23.1 INTRODUCTION TO VIRUSES AND CANCER
A cancer in a human or an animal is a malignant tumor and involves continuous proliferation of a clone of cells derived from one of the body’s normal cells. The cell undergoes changes known as transformation as a result of events that include mutation, activation of oncogenes (tumor genes), and inactivation of tumor suppressors. The types of cancer that will be discussed in this chapter, and the cell types from which they are derived, are listed in Table 23.1.
Table 23.1 Types of cancer and the cell types from which they are derived
Cancer | Cell type |
Carcinoma | Epithelium |
Leukemia | Blood-forming |
Lymphoma | Lymphocyte |
Mesothelioma | Mesothelium |
Sarcoma | Connective tissue |
For most cancers the full sequence of events that turns a normal cell into a tumor cell (the process of oncogenesis) is not fully understood, but it is thought that between four and six steps are involved. Some of the steps in this transformation can be triggered by environmental factors including some chemicals, some forms of irradiation, and some viruses.
A virus that is able to cause cancer is known as an oncogenic virus. Evidence that a virus is oncogenic includes the regular presence in the tumor cells of virus DNA, which might be all or a part of the virus genome. In some types of tumor the virus DNA is integrated into a cell chromosome, while in other types it is present as multiple copies of covalently closed circular DNA (cccDNA), as discussed in the context of latent virus infections (see Figure 9.7). In many cases one or more of the virus genes are expressed in the tumor cell and virus proteins can be detected.
For some types of cancer there is evidence for involvement of a virus in most, if not all, cases of the cancer. For other types virus DNA and/or proteins are detectable in only a minority of cases and it is possible that the virus is just one of a number of carcinogenic factors that can give rise to these cancers. Some human adenoviruses are oncogenic in that they can transform cells in culture and can cause tumors when inoculated into animals, though there is no evidence that adenoviruses cause cancer in humans. Studies with these viruses have, however, contributed to understanding of oncogenic mechanisms.
This chapter is mainly concerned with viruses that cause human cancers, of which about 20% of cases in females and about 8% in males are thought to be caused by viruses. The relative incidences of the main virus-associated cancers are shown in Figure 23.1. The viruses involved are found worldwide and some are very common, though the prevalence of some varies between regions. We shall also briefly consider some animal cancers caused by viruses.
Figure 23.1 Virus-associated cancers in humans.
Source: Modified from Microbiology Today, August 2005, with the permission of Professor D. H. Crawford (University of Edinburgh) and the Society for General Microbiology.
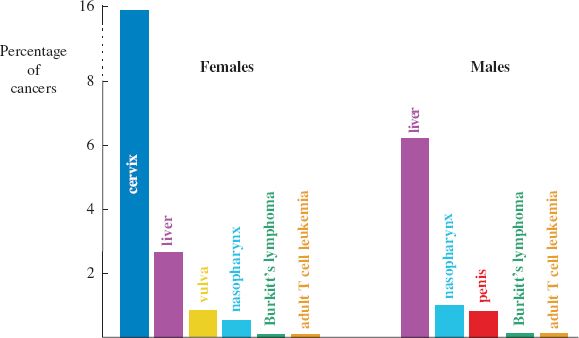
23.2 PAPILLOMAVIRUS-LINKED CANCERS
Cervical carcinoma is the third most common cancer in women, constituting almost 16% of cancers in females (Figure 23.1). In the world each year there are approximately half a million new cases of cervical cancer and 275 000 deaths caused by this cancer. The vast majority of these cases result from infection with a papillomavirus.
The papillomaviruses are small DNA viruses of mammals and birds (Figures 3.16 and 23.2). They enter the body through small abrasions in skin and mucous membranes, and infect basal cells in the epithelium. Sequencing the genomes of human papillomaviruses (HPVs) has differentiated about 200 types. Each HPV type infects a preferred site, such as the hands or the genitals, and infection may result in a benign wart (papilloma) or a carcinoma.
Figure 23.2 Virion characteristics of the families Papillomaviridae and Polyomaviridae. The virions of both families consist of icosahedral capsids containing dsDNA; papillomavirus virions are larger (50–55 nm diameter) than those of polyomaviruses (40–45 nm diameter).
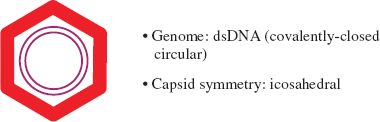
The papillomaviruses that infect the genitals are transmitted between individuals during sexual contact. Most papillomavirus infections do not become persistent, but in a minority of hosts the infection is not cleared by the host’s immune response. In individuals who harbor a persistent infection there is a risk of cancer developing. This risk is associated with about 15 of the HPV types; these “high-risk” types include HPV-16 and 18 (Figure 23.3). Infection with other HPV types that infect the genitals carries little or no risk of cancer; these “low-risk” HPV types include HPV-6 and 11, and are associated almost exclusively with benign genital warts.
Figure 23.3 Phylogenetic tree showing relationships between some HPVs. The high-risk HPVs cluster in two regions of the tree.
Source: Modified from Microbiology Today, August 2005, with the permission of Professor N. J. Maitland (University of York) and the Society for General Microbiology. Data from Los Alamos National Laboratory HPV website (http://hpv-web.lanl.gov).
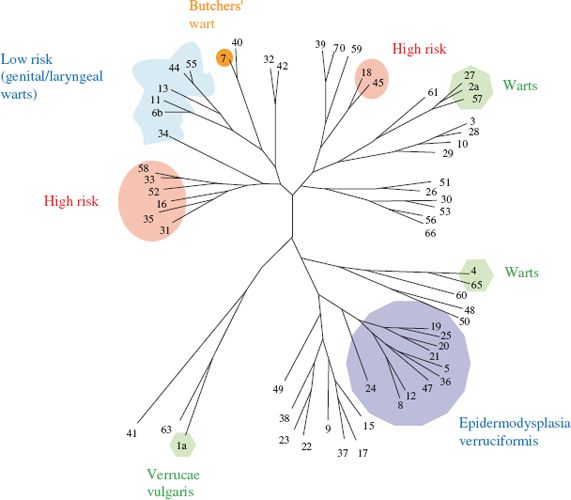
The stages from normal cervical cell to cancer cell involve a number of morphological changes that can be detected by microscopic observation of a sample of cells taken from the cervix. If precancer cells are detected they can be killed or removed to prevent the development of a tumor. This is one approach to cervical cancer screening; an alternative approach involves testing the cells for the DNA of a high-risk HPV. All or part of the genome of one of these viruses can nearly always be found (usually integrated into a chromosome (Figure 9.7)) in precancer cells and in cancer cells. The virus replication cycle is not completed in these cells so no progeny virions are formed.
There is evidence that high-risk HPVs (especially HPV-16) are also causative agents of some carcinomas at other body sites, including the vulva, vagina, penis, anus, mouth, head, and neck. Again the evidence is the presence in the tumor cells of HPV DNA.
HPVs are also involved in a very rare form of skin cancer, which has a genetic basis: epidermodysplasia verruciformis. In this disease the patient is highly susceptible to infection with HPVs, especially a number of types that are rare in the normal population, mainly types 5 and 8 (Figure 23.3). Warts spread over the entire body during childhood and 25–33% of patients develop cancer (squamous cell carcinoma) in areas of the skin exposed to ultraviolet light (sunlight). HPV DNA can be detected in more than 80% of these cancers but, in contrast to cervical cancers, the DNA is rarely integrated into a cell chromosome.
23.3 POLYOMAVIRUS-LINKED CANCERS
Polyomavirus virions have the same characteristics as those of the papillomaviruses, except that they are slightly smaller (Figure 23.2). They are found in mammals and birds, and most infections are subclinical. Injection of a polyomavirus into certain animal species, however, can induce the development of many types of tumor (“poly-oma”).
Several human polyomaviruses have been discovered and have been found to be extremely common. There is evidence that at least one of them has a role in the development of a cancer: Merkel cell carcinoma, a rare skin cancer that mainly affects elderly and immunosuppressed people. The tumor cells contain the integrated genome of Merkel cell polyoma virus and express the T (= tumor) antigen of this virus.
A monkey polyomavirus, simian virus 40 (SV40), was first isolated from primary cell cultures derived from rhesus monkey kidneys. The cell cultures were being used to produce poliovirus for Salk vaccine. The quality control procedures included injection of vaccine into hamsters, and some of these animals developed tumors. It was subsequently found that injection of SV40 into a newborn hamster could cause a carcinoma, sarcoma, lymphoma, or leukemia, depending on the injection site. Millions of humans became infected with SV40 when they received polio vaccine that was unknowingly contaminated with the virus. There have been suggestions that SV40 is linked with some types of human cancer, including primary brain cancer and malignant mesothelioma, but these suggestions are disputed.
23.4 EPSTEIN–BARR VIRUS-LINKED CANCERS
“Endemic” Burkitt’s lymphoma (BL) is a B cell tumor that occurs with a high frequency in children in central Africa and Papua New Guinea. Shortly after it was first described (by Denis Burkitt) Anthony Epstein established cell lines from the tumor of a patient. The cells were found to be persistently infected with a herpesvirus, which was named Epstein–Barr virus (EBV). Please see Chapter 11 for general characteristics of herpesviruses. Cases of “sporadic” BL and “HIV-associated” BL occur throughout the world, but generally without an association with EBV.
A consistent abnormality in BL tumor cells is a chromosomal rearrangement that results in the c-myc gene being placed next to an enhancer of an immunoglobulin gene. This results in the expression of c-myc at abnormally high levels. The chromosomal rearrangement is present in all cases of BL, irrespective of whether EBV is present or not. Perhaps EBV is one of several agents that can trigger the rearrangement.
Another tumor that has an association with EBV is nasopharyngeal carcinoma (NPC). This tumor, like BL, is more prevalent in particular regions (Figure 23.4). In both tumors the EBV genome is present in the tumor cells as cccDNA molecules. You will recall that when a herpesvirus infects a cell the linear virus genome becomes circularized (Section 11.5.1).
Figure 23.4 Incidence rates of nasopharyngeal carcinoma in males.
Source: Data (age standardized incidence rates per 100 000) published by Busson et al. (2004) Trends in Microbiology, 12, 356. Map drawn by V. Gaborieau and M. Corbex (Genetic Epidemiology Unit, International Agency for Research on Cancer). Reproduced by permission of Elsevier Limited and the authors.
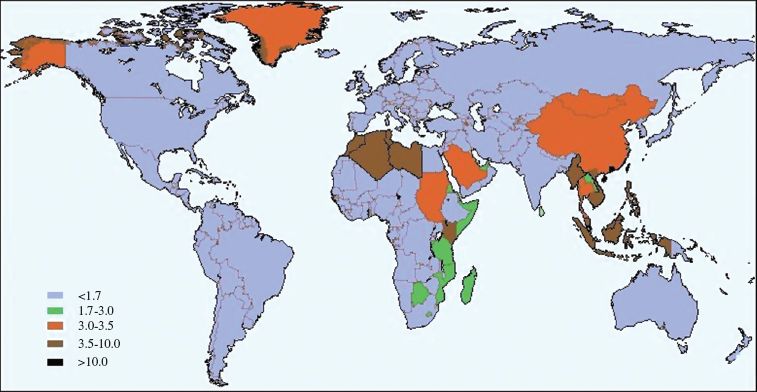
EBV is found worldwide and the majority of humans are infected with the virus. The factors that restrict high incidences of endemic BL and NPC to particular geographical locations are not understood. The endemic BL zone corresponds closely with the zone of malaria caused by Plasmodium falciparum, so this parasite may play a role. In southern China there are clusters of NPC in families, so there may be a human gene that increases the risk. Components of the diet, such as salted fish, are also suspected as co-factors in NPC.
A number of other cancers have been found to have associations with EBV, including:
- some cases of Hodgkin’s lymphoma;
- non-Hodgkin’s lymphoma in AIDS;
- post-transplant lymphoproliferative disorder.
The last two cancers develop in patients who are immunocompromised as a result of AIDS or immunosuppressive treatment, respectively.
The oncogenic potential of EBV can be demonstrated when cultures of B cells are infected with the virus. Infected cells synthesize a number of virus proteins that push the cells permanently into the cell cycle (Figure 4.5), resulting in the formation of lympho blastoid cell lines. In the body this B cell proliferation is normally controlled by T cells, but in individuals who are T cell immunocompromised the control may be inadequate and a B cell tumor may result.
23.5 KAPOSI’S SARCOMA
Kaposi’s sarcoma was first described in the nineteenth century as a rare skin cancer that affected elderly men in the Mediterranean region. Since the arrival of AIDS that picture has changed. It is one of the most common cancers in people with AIDS; in these patients the cancer is more aggressive and it disseminates throughout the body.
In 1994 it was discovered that the tumor cells contain the DNA of a herpesvirus. The virus was subsequently isolated and was named Kaposi’s sarcoma-associated herpesvirus (KSHV). It can be found in most parts of the world, but serological studies have shown that it is more common in certain regions, such as central Africa, and it is more common in homosexual men. The risk of developing Kaposi’s sarcoma parallels the prevalence of the virus in the population.
There is also good evidence linking KSHV with two other human cancers: primary effusion lymphoma and multicentric Castleman’s disease.
KSHV initiates latent infections in B cells and in endothelial cells lining blood vessels. If the host becomes immunocompromised one of these cells may be triggered into cycles of uncontrolled growth and division. If the cell is a B cell, then a primary effusion lymphoma or multicentric Castleman’s disease may ensue; if the cell is an endothelial cell, then the outcome may be Kaposi’s sarcoma. As with EBV-associated tumors, copies of the KSHV genome are present as cccDNA in the nuclei of the tumor cells.
23.6 ADULT T CELL LEUKEMIA
Adult T cell leukemia is associated with human T-lymphotropic virus 1 (HTLV-1) infection. Regions of the world with a high prevalence of the virus, such as southwest Japan, have a high prevalence of this cancer. HTLV-1 is a retrovirus and the tumor cells each have a copy of the proviral DNA integrated into a chromosome (Chapter 17).
23.7 HEPATOCELLULAR CARCINOMA
Hepatocellular carcinoma (liver cancer) accounts for 4–5% of cancer cases in the world. A number of factors are implicated as causative agents, including consumption of mold toxins in food, but most cases are associated with two viruses: hepatitis B virus (HBV) and hepatitis C virus (HCV). Liver cancer is the most common virus-associated cancer in men (Figure 23.1).
HBV is the most significant agent; the prevalence of liver cancer closely parallels the prevalence of persistent HBV infection (Section 19.2), with the highest incidences in Asia and in central and southern Africa. The other virus associated with liver cancer, HCV, is a flavivirus (Figure 22.5). It is the only class IV virus (plus-strand RNA virus) that is known to be oncogenic. Its genome is not found routinely in the cancer cells, in contrast to the other human oncogenic viruses.
Both HBV and HCV elicit immune responses when they infect the body. In some individuals the immune response successfully eliminates the infection, but in many cases the infection persists for life, as discussed in Section 19.2 for HBV. As far as HCV is concerned, it is estimated that about 75% of individuals who become infected are unable to eliminate the infection and that about 175 million people in the world are infected. In some individuals who are persistently infected with HBV or HCV, liver cirrhosis develops. This results in a high rate of cell division to replace the damaged hepatocytes, during which a mutation might occur in one of the cells, and this might eventually lead to cancer.
23.8 VIRUS-ASSOCIATED CANCERS IN ANIMALS
A number of cancers in animal species have been found to be associated with viruses. Outbreaks of some of these diseases can have serious economic consequences in agriculture. A few examples are given here.
It was demonstrated early in the twentieth century that filterable agents cause a leukosis (a B cell leu kemia/lymphoma) and a sarcoma in chickens. These agents were subsequently shown to be retroviruses; the sarcoma-causing virus was named Rous sarcoma virus after its discoverer, Peyton Rous (Section 17.4). Other retroviruses were subsequently found to be causative agents of leukemia in cats and cattle, and a retrovirus known as Jaagsiekte sheep retrovirus was found to be responsible for adenocarcinoma of the lung in sheep.
Marek’s disease in chickens is a lymphoma, similar to that caused by retroviruses, but the causative agent is a herpesvirus and the transformed lymphocytes contain multiple copies of the viral genome. Papillomaviruses, already discussed as causative agents of human cancers (Section 23.2), cause cancers in cattle, horses, and rabbits.
23.9 CELL LINES DERIVED FROM VIRUS-ASSOCIATED CANCERS
Cancer cells, which by definition multiply continuously in the body, will often continue to multiply continuously if transferred into cell culture, when permanent cell lines may be established from them. Cell lines derived from most virus-induced cancers contain part or all of the virus genome. The most famous of these, the HeLa cell line, was established from a cervical cancer in the mid-twentieth century and is now used in laboratories around the world. Each HeLa cell contains a copy of part of the genome of HPV-18; this will be discussed further in Section 23.10.5.
Cell lines derived from Burkitt’s lymphoma contain copies of the EBV genome and some produce infectious EBV; the virus was first discovered in one of these cell lines. Multiple copies of Marek’s disease virus genome are present in a cell line derived from a Marek’s tumor. A cell line derived from a liver cancer contains HBV DNA integrated at seven sites, and the cells secrete HBsAg. Cell lines derived from Kaposi’s sarcoma, however, do not contain KSHV DNA; the virus DNA is lost from the tumor cells when they grow in culture.
23.10 HOW DO VIRUSES CAUSE CANCER?
Most virus-induced cancers develop after a long period of persistent infection with an oncogenic virus; for adult T cell leukemia this period is exceptionally long (around 60 years). The virus infections persist in their hosts in spite of immune responses, such as the production of virus-specific antibodies. Some persistent infections are latent for much of the time (e.g. EBV and KSHV), with only small numbers of virus genes expressed. Others, including HBV and HCV infections, are productive. Both of the latter viruses are able to evolve rapidly (Chapter 21), and this probably allows them to keep one step ahead of acquired immune responses.
Although many humans are persistently infected by viruses that are potentially oncogenic, only small percentages of those infected develop virus-linked cancers. Relatively few people develop EBV-related tumors, though over 90% of adults worldwide are infected with the virus. About 3% of women persistently infected with one of the high-risk strains of HPV develop cervical carcinoma, and similar percentages of individuals persistently infected with HBV, HCV, or HTLV-1 develop a virus-linked cancer.
As cancer develops in only a small proportion of virus-infected hosts it is clear that the virus infections alone do not cause cancer. Other factors are involved and these include exposure to particular environmental factors, host genetic factors, and immunodeficiency.
Immunodeficiency increases the risk of a virus-associated tumor, the nature of the immunodeficiency influencing the types of tumor that may develop. AIDS patients are much more likely to develop Kaposi’s sarcoma than immunosuppressed transplant recipients. Non-Hodgkin’s lymphoma in AIDS and post-transplant lymphoproliferative disorder (EBV-related tumors; Section 23.4) each occurs in a distinct class of immunodeficient patient. Immunodeficiency does not increase the risk of all tumors with a viral link; for example, there is no increased incidence in AIDS patients of NPC or of liver cancer.
The probability of cancer developing in a host infected with an oncogenic virus therefore depends on a complex interplay between the state of the host, environmental factors to which the host is exposed, and cellular changes induced by the virus infection. There is increasing evidence that virus proteins synthesized during persistent infections play roles in the conversion of normal cells to cancer cells. In some cases virus proteins “deliberately” interfere with cell activities in order to provide appropriate conditions for virus replication. In other cases virus proteins may “accidentally” interact with cell components with outcomes that are of no benefit to the virus. The roles of virus proteins in the development of cancers are discussed in the next sections.
23.10.1 “Deliberate” interference with control of cell activities
A virus needs to manipulate the internal environment of its host cell so that all the requirements for virus replication are fulfilled. A requirement for small DNA viruses of eukaryotes (papillomaviruses, polyomaviruses, and adenoviruses) is that the cell’s DNA synthesizing functions are available (i.e., that the cell is in the S phase of the cell cycle; Figure 4.5).
Many cells in the animal body grow and divide either slowly or not at all; the latter are arrested in the G1 phase of the cell cycle and are said to be in the G0 state. The control of the cell cycle is mediated by many proteins; two that play key roles in humans are p53 and retinoblastoma protein (pRb). The latter protein is so named because the absence of the protein in some individuals, due to mutations in both copies of the gene, leads to the development of retinoblastoma (cancer of the retina). It has been demonstrated that several proteins produced by oncogenic viruses can interact with p53, pRb, and other proteins that control cell growth and division, increasing the probability of a cell being pushed into repeated cycles of division.
Virus proteins that can interfere with control of the cell cycle include the HPV early proteins E6 and E7, which localize to the nucleus and bind to cell proteins. E6 binds to p53, promoting its degradation, and E7 binds to pRb–E2F complexes, causing their dissociation (E2F is a cell transcription factor that activates DNA synthesis). The outcome of these interactions is the transition of the cell to the S phase.
Many other proteins of viruses considered in this chapter can interact with p53 and/or pRB. Examples include:
- SV40 large T antigen (binds to p53 and pRB);
- KSHV latency-associated nuclear antigen (binds to p53 and pRB);
- HTLV-1 Tax protein (binds to p53);
- HBV X protein (binds to p53);
- Adenovirus E1A protein (binds to pRB).
As well as affecting the cell cycle, some viruses may interfere with attempts by the infected cell to destroy itself via one of the apoptosis pathways. The cell protein p53 is involved in some of these pathways, which may be blocked as a result of a virus protein binding to p53.
23.10.2 “Accidental” activation of cell genes
Some virus proteins are able to bind to cell proteins that may not be the intended targets and may thus trigger events that are of no value to the virus, but may be harmful to the host. A virus protein might inadvertently push a cell toward a cancerous state by activating a cell gene that is switched off, or by enhancing the transcription of a gene that is normally expressed at a low level.
Some retroviruses can induce tumor formation as a consequence of integrating their provirus into a cell chromosome at a site that puts a cell gene under the control of a virus promoter (Figure 23.5). Some cell genes that can be activated in this way are known as proto-oncogenes because their activation may result in a cancer. The proteins encoded by proto-oncogenes are generally involved in gene regulation or cell cycle control. An example of a proto-oncogene is c-myc. Infection of a chicken with an avian leukosis virus (Section 23.8) can cause a tumor when proviral integration next to c-myc results in greatly enhanced transcription of this gene. There were unfortunate outcomes in some gene therapy trials as a result of proto-oncogene activation by a retrovirus. A retroviral vector was used to introduce a gene into the patients’ cells and some of the patients went on to develop leukemia.
Figure 23.5 Activation of a cell gene by insertion of a retroviral provirus. Initiation of transcription at either of the provirus LTRs may lead to expression of the cell gene.
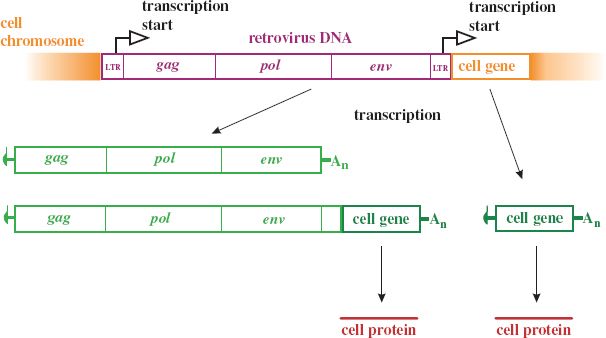
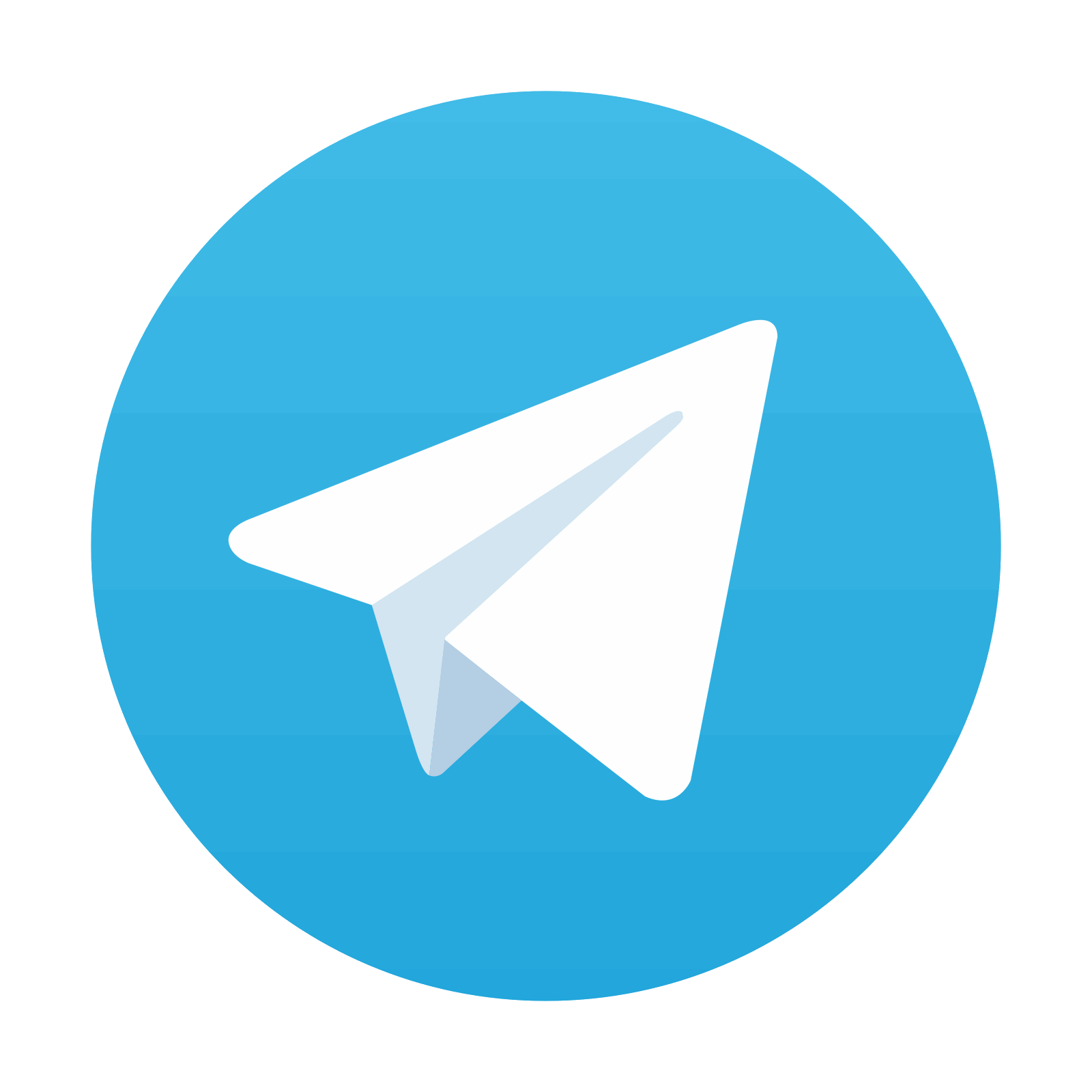
Stay updated, free articles. Join our Telegram channel

Full access? Get Clinical Tree
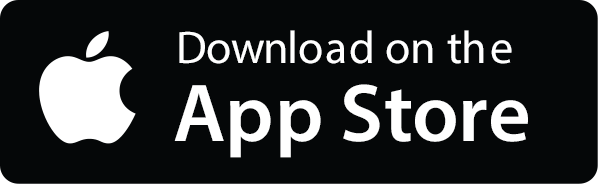
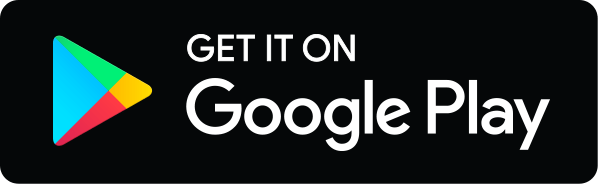