Cell type
Advantages
Disadvantages
MNC
Multipotent and autologous
Limited expansion and high cell heterogeneity
Skeletal myoblast
Autologous, easy isolation and expansion
Arrhythmogenic and lack of electromechanical coupling
MSC
Multipotent, autologous, lack of immunogenicity, and cryopreservable
Invasive and painful isolation, risk of infection (bone marrow), require expansion, and sometimes adverse events following cell implantation (e.g., calcification and bone formation)
CPC
Autologous, high expansion, cardiomyocyte phenotype, and electromechanical compatible
Limited availability and survival
ESC
Totipotent and high expansion
Teratoma formation following cell implantation, host immune response, and both legal and ethical refusal
iPSC
Pluripotent, autologous, epigenetically and functionally indistinguishable from ESCs, acceptable availability, and neither legal nor ethical constraints
Teratoma formation
In addition, totipotent embryonic stem cells (ESCs) derived from the inner cell mass of blastocysts are nonautologous but can differentiate toward cardiomyocyte-like cells that are electrically connected to the surrounding myocardium without triggering arrhythmias [23]. Although the use of ESCs is at present restricted in many countries because of both legal and social barriers, the implementation of advanced genetic reprogramming techniques to generate cytoplasmic hybrids, or cyhibrids, which are created by taking an egg from a nonhuman animal and removing the nuclear DNA, leaving only the cytoplasm or ooplasm of the animal egg, should help create a more ethical source of “stem” or progenitor cells [24]. However, the undifferentiated state of ESCs is currently associated with a risk of teratoma formation [25]. In contrast to ESCs, there have been no ethical objections raised in relation to the use of induced pluripotent stem cells (iPSCs), which are artificially derived from adult somatic cells through the induction of an embryonic gene profile and are thought to be equivalent to ESCs with regard to doubling time, differentiability, and (unfortunately) teratoma formation [15].
In the clinical arena, despite being safe and technologically feasible, cellular cardiomyoplasty cannot be used successfully (e.g., after MI) because of the formation of a noncontractile scar and extreme ischemia [26–29]. Taken together, clinical advances are not as solid or conclusive as advances in animal models (generally rodents and pigs), which have provided the proof of concept for the regeneration of damaged cardiac tissue [30]. For instance, data reported from acute and chronic MI patients infused with bone marrow-derived cells through intracoronary or intramyocardial delivery routes regarding improvement of the left ventricular ejection fraction, local contractility, and reduction of infarct size have been modest at best, mainly because of low cell engraftment and survival within the fibrous myocardium [31–52]. Thus, addressing paramount issues, such as optimal dose and delivery system, as well as increasing cell homogeneity and functionality (e.g., declining to harvest cells from patients that exhibit cardiovascular risk), remain major challenges to increasing the efficacy of future cell infusion studies. For this purpose, additional details about both clinical and functional outcomes of ongoing trials, such as NCT00462774 (Cardio 133), NCT00529932 (SELECT-AMI), NCT01625949 (COAT), and NCT01414452 (R2ACE), are awaited with great interest [www.clinicaltrials.org].
Novel therapeutic strategies have recently been developed to deliver therapeutic cells in combination with biomaterials, growth factors, and advanced monitoring devices (“tissue engineering”) [18, 53]. Although the results using these techniques, which aim to re-establish electromechanical coupling, stable contractile function, and functional vascularization, are clearly promising, the challenges are enormous. A majority of these experimental procedures have only been tested in small animal models because the major disadvantage is the difficulty of extrapolating to the porcine model (more similar to humans) and, consequently, to the clinical context. The size of the human heart makes these procedures unviable because of the limited matrix dimensions and number of cells that can actually be implanted. Thus, there are few trials currently employing tissue engineering for cardiac regeneration in the clinical context; such trials include the intramyocardial application of alginate (NCT01311791) and the intracoronary delivery of a combination of sodium alginate and calcium gluconate (NCT01226563).
As previously mentioned, therapeutic procedures that are able to strongly support blood supply to injured tissues, including infarcted myocardium, will be more useful for human regenerative medicine. Thus, the possibility of applying vascular precursor cells belonging to the endothelial lineage draws great deal of attention. However, the identification and isolation of genuine vascular precursors from different biological sources (e.g., bone marrow, peripheral blood, UCB, and fetal liver [54–57]) has been the subject of recurring debate since the key findings published in the 1990s by Asahara and colleagues [58–61]. For that reason, and prior to the obtaining of more homogeneous, clonogenic, and proliferative hierarchies of progenitor cells committed to the endothelial lineage, alternative cell sources have been investigated. Basically, these include ESCs [62] and both HPCs and MSCs derived from bone marrow [63–65], adipose tissue [66], and (more recently) UCB [67–69]. The most relevant questions about the role and application of UCB-derived progenitor cells in multiple human disorders are described below.
3 Umbilical Cord Blood as Source of Regenerative Cells for Multiple Clinical Settings
The continuous growth of the global population, which is expected to remain at a constant level of more than 130 million annual births according to an estimate from the United States Census Bureau, is one of the numerous reasons why UCB is currently recognized as the richest source of “stem” or progenitor cells for a vast number of regenerative applications in humans [70]. Compared with other unrelated donor cell sources, UCB can also be obtained safely and painlessly, can be long-term cryopreserved without loss of cell viability, has low risk of transmitting viral infections and somatic mutations, and its application is not subject to the ethical restrictions that complicate the use of other sources [71]. Moreover, there is considerable support for UCB collection, donation, and storage from the major world religions [72].
Since Broxmeyer, Gluckman, and colleagues reported convincing evidence in the 1980s, there has been an increasing consensus that UCB could be used in clinical settings for hematopoietic cell transplantation [73]. In particular, Broxmeyer et al. were the first to firmly demonstrate that UCB is a rich source of HPCs [74]; in the same year, Gluckman et al. documented the first hematopoietic cell transplant to use UCB instead of bone marrow as the source of hematopoietic cells. Remarkably, they were able to reconstitute the hematopoietic system of a child with Fanconi anemia using UCB from an HLA-identical sibling [75]. However, the earliest evidence dates from 1974, when Knudtzon demonstrated the presence of relatively mature HPCs in human UCB [76]. About 10 years later, Ogawa and colleagues reported the presence of more primitive subpopulations of HPCs in UCB [77, 78]. Since then, evidence has continued to accumulate and a global network of UCB banks has been established worldwide; UCB has become one of the most commonly used sources for allogeneic transplant of HPCs for adults and children afflicted by hematological disorders, with an estimated 600,000 samples collected and more than 20,000 UCB units distributed around the world [70].
The spectrum of diseases against which UCB-derived hematopoietic progenitors are effective has been expanded to include bone marrow failure, hemoglobinopathies, and inborn errors of metabolism, among other human disorders. A variety of possible new applications are being investigated as emerging cell therapies, many which are being tested in multiple clinical trials. This active field of investigation includes ex vivo expansion of UCB-derived hematological progenitors (NCT00343798, NCT01175785, and NCT00498316), neurological improvements in cerebral palsy (NCT01072370, NCT01147653, and NCT01528436), neonatal ischemic encephalopathy (NCT00593242), orthopedic cartilage repair (NCT01041001), and treatment of metabolic diseases such as type I diabetes (NCT00305344 and NCT00873925) [19]. Furthermore, owing to its enrichment in vascular progenitors [79], angiogenesis was induced in a 27-year-old woman with Behçet’s multisystemic disease [80] and in autistic children [81] receiving cells derived from UCB.
In addition to current clinical trials, UCB (and particularly UCB-derived MSCs) are undergoing powerful preclinical and clinical research in the cardiovascular area ranging from MI to stroke [68, 82–85]. For instance, Leor and co-workers showed that intravenous infusion of human UCB-derived CD133+ cells promotes functional recovery by preventing scar thinning and left ventricular systolic dilatation after an extensive MI in rats [83]. In 2008, Ichim et al. presented a case report of a patient suffering dilated cardiomyopathy who was treated with intravenous allogeneic MSCs and expanded UCB CD34+ cells and experienced profound clinical improvement [85].
4 Past and Current Investigations Using UCB-Derived Cells in the Cardiovascular Area
In addition to being a source of HPCs, UCB also contains MSCs at a lower frequency similar to that of other human tissues, including bone marrow [86], adipose tissue [87], placenta [88], dermal tissues [89], and umbilical cord [90]. These cells are recognized by their capability to differentiate into osteogenic, chondrogenic, and adipogenic lineages in vitro, typical mesenchymal-like morphology, plastic-adherence when maintained in standard culture conditions, and exhibition of a nonhematopoietic cell surface pattern, according to the International Society of Cellular Therapy criteria [91]. However, distinct populations of mesenchymal-like stem cells with similar adhesion properties and antigen surface expression patterns, but different pluripotencies, have been isolated from UCB [19]. Briefly, Kogler et al. described intrinsically pluripotent or unrestricted somatic “stem” cells with the potential to reprogram into mesodermal, endodermal, and ectodermal fates [92]. Subsequently, other investigators used MSCs with more restricted pluripotency [93, 94].
A decade ago, Erices and colleagues investigated the ability of transplanted human UCB-derived MSCs to home in the bone marrow of unconditioned nude mice. As a result, after systemic infusion, they detected human DNA in cardiac muscle, as well as in other recipient tissues [95]. Since then, one central unanswered question is whether these cells have cardiomyogenic potential [19]. Although several investigators have reported the differentiation of UCB-derived MSCs into the cardiomyogenic lineage in vitro [96, 97], others have failed to demonstrate such differentiation [98, 99] using a broad range of pro-cardiogenic stimuli, including 5-azacytidine [96, 100], dimethyl sulfoxide [101], a combination of growth factors involved in early cardiomyogenesis [102], activation of Wnt signaling pathways [101, 103], and coculture with neonatal rat cardiomyocytes [104, 105]. A coculture system using rat primary cardiomyocytes had been effective in inducing a cardiomyocyte-like phenotype in UCB-derived CD133+ hematopoietic progenitors that were first selected from UCB by immunomagnetic separation and then expanded by stimulation with platelet-derived and epidermal growth factors [106]. When cultured for up to 4 weeks in a cardiac differentiation-promoting medium, this same cell population gained the expression of certain cardiac-specific markers (e.g., α-actin, myosin heavy chain, and troponin I) in association with the upregulation of the cardiac-specific transcription factors GATA-4 and MEF2C and the nuclear receptor transcription factors PPARα, PPARγ, RXRα, and RXRβ [107]. However, other authors did not find that direct contact with neonatal rat cardiomyocytes promoted either the expression of cardiomyocyte-specific proteins or the presence of rhythmic calcium oscillations and potential-dependent fluorescence emission in UCB-derived MSCs [99]. Thus, they concluded that alternative strategies, regulatory factors, or signaling clues might be better suited to recruit UCB-derived MSCs into the cardiac cell lineage. Surprisingly, a nonhematopoietic cellular subpopulation within the mononuclear cell fraction isolated from UCB was differentiated toward the cardiomyogenic lineage after these cells were cocultured with brown adipose tissue-derived cells [108]. Additional details about the cardiomyogenic potential of UCB-derived MSCs from ongoing investigations in both preclinical and clinical scenarios will definitively answer this crucial question.
The vascular potential of both HPCs and MSCs derived from UCB has garnered a great deal of attention for therapeutic purposes (Fig. 26.1 ). In 2005, Ma et al. described hematopoietic precursors isolated from UCB that were responsible for increasing capillary density after MI in mice [67]. These authors observed that mononuclear cells (containing 0.11–1.1 % CD34+ cells) injected into the tail vein of recipient animals migrated to the infarcted myocardium, where they engrafted, participated in neoangiogenesis, and beneficially influenced remodeling processes. Intramyocardially administered CD105+ MSCs also exhibited favorable survival in infarcted mouse hearts, which translates into better capillary density in both border and remote zones 6 weeks postinfarction and more robust preservation of cardiac function [109]. Lee et al. have recently found that N-cadherin determines individual variations in the therapeutic efficacy of human UCB-derived MSCs in a rat MI model, and that variations in capillary density are correlated with therapeutic efficacy in improving left ventricular function after MI [110].


Fig. 26.1
Potential of UCB-derived “stem” or progenitor cells for human regenerative medicine. Since Gluckman et al. reconstituted the hematopoietic system of a child afflicted by Fanconi anemia in 1989, UCB has become one of the most used source for allogeneic transplant of HPCs against multiple hematological disorders. In addition, there is increasing evidences from animal models that point out UCB as a promising source of nonhematopoietic progenitor cells (endothelial progenitors and MSCs) for blood vessel growth and to develop vascular grafts and heart valve leaflets. For instance, they could be useful for cardiac regeneration following MI
Nondestructive monitoring techniques like bioluminescence imaging (BLI), which is based on the application of natural reactants with light-emitting capabilities (photoproteins and luciferases), currently provide valuable information about the location and functional status of regenerative cells implanted into numerous animal models of cardiovascular disease [111]. Notably, BLI was used by Roura et al. to follow the expression of chimeric luciferase/fluorescent proteins by human UCB-derived MSCs in a mouse model of angiogenesis [19]. Their results revealed that the promoter of endothelial cell marker CD31 was highly activated in the injected human cells, suggesting efficient cell differentiation; the injected cells also self-organized into new functional blood vessels connected with the circulatory system in the recipient animals. In this study, the administered cells also carried another chimeric reporter of “cell number” to evaluate cell survival over time. Once again, BLI in combination with advanced reporter gene technology allowed basic researchers to illuminate current efforts in cardiac regeneration, where the future is bright (Fig. 26.2 ). More recently, these same authors employed these genetically modified UCB-derived MSCs embedded in a fibrin patch to assess the effect of their implantation after acute MI in mice [69]. They have demonstrated that UCB-derived MSCs of the engineered construct survived for 4 weeks postimplantation and organized into CD31+ network structures over the infarcted myocardium. MI-treated animals also exhibited a reduced infarct scar and a larger vessel density in comparison with MI-control animals.


Fig. 26.2
Use of UCB-derived MSCs to validate efforts in cardiac regeneration. By using advanced reporter gene technology and BLI, researchers can first label UCB-derived MSCs and subsequently perform a noninvasive monitoring of cell survival and differentiation in the context of cell-based therapies. Accordingly, implanted cell retention and therapeutic outcomes can be correlated and the most suitable cell type and dose determined
Numerous authors have also proposed using UCB-derived cells and biodegradable scaffold materials for cardiovascular tissue engineering [112, 113]. For instance, UCB-derived endothelial progenitors exhibited exceptional growth characteristics appropriate to the development of heart valve leaflets and vascular grafts [114, 115].
5 Conclusions and Future Perspectives
Unlike other vertebrate animals (e.g., salamanders, zebra fish and axolotl), the mammalian heart has limited regenerative potential. A growing body of evidence indicates that cell therapy, together with endogenous stimulation by selected micro RNAs [116–118], is an important regime to repair myocardium postinjury. This regenerative procedure, which has garnered expanding interest in the clinical setting, is based on the implantation of cells that are able to promote myocardial revascularization, reduce ventricular remodeling and cardiomyocyte apoptosis, and improve global left ventricular function. Although ESCs are capable of unlimited self-renewal and have the potential to give rise to all tissue types in the body, ethical difficulties and technical hurdles may limit their application. Meanwhile, adult and perinatal “stem” or progenitor cells, which have been widely used to reconstitute the hematopoietic system in humans, might represent a valuable alternative.
Although numerous clinical trials have already demonstrated the indisputable safety and feasibility of cardiac cell therapy, it is necessary to address many extremely important issues, such as the optimal cell type, route of delivery, and time point of administration. Thus, additional multicenter studies are ongoing in an attempt to resolve these questions and to reveal true benefit in clinical outcomes. Notably, increasing the number of introduced cells that are retained in the injured myocardium is the key challenge for developing more effective treatments. However, it is mandatory to understand that “stem” or progenitor cells are not an alternative to cardiac transplantation; ideal patients should be at an early stage of heart dysfunction, as the overarching aim of this regenerative approach is to avoid or delay organ transplantation. Because providing a proper niche for implanted cell survival and function is of paramount importance, the most realistic outlook includes the use of tissue-engineered scaffolds or matrices to evolve from an inert cell delivery approach to a more dynamic and efficient regenerative product. Increasing both experimental and clinical experience using these approaches to deliver cells with cardiac potential, including UCB-derived cells, are emerging as novel strategies to treat an old problem, as Friedrich and Böhm envisioned 8 years ago [119].
References
1.
Cleland JG, Khand A, Clark A. The heart failure epidemic: exactly how big is it? Eur Heart J. 2001;22:623–6.PubMed
2.
Cines DB, Pollak ES, Buck CA, Loscalzo J, Zimmerman GA, McEver RP, et al. Endothelial cells in physiology and in the pathophysiology of vascular disorders. Blood. 1998;91:3527–61.PubMed
3.
Pfeffer MA, Braunwald E. Ventricular remodeling after myocardial infarction. Experimental observations and clinical implications. Circulation. 1990;81:1161–72.PubMed
4.
Anversa P, Kajstura J. Ventricular myocytes are not terminally differentiated in the adult mammalian heart. Circ Res. 1998;83:1–14.PubMed
5.
Beltrami A, Urbanek K, Kajstura J, Jan SM, Finato N, Bussani R, et al. Evidence that human cardiac myocytes divide after myocardial infarction. N Engl J Med. 2001;344:1750–7.PubMed
6.
Anversa P, Nadal-Ginard B. Myocyte renewal and ventricular remodelling. Nature. 2002;415:240–3.PubMed
7.
Quaini F, Urbanek K, Beltrami AP, Finato N, Beltrami CA, Nadal-Ginard B, et al. Chimerism of the transplanted heart. N Engl J Med. 2002;346:5–15.PubMed
8.
Laflamme MA, Myerson D, Saffitz JE, Murry CE. Evidence for cardiomyocyte repopulation by extracardiac progenitors in transplanted human hearts. Circ Res. 2002;90:634–40.PubMed
9.
Bayes-Genis A, Salido M, Solé Ristol F, Puig M, Brossa V, Campreciós M, et al. Host cell-derived cardiomyocytes in sex-mismatch cardiac allografts. Cardiovasc Res. 2002;56:404–10.PubMed
10.
Bayes-Genis A, Muñiz-Diaz E, Catasus L, Arilla M, Rodriguez C, Sierra J, et al. Cardiac chimerism in recipients of peripheral-blood and bone marrow stem cells. Eur J Heart Fail. 2004;6:399–402.PubMed
11.
Bayes-Genis A, Bellosillo B, de la Calle O, Salido M, Roura S, Ristol FS, et al. Identification of male cardiomyocytes of extracardiac origin in the hearts of women with male progeny: male fetal cell microchimerism of the heart. J Heart Lung Transplant. 2005;24:2179–83.PubMed
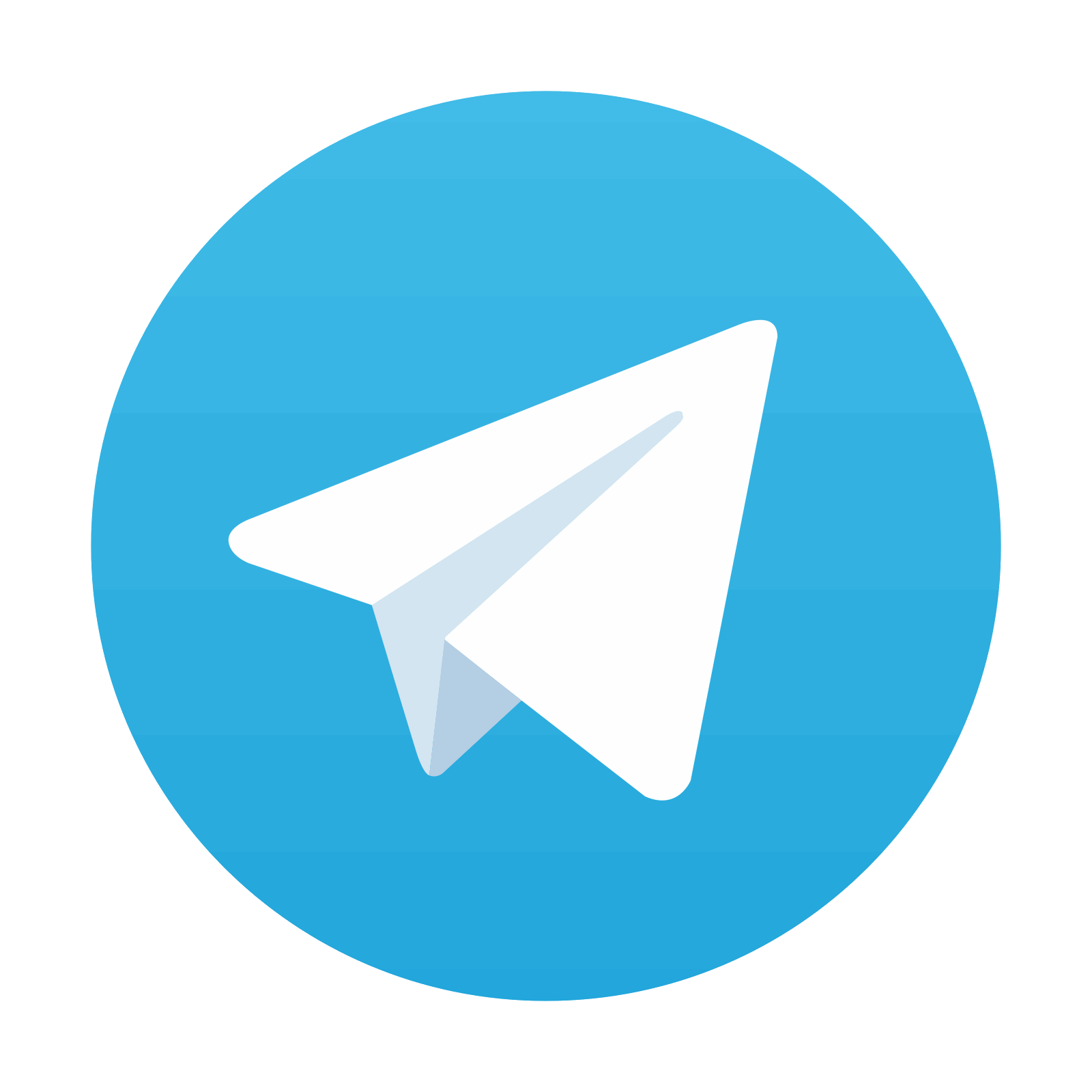
Stay updated, free articles. Join our Telegram channel

Full access? Get Clinical Tree
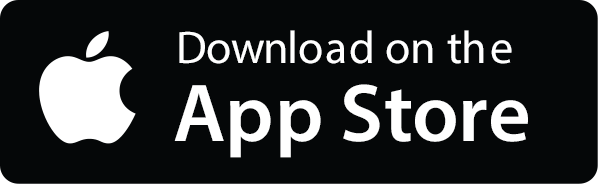
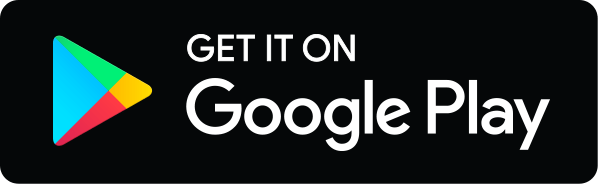