by VEGF, basic fibroblast growth factor (bFGF), and TGFb. These factors are secreted by several cell types including vascular endothelial cells, epidermal cells, fibroblasts, and macrophages.
As the proliferative phase progresses the predominant cell in the wound site is the fibroblast. This multifunctional cell of mesenchymal origin mainly produces and deposits the new matrix for structural integrity at the level of the wound bed (Fig. 5-9B). ECM production is the defining feature of the proliferative phase. The ECM is primarily collagen. At least 23 individual types of collagen have been identified – type I is present mostly in scar tissues.5 Fibroblasts produce collagen via their attachment to the cables of the provisional fibrin matrix.6 After transcription and processing of the collagen messenger ribonucleic acid, it is attached to polyribosomes on the endoplasmic reticulum where the new collagen chains are produced. During this process, there is an important step involving hydroxylation of proline and lysine residues.7 The collagen molecule transforms itself into the classical triple helical structure and thereafter its nascent chains are modified through glycosylation8; this procollagen molecule is released into the extracellular space.9 The hydroxyproline in collagen gives the molecule its stable helical conformation.10 Whereas fully hydroxylated collagen has a higher stability, unhydroxylated forms are fragile, similar to collagen produced under anaerobic disease conditions or vitamin C-deficient states (scurvy), wherein the collagen undergoes denaturation easily and can break.
Figure 5-9. A: Phase 3 – Proliferation. Vast array of cells are recruited into the wound bed and carry out diverse functions including proliferation and deposition of ECM. B: Fibroblast functions.
Finally, collagen released into the extracellular space undergoes further processing by cleavage of the procollagen N- and C-terminal peptides. In the extracellular spaces, an important enzyme, lysyl oxidase, acts on the collagen to form stable cross-links. As the collagen matures and becomes older, more and more of these intramolecular and intermolecular cross-links are placed in the molecules. This important cross-linking step gives collagen its strength and stability over time.11
Remodeling Phase
The remodeling phase is characterized by continued synthesis and degradation of the ECM components trying to establish a new equilibrium – and the formation of an organized scar (Fig. 5-10). Collagen degradation occurs12 via the action of specific collagenases that are secreted by various cells: fibroblasts, neutrophils, and macrophages each of which can cleave the collagen molecule at differing but specific locations on all three chains, and break it down to characteristic three-quarter and one-quarter pieces. These collagen fragments undergo further denaturation and digestion by other proteases. Several molecules including TGF play a major role in the remodeling phase. TGF-β-induced intracellular signaling acts via a set of proteins called the SMAD proteins, which act as direct links between the cell surface and the nucleus. The recent development of several SMAD pathway specific knockout mice and transgenic animals has confirmed the pivotal nature of the SMAD pathway in fibrogenesis and tumorigenesis. Still, several difficulties remain before the TGF-β/SMAD pathway can be efficiently targeted in situations such as tissue fibrosis or impaired wound healing. In particular, the precise spatiotemporal role of each TGF-β/SMAD pathway component during the development of excessive ECM deposition leading to tissue fibrosis remains to be ascertained.
As the scar matures, late remodeling occurs (that takes up to 1 year); the scar contracts and thins out (Fig. 5-11).
CLINICAL APPLICATIONS
Surgical Technique
The surgeon equipped with the knowledge of the fundamentals of wound healing is prepared to insightfully minimize risks of wound healing complications while performing a surgical procedure from start to finish. The stage is set for healing from the moment the incision is made. The skin and dermis should be incised perpendicularly to the plane of the surface. Attention to this principle is particularly important when making an incision on a curved surface. Electrosurgical currents should be used set on the lowest power settings that accomplish hemostasis. The deep tissues should be handled as atraumatically as possible. The incision is carried down through deeper layers ensuring that each new incision is accurately placed in the same line as the previous one. This avoids a saw tooth surface with devitalized sections. Proper tissue handling techniques in the subcutaneous fat and adjacent soft tissue are based on well-established wound healing principles, which minimize the risk of infection, seroma, delayed healing, unnecessary scarring, and other postoperative wound complications. For traumatic wounds, the first step in treatment is to convert them into controlled surgical wounds by thoughtful debridement and tissue repair. Treating traumatic wounds minimizing the risk of postoperative wound complications requires mindfulness of wound healing principles in all of these clinical circumstances. This is true regardless whether performing repair of a simple laceration or the most complex specialized procedure. Controlling the degree of injury leads to improved outcomes with fewer complications related to a failure in the wound healing process. An awareness of the wound healing process informs proper surgical technique. A clear understanding of wound healing allows the surgeon to advance in technical skill and achieve continuously improving outcomes throughout a professional career.
Figure 5-11. Contraction of scar – This process occurs over the course of 1 year.
Figure 5-12. Effects of biofilm on wound healing.
Elective surgery creates controlled tissue injury. Minimizing tissue injury forms the basis of proper surgical technique. Trauma inures tissues in an uncontrolled fashion. Wounds can occur under special conditions such as pressure, physiologic impairment (e.g., diabetes) that create traumatic tissue damage in an uncontrolled injury. Finally, there are wounds that occur under specialized circumstances such as radiotherapy in cancer treatment.
Biofilm
Biofilm comprises a colony of microorganisms enveloped with a matrix of extracellular polymers. Estimated biofilm-associated infections costs >$1 billion annually. Both chronic and acute dermal wounds are susceptible to the formation and propagation of biofilm. Covered in other chapters of this book, biofilm is relevant to wound healing due to the several inhibitory effects on healing processes (Fig. 5-12).
Table 5-2 Comparison of Acute and Chronic Wounds
Chronic Versus Acute wounds
Wounds heal within a reasonable time of 4 to 6 week. Those that do not are termed chronic. As shown in Table 5-2, a variety of factors and disease conditions impair wound healing and result in chronic nonhealing wounds. Reasons that lead to chronicity instead of normal healing, are complex – but the salient points are defined in Figure 5-13. The most important concept is a persistent proinflammatory condition that paradoxically leads to increased degradation of matrix proteins, and an unstable wound that is recalcitrant to healing.
Comorbid Conditions that Influence Wound Healing
3 Wound healing is a complex biological process that consists of hemostasis, inflammation, proliferation, and remodeling. Large numbers of cell types – including neutrophils, macrophages, lymphocytes, keratinocytes, fibroblasts, and endothelial cells – are involved in this process. Multiple factors can cause impaired wound healing by affecting one or more phases of the process and are categorized into local and systemic factors (Table 5-3). The influences of these factors are not mutually exclusive.13 Single or multiple factors may play a role in any one or more individual phases, contributing to the overall outcome of the healing process (Fig. 5-14).
Advanced Age and Gender
The elderly population (people over 60 years of age) is growing faster than any other age group (World Health Organization [WHO, www.who.int/topics/ageing]), and increased age is a major risk factor for impaired wound healing. Many clinical and animal studies at the cellular and molecular level have examined age-related changes and delays in wound healing. It is commonly recognized that, in healthy older adults, the effect of aging causes a temporal delay in wound healing, but not an actual impairment in terms of the quality of healing.13 Delayed wound healing in the aged is associated with an altered inflammatory response, such as delayed T-cell infiltration into the wound area with alterations in chemokine production and reduced macrophage phagocytic capacity.14 Overall, there are global differences in wound healing between young and aged individuals. A review of the age-related changes in healing capacity demonstrates that every phase of healing undergoes characteristic age-related changes, including enhanced platelet aggregation, increased secretion of inflammatory mediators, delayed infiltration of macrophages and lymphocytes, impaired macrophage function, decreased secretion of growth factors, delayed re-epithelialization, delayed angiogenesis and collagen deposition, reduced collagen turnover and remodeling, and decreased wound strength.
Table 5-3 Factors that Affect Wound Healing
Figure 5-13. Comparison of cellular mechanisms in normal and poor wound healing.
Sex hormones play a role in age-related wound healing deficits. Compared with aged females, aged males have been shown to have delayed healing of acute wounds. A partial explanation for this is that the female estrogens (estrone and 17β-estradiol), male androgens (testosterone and 5α-dihydrotestosterone, DHT), and their steroid precursor dehydroepiandrosterone (DHEA) appear to have significant effects on the wound healing process.15 It was recently found that the differences in gene expression between elderly male and young human wounds are almost exclusively estrogen regulated.16 Estrogen affects wound healing by regulating a variety of genes associated with regeneration, matrix production, protease inhibition, epidermal function, and genes primarily associated with inflammation.17 Studies indicate that estrogen can improve the age-related impairment in healing in both men and women, while androgens regulate cutaneous wound healing negatively.
Figure 5-14. Mechanisms that elicit the effects of comorbid conditions on wound healing.
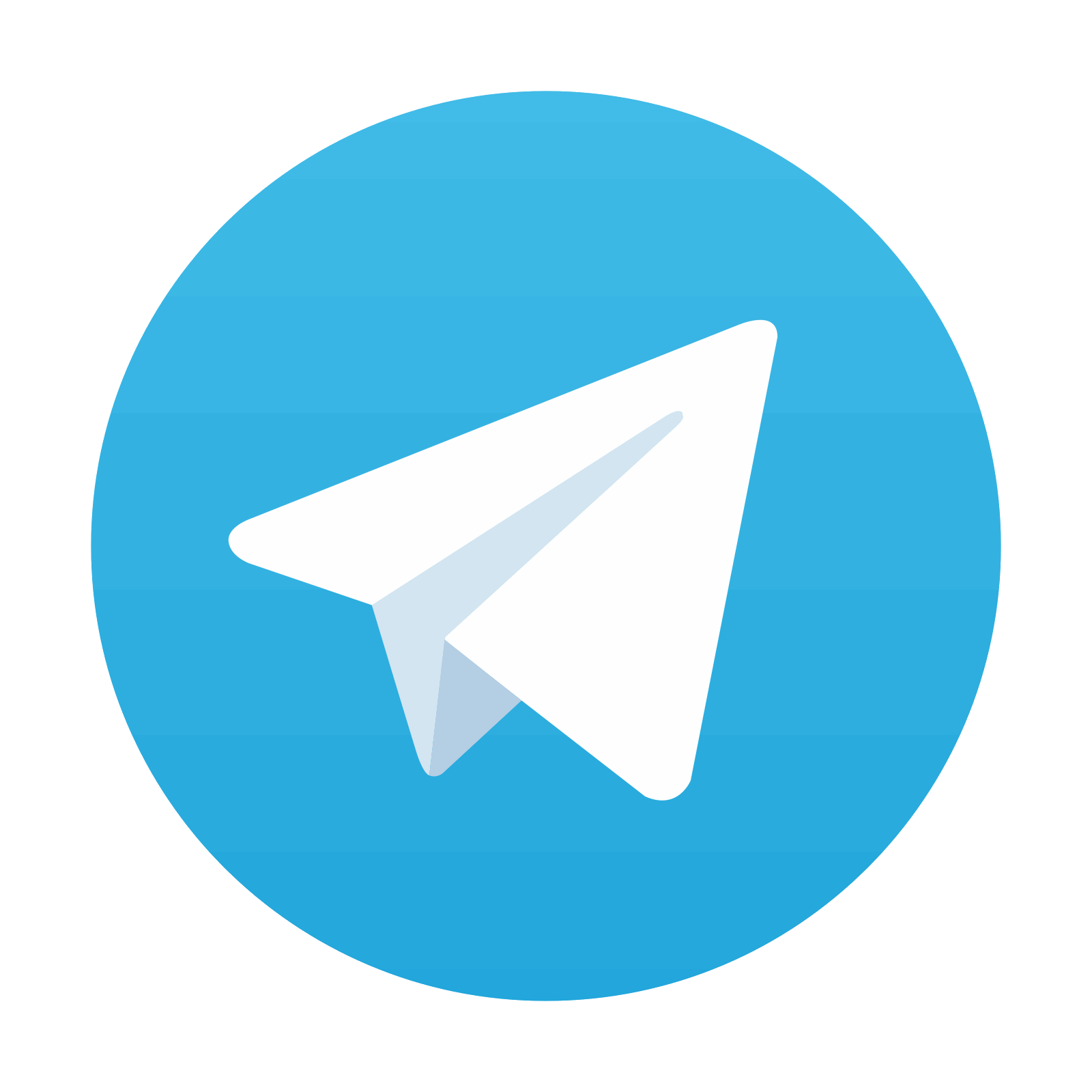
Stay updated, free articles. Join our Telegram channel

Full access? Get Clinical Tree
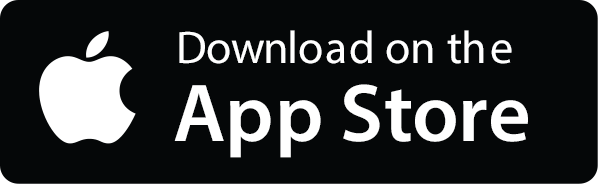
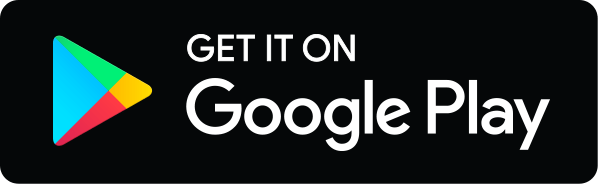