Approximately 60% of our body is water. It acts as a solvent for the substances we need, such as K+, glucose, adenosine triphosphate (ATP), and proteins. It is important for the transport of molecules and heat. Many of the compounds produced in the body and dissolved in water contain chemical groups that act as acids or bases, releasing or accepting hydrogen ions. The hydrogen ion content and the amount of body water are controlled to maintain a constant environment for the cells called homeostasis (same state) (Fig. 4.1). Significant deviations from a constant environment, such as acidosis or dehydration, may be life-threatening. This chapter describes the role of water in the body and the buffer systems used by the body to protect itself from acids and bases produced from metabolism.
FIGURE 4.1 Maintenance of body pH. The body produces approximately 13 to 22 mol/day of acid from normal metabolism. The body protects itself against this acidity by buffers that maintain a neutral pH and by the expiration of CO2 through the lungs and by the excretion of NH4+ and other ions through the kidneys.
Water. Water is distributed between intracellular and extracellular compartments, the latter comprising interstitial fluids, blood, and lymph. Because water is a dipolar molecule with an uneven distribution of electrons between the hydrogen and oxygen atoms, it forms hydrogen bonds with other polar molecules and acts as a solvent.
The pH of Water. Water dissociates to a slight extent to form hydrogen (H+) and hydroxyl (OH−) ions. The concentration of hydrogen ions determines the acidity of the solution, which is expressed in terms of pH. The pH of a solution is the negative log of its hydrogen ion concentration.
Acids and Bases. An acid is a substance that can release hydrogen ions (protons), and a base is a substance that can accept hydrogen ions. When dissolved in water, almost all the molecules of a strong acid dissociate and release their hydrogen ions, but only a small percentage of the total molecules of a weak acid dissociate. A weak acid has a characteristic acid dissociation constant, Ka. The relationship between the pH of a solution, the Ka of an acid, and the extent of its dissociation are given by the Henderson–Hasselbalch equation.
Buffers. A buffer is a mixture of an undissociated acid and its conjugate base (the form of the acid that has lost its proton). It causes a solution to resist changes in pH when either H+ or OH− is added. A buffer has its greatest buffering capacity in the pH range near its pKa (the negative log of its Ka). Two factors determine the effectiveness of a buffer: its pKa relative to the pH of the solution and its concentration.
Metabolic Acids and Bases. Normal metabolism generates CO2, metabolic acids (e.g., lactic acid and ketone bodies), and inorganic acids (e.g., sulfuric acid). The major source of acid is CO2, which reacts with water to produce carbonic acid. To maintain the pH of body fluids in a range compatible with life, the body has buffers such as bicarbonate, phosphate, and hemoglobin (see Fig. 4.1). Ultimately, respiratory mechanisms remove carbonic acid through the expiration of CO2, and the kidneys excrete acid as ammonium ion (NH4+) and other ions.
THE WAITING ROOM 
Dianne (Di) A. is a 26-year-old woman who was diagnosed with type 1 diabetes mellitus at the age of 12 years. She has an absolute insulin deficiency resulting from autoimmune destruction of the β-cells of her pancreas. As a result, she depends on daily injections of insulin to prevent severe elevations of glucose and ketone bodies in her blood. When she could not be aroused from an afternoon nap, her roommate called an ambulance, and Dianne A. was brought to the emergency department of the hospital in a coma. Her roommate reported that Dianne A. had been feeling nauseated and drowsy and had been vomiting for 24 hours. Dianne A. is clinically dehydrated, and her blood pressure is low. Her respirations are deep and rapid, and her pulse rate is rapid. Her breath has the “fruity” odor of acetone.
Blood samples are drawn for measurement of her arterial blood pH, arterial partial pressure of carbon dioxide (PaCO2), serum glucose, and serum bicarbonate (HCO3−). In addition, serum and urine are tested for the presence of ketone bodies, and Dianne A. is treated with intravenous normal saline and insulin. The laboratory reports that her blood pH is 7.08 (reference range 7.36 to 7.44) and that ketone bodies are present in both blood and urine. Her blood glucose level is 648 mg/dL (reference range 70 to 100 mg/dL after an overnight fast, and no higher than 200 mg/dL in a casual glucose sample taken without regard to the time of the last meal).
Dennis V., age 3 years, was brought to the emergency department by his grandfather, Percy V. While Dennis V. was visiting his grandfather, he climbed up on a chair and took a half-full 500-tablet bottle of 325-mg aspirin (acetylsalicylic acid) tablets from the kitchen counter. Percy V. discovered Dennis V. with a mouthful of aspirin, which he removed, but he could not tell how many tablets Dennis V. had already swallowed. When they arrived at the emergency department, the child appeared bright and alert, but Dennis V. was hyperventilating.
I. Water
Water is the solvent of life. It bathes our cells, dissolves and transports compounds in the blood, provides a medium for movement of molecules into and throughout cellular compartments, separates charged molecules, dissipates heat, and participates in chemical reactions. Most compounds in the body, including proteins, must interact with an aqueous medium in order to function. In spite of the variation in the amount of water we ingest each day and produce from metabolism, our body maintains a nearly constant amount of water that is approximately 60% of our body weight (Fig. 4.2).
FIGURE 4.2 Fluid compartments in the body based on an average 70-kg man.
A. Fluid Compartments in the Body
Total body water is roughly 50% to 60% of body weight in adults and 75% of body weight in children. Because fat has relatively little water associated with it, obese people tend to have a lower percentage of body water than thin people, women tend to have a lower percentage than men, and older people have a lower percentage than younger people.
Approximately 60% of the total body water is intracellular and 40% extracellular (see Fig. 4.2). The extracellular water includes the fluid in plasma (blood after the cells have been removed) and interstitial water (the fluid in the tissue spaces, lying between cells). Transcellular water is a small, specialized portion of extracellular water that includes gastrointestinal secretions, urine, sweat, and fluid that has leaked through capillary walls because of such processes as increased hydrostatic pressure or inflammation.
B. Hydrogen Bonds in Water
The dipolar nature of the water (H2O) molecule allows it to form hydrogen bonds, a property that is responsible for the role of water as a solvent. In H2O, the oxygen atom has two unshared electrons that form an electron-dense cloud around it. This cloud lies above and below the plane formed by the water molecule (Fig. 4.3). In the covalent bond formed between the hydrogen and oxygen atoms, the shared electrons are attracted toward the oxygen atom, thus giving the oxygen atom a partial negative charge and the hydrogen atom a partial positive charge. As a result, the oxygen side of the molecule is much more electronegative than the hydrogen side, and the molecule is dipolar.
FIGURE 4.3 Hydrogen bonds between water molecules. The oxygen atoms are shown in black.
Both the hydrogen and oxygen atoms of the water molecule form hydrogen bonds and participate in hydration shells. A hydrogen bond is a weak noncovalent interaction between the hydrogen of one molecule and the more electronegative atom of an acceptor molecule. The oxygen of water can form hydrogen bonds with two other water molecules so that each water molecule is hydrogen-bonded to approximately four close neighboring water molecules in a fluid three-dimensional lattice (see Fig. 4.3).
1. Water as a Solvent
Polar organic molecules and inorganic salts can readily dissolve in water because water also forms hydrogen bonds and electrostatic interactions with these molecules. Organic molecules containing a high proportion of electronegative atoms (generally oxygen or nitrogen) are soluble in water because these atoms participate in hydrogen bonding with water molecules (Fig. 4.4A). Chloride (Cl−), bicarbonate (HCO3−), and other anions are surrounded by a hydration shell of water molecules arranged with their hydrogen atoms closest to the anion. Similarly, the oxygen atom of water molecules interacts with inorganic cations such as Na+ and K+ to surround them with a hydration shell (see Fig. 4.4B).
FIGURE 4.4 A. Hydrogen bonds between water and polar molecules. R denotes additional atoms. B. Hydration shells surrounding anions and cations.
Although hydrogen bonds are strong enough to dissolve polar molecules in water and to separate charges, they are weak enough to allow the movement of water and solutes. The strength of the hydrogen bond between two water molecules is only approximately 4 kcal, roughly 1/20th of the strength of the covalent O–H bond in the water molecule. Thus, the extensive water lattice is dynamic and has many strained bonds that are continuously breaking and re-forming. The average hydrogen bond between water molecules lasts only about 10 picoseconds (1 picosecond is 10−12 seconds), and each water molecule in the hydration shell of an ion stays only 2.4 nanoseconds (1 nanosecond = 10−9 seconds). As a result, hydrogen bonds between water molecules and polar solutes continuously dissociate and re-form, thereby permitting solutes to move through water and water to pass through channels in cellular membranes.
2. Water and Thermal Regulation
The structure of water also allows it to resist temperature change. Its heat of fusion is high, so a large drop in temperature is needed to convert liquid water to the solid state of ice. The thermal conductivity of water is also high, thereby facilitating heat dissipation from high energy utilizing areas such as the brain into the blood and the total body water pool. Its heat capacity and heat of vaporization are remarkably high; as liquid water is converted to a gas and evaporates from the skin, we feel a cooling effect. Water responds to the input of heat by decreasing the extent of hydrogen bonding and to cooling by increasing the bonding between water molecules.
C. Electrolytes
Both extracellular fluid (ECF) and intracellular fluid (ICF) contain electrolytes, a general term applied to bicarbonate and inorganic anions and cations. The electrolytes are unevenly distributed between compartments; Na+ and Cl− are the major electrolytes in the ECF (plasma and interstitial fluid), and K+ and phosphates such as HPO42− are the major electrolytes in cells (Table 4.1). This distribution is maintained principally by energy-requiring transporters that pump Na+ out of cells in exchange for K+ (see Chapter 10).
TABLE 4.1 Distribution of Ions in Body Fluids
ECFa (MMOL/L) | ICF (MMOL/L) | |
Cations | ||
Na+ | 145 | 12 |
K+ | 4 | 150 |
Anions | ||
Cl− | 105 | 5 |
HCO3− | 25 | 12 |
Inorganic phosphate | 2 | 100 |
ECF, extracellular fluid; ICF, intracellular fluid.
aThe content of inorganic ions is very similar in plasma and interstitial fluid, the two components of the extracellular fluid.
D. Osmolality and Water Movement
Water distributes between the different fluid compartments according to the concentration of solutes, or osmolality, of each compartment. The osmolality of a fluid is proportional to the total concentration of all dissolved molecules, including ions, organic metabolites, and proteins, and is usually expressed as milliosmoles (mOsm)/kg water. The semipermeable cellular membrane that separates the extracellular and intracellular compartments contains a number of ion channels through which water can move freely but other molecules cannot. Likewise, water can move freely through the capillaries separating the interstitial fluid and the plasma. As a result, water will move from a compartment with a low concentration of solutes (lower osmolality) to one with a higher concentration to achieve an equal osmolality on both sides of the membrane. The force it would take to keep the same amount of water on both sides of the membrane is called the osmotic pressure.
As water is lost from one fluid compartment, it is replaced with water from another compartment to maintain a nearly constant osmolality. The blood contains a high content of dissolved negatively charged proteins and the electrolytes needed to balance these charges. As water is passed from the blood into the urine to balance the excretion of ions, the blood volume is repleted with water from interstitial fluid. When the osmolality of the blood and interstitial fluid is too high, water moves out of the cells. The loss of cellular water also can occur in hyperglycemia because the high concentration of glucose increases the osmolality of the blood.
II. Acids and Bases
Acids are compounds that donate a hydrogen ion (H+) to a solution, and bases are compounds (such as the OH− ion) that accept hydrogen ions. Water itself dissociates to a slight extent, generating hydrogen ions (H+), which are also called protons, and hydroxide ions (OH−) (Fig. 4.5). The hydrogen ions are extensively hydrated in water to form species such as H3O+, but nevertheless are usually represented as simply H+. Water itself is neutral, neither acidic nor basic.
FIGURE 4.5 Dissociation of water.
A. The pH of Water
The extent of dissociation by water molecules into H+ and OH− is very slight, and the hydrogen ion concentration of pure water is only 0.0000001 M or 10−7 mol/L. The concentration of hydrogen ions in a solution is usually denoted by the term pH, which is the negative log10 of the hydrogen ion concentration expressed in moles per liter (mol/L) (Equation 4.1). Therefore, the pH of pure water is 7.
Equation 4.1. Definition of pH
pH = −log[H+]
The dissociation constant for water, Kd, expresses the relationship between the hydrogen ion concentration [H+], the hydroxide ion concentration [OH−], and the concentration of water [H2O] at equilibrium (Equation 4.2). Because water dissociates to such a small extent, [H2O] is essentially constant at 55.5 M. Multiplication of the Kd for water (approximately 1.8 × 10−16 M) by 55.5 M gives a value of approximately 10−14 (mol/L)2, which is called the ion product of water (Kw) (Equation 4.3). Because Kw, the product of [H+] and [OH−], is always constant, a decrease of [H+] must be accompanied by a proportionate increase of [OH−].
Equation 4.2. Dissociation constant of water
Equation 4.3. Ion product of water
Kw = [H+] [OH−] = 1 × 10−14
A pH of 7 is termed neutral because [H+] and [OH−] are equal. Acidic solutions have a greater hydrogen ion concentration and a lower hydroxide ion concentration than pure water (pH < 7.0), and basic solutions have a lower hydrogen ion concentration and a greater hydroxide ion concentration (pH > 7.0).
B. Strong and Weak Acids
During metabolism, the body produces a number of acids that increase the hydrogen ion concentration of the blood or other body fluids and tend to lower the pH (Table 4.2). These metabolically important acids can be classified as weak acids or strong acids by their degree of dissociation into a hydrogen ion and a base (the anion component). Inorganic acids such as sulfuric acid (H2SO4) and hydrochloric acid (HCl) are strong acids that dissociate completely in solution (Fig. 4.6). Organic acids containing carboxylic acid groups (e.g., the ketone bodies acetoacetic acid and β-hydroxybutyric acid) are weak acids that dissociate to only a limited extent in water. In general, a weak acid (HA), called the conjugate acid, dissociates into a hydrogen ion and an anionic component (A−), called the conjugate base. The name of an undissociated acid usually ends in “ic acid” (e.g., acetoacetic acid), and the name of the dissociated anionic component ends in “ate” (e.g., acetoacetate).
TABLE 4.2 Acids in the Blood of a Healthy Individual
ACID | ANION | pKa | MAJOR SOURCES |
Strong acid | |||
Sulfuric acid (H2SO4) | Sulfate (SO42−) | Completely dissociated | Dietary sulfate and S-containing amino acids |
Weak acid | |||
Carbonic acid (R–COOH) | Bicarbonate (R–COO−) | 3.80 | CO2 from TCA cycle |
Lactic acid (R–COOH) | Lactate (R–COO−) | 3.73 | Anaerobic glycolysis |
Pyruvic acid (R–COOH) | Pyruvate (R–COO−) | 2.39 | Glycolysis |
Citric acid (R–3COOH) | Citrate (R–3COO−) | 3.13; 4.76; 6.40 | TCA cycle and diet (e.g., citrus fruits) |
Acetoacetic acid (R–COOH) | Acetoacetate (R–COO–) | 3.62 | Fatty acid oxidation to ketone bodies |
β-Hydroxybutyric acid (R–COOH) | β-Hydroxybutyrate (R–COO−) | 4.41 | Fatty acid oxidation to ketone bodies |
Acetic acid (R–COOH) | Acetate (R–COO−) | 4.76 | Ethanol metabolism |
Dihydrogen phosphate (H2PO4–) | Monohydrogen phosphate (HPO42−) | 7.2 | Dietary organic phosphates |
Ammonium ion (NH4+) | Ammonia (NH3) | 9.25 | Dietary nitrogen-containing compounds |
pKa, the pH at which 50% dissociation occurs; TCA, tricarboxylic acid.
FIGURE 4.6 Dissociation of acids. Sulfuric acid is a strong acid that dissociates into H+ ions and sulfate. The ketone bodies acetoacetic acid and β-hydroxybutyric acid are weak acids that partially dissociate into H+ and their conjugate bases.
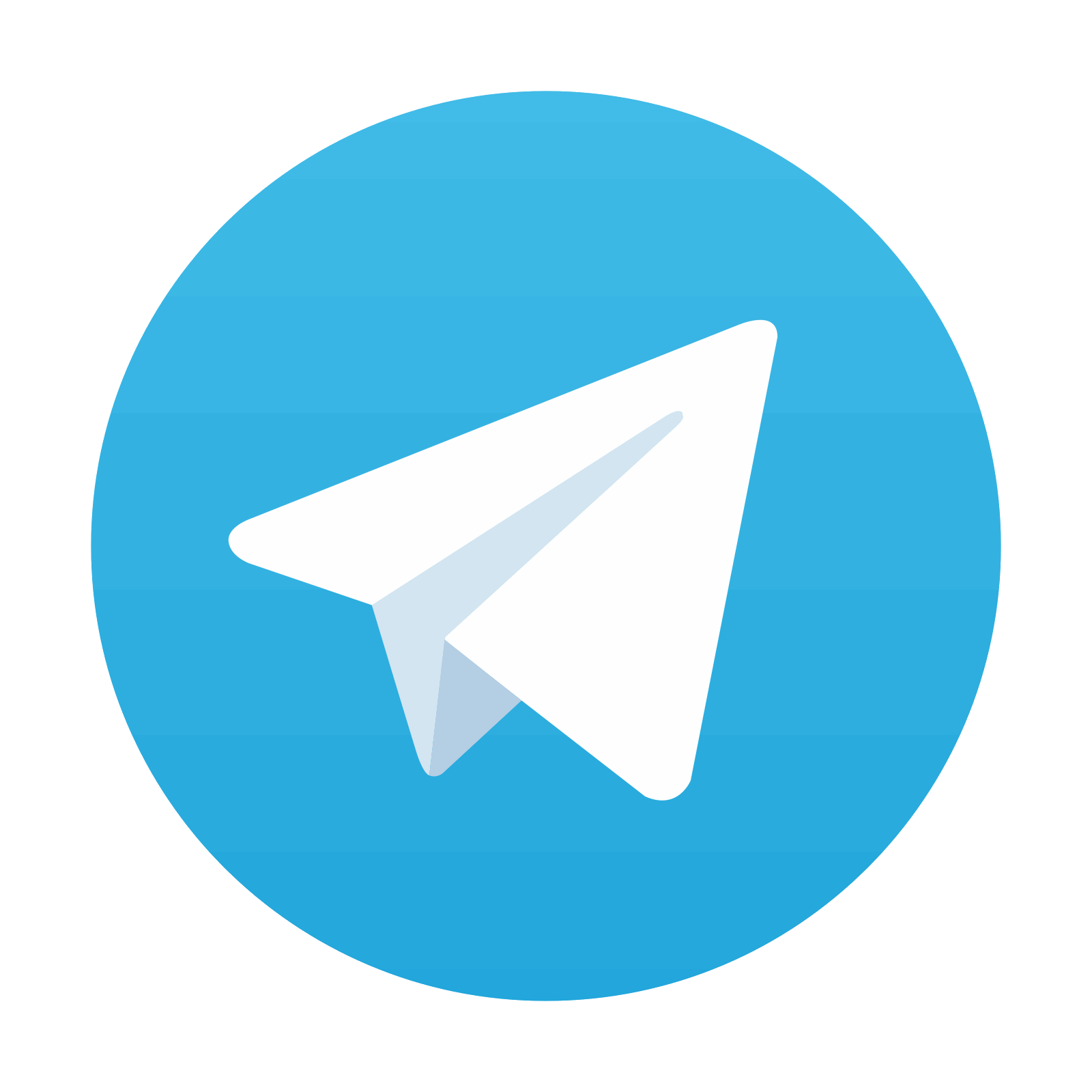
Stay updated, free articles. Join our Telegram channel

Full access? Get Clinical Tree
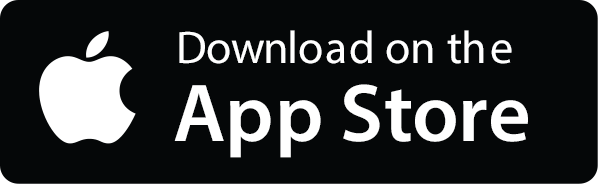
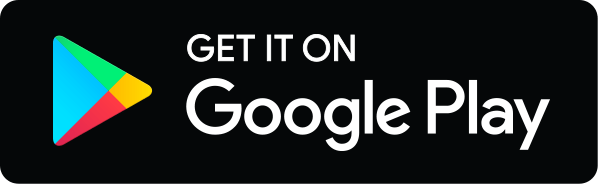