© Springer Science+Business Media New York 2014
Anthony Atala and Sean V. Murphy (eds.)Perinatal Stem Cells10.1007/978-1-4939-1118-9_3131. Towards Clinical Applications of Umbilical Cord Derived Mesenchymal Stem Cells
Rouzbeh R. Taghizadeh1 , Paul W. Holzer2, Teresa Marino3, Kyle J. Cetrulo1 , Curtis L. CetruloSr.4 and Curtis L. CetruloJr.5
(1)
AuxoCell Laboratories, Inc., 245 First Street Suite 1800, Cambridge, MA 02142, USA
(2)
Transplantation Biology Research Center (TBRC), Massachusetts General Hospital, 142 Commercial Street, Apt # 304, Boston, MA 02109, USA
(3)
Obstetrics and Gynecology, Tufts Medical Center, 800 Washington Street, Boston, MA 02111, USA
(4)
Maternal Fetal Medicine, Obstetrics and Gynecology, Tufts Medical Center, Tufts University School of Medicine, Washington Street, Boston, MA 02111, USA
(5)
Division of Plastic and Reconstructive Surgery, Massachusetts General Hospital, Boston, MA, USA
Abstract
Stem cells hold great promise for treating and, ultimately, curing diseases that are currently being managed with pharmaceutical intervention to control the disease progression and/or disease-related manifestations. Stem cells can be used to regenerate normal cells and tissue, while replacing diseased cells and tissue, in order to restore homeostasis and normal tissue function. Although, there is currently great ethical debate concerning embryonic stem cells—specifically in regards to the dilemma of terminating (potential) life, in order to derive stem cells that could be used to treat and potentially cure another ailing individual—no such ethical quandaries exist with adult stem cell sources—with appropriate patient education and consent. In fact, adult stem cell treatments have been in use for more than 50 years, beginning with the first bone marrow transplants spearheaded in the 1950s to organ transplantations of today. As the stem cell field moves forward towards the next generation of cell-based and regenerative medicine therapies, a new paradigm is being explored as a valuable source of primitive stem cells—otherwise known as perinatal stem cells. This chapter explores, in depth, the potential clinical use and benefit of perinatal stem cells and analogous regenerative medicine therapies sourced from the umbilical cord tissue and Wharton’s Jelly.
1 Introduction
Over the past several decades, there has been increasing interest in perinatal stem cells for use in the clinic, as a cell-based therapy and as the starting material for regenerative medicine-based products. In the broader sense, perinatal stem cells are stem cells derived from the various tissue sources related to pregnancy. These sources include umbilical cord blood (UCB), umbilical cord (UC) tissue/Wharton’s Jelly (WJ), amniotic fluid, amnion, placental tissue, and placental blood. Specifically, perinatal stem cells can refer to stem cells that are derived from the fetus or mother, depending on the genetic origin of the after-birth tissue.
Perinatal stem cells are neither embryonic stem cells (ESCs) nor are they adult (somatic) stem cells (ASCs). Perinatal stem cells represent a new paradigm that offers properties of both embryonic and ASCs. For instance, perinatal stem cells are more pluripotent than ASCs, but are not totipotent like ESCs. Mesenchymal stem cells (MSCs) derived from Wharton’s Jelly (WJ-MSC), for instance, express genes regulated in human ESCs (e.g., Oct-4, nanog, sox-2), whose expression relates to maintaining pluripotency of human ESCs. Furthermore, these genes are regulated in deriving induced pluripotent stem (iPS) cells from somatic cells. Interestingly, however, these genes are not expressed in ASC sources (e.g., bone marrow, BM). Unlike human ESCs and like ASCs, perinatal stem cells are not tumorigenic. Like human ESCs, perinatal stem cells have long telomeres that allow for greater regenerative potential before reaching senescence. ASCs have shorter telomeres and, therefore, have limited regenerative potential since they reach senescence relatively faster [55, 59]. In turn, perinatal stem cells represent a stem cell source with properties of both human ESC and ASCs, without the misgivings of either source. This chapter focuses on one specific perinatal stem cell source—MSCs derived from the Wharton’s Jelly of the umbilical cord.
2 Umbilical Cord Mesenchymal Stem Cells for Use in Hematopoietic Reconstitution
Perinatal MSCs derived from the Wharton’s Jelly of the umbilical cord tissue possess widespread clinical applications—from (stem) cell-based to regenerative medicine therapies. In the context of this chapter, (stem) cell-based therapies refers to clinical use of primary cell populations—derived under minimal manipulation (e.g., UCB); regenerative medicine therapies refers to stem cell populations that have been culture expanded and/or genetically or otherwise biologically modified (e.g., Osiris’ Prochymal® off-the-shelf bone marrow MSC product).
For (stem) cell-based therapies, umbilical cord tissue derived MSCs have therapeutic benefit in UCB-related transplantations. UCB is a great perinatal source of blood forming stem cells, otherwise known as hematopoietic stem cells (HSCs). Currently, UCB derived HSCs are used in the treatment of a host of blood-related diseases due to the ability of these cells to reconstitute the blood and immune system. A major drawback of UCB and UCB-related transplantations, however, is the finite volume of cord blood that can be collected. The collection of UCB can occur at only one point in time—at delivery. After the fetus has been delivered, the fetal end of the umbilical cord is clamped and cut—thereby, physically detaching the fetus from the mother. If the UCB is to be collected, a needle attached to a blood collection bag with an anticoagulant solution (e.g., citrate phosphate dextrose, CPD) is inserted into the umbilical cord vein—the largest vessel of the three vessels within the umbilical cord. The umbilical cord vein is “milked” until blood flow from the collection has ceased. Due to the finite (and variable) volume of UCB that can be collected at delivery, a finite number of stem cells can be derived from a single UCB collection—as the UCB volume directly correlates with the number of attainable HSCs. The higher the number of HSCs available for a transplant (i.e., dose), the more efficient the UCB unit can engraft when transplanted. Numerous methods are currently under investigation to increase the efficiency of UCB transplantations, including ex vivo HSC expansion technologies, increasing homing capacity to the marrow cavity, direct intra-femoral injections, and multiple unit transplantations. A seemingly more straightforward method takes advantage of the unique properties of another important stem cell population—MSCs—in the setting of co-transplantation with an UCB unit [57].
The rationale for the use of MSCs to enhance engraftment of HSCs is several-fold. First, the current gold standard source in attaining the most-efficient hematopoietic engraftment is bone marrow. If a bone marrow unit is available—either from one’s own disease-free bone marrow (autologous) or from a healthy HLA (human leukocyte antigen) matched donor (allogeneic), it is the preferred (stem) cell source for hematopoietic reconstitution. The primary reason for this is the relatively quick time to hematopoietic engraftment—determined by time to absolute neutrophil counts (ANC) above 500 (per microliter blood) for three consecutive days and megakaryocyte counts between 20,000 and 50,000 (per microliter blood) [69]. On average, bone marrow units reach this ANC in 18 days [26, 58]. This is significantly better than current UCB unit transplantations, where time to neutrophil engraftment takes 22–27 days, on average [26, 58]. The reason for this discrepancy is possibly explained by the following concept: bone marrow contains HSCs and MSCs, as well as differentiated progeny of each stem cell population, whereas UCB primarily contains HSCs and its differentiated progeny. When bone marrow is transplanted from cells derived from either an autologous source or from a HLA-matched donor, both HSCs and MSCs are transplanted. This is significant since HSCs and MSCs work in concert to maintain proper blood and immune cell production and function to sustain life and proper homeostasis. UCB, on the other hand, is a rich source of blood forming HSCs, but a poor source of MSCs. MSCs are protein factories that provide support for blood and immune function, by producing growth factors and cytokines that signal and cue HSCs to perform a specific function to maintain proper hematopoietic activity. Due to this interdependent relationship, it has recently been found that MSCs may also play a critical role in engraftment and maintenance of proper blood formation when co-transplanted into patients with diseased and damaged blood [57].
MSCs exist in adult bone marrow (BM-MSCs), as previously described, and adipose (fat) tissue, but also can be found in after-birth tissue—specifically within the Wharton’s Jelly of the umbilical cord tissue. The human umbilical cord contains three vessels—one umbilical vein and two umbilical arteries. The tissue surrounding the vessels is referred to as Wharton’s Jelly. Wharton’s Jelly is the primitive mucous, connective tissue of the umbilical cord lying between the amniotic epithelium and the umbilical vessels. This gelatinous substance, first observed by Thomas Wharton in 1656, is comprised of proteoglycans and various collagen types. The principle function of the Wharton’s Jelly is to prevent the compression, torsion, and bending of the umbilical vessels which provide the bi-directions flow of oxygen, glucose, and amino acids to the developing fetus, while also depleting the fetus and placenta of carbon dioxide and other waste products [6, 59, 61]. Cells found in Wharton’s Jelly are a primitive MSC, likely trapped in the connective tissue matrix as they migrated to the AGM (aorta-gonad-mesonephros) region through the developing cord, during embryogenesis (prior to E10.5) [61].
Wharton’s Jelly has powerful therapeutic benefits, especially since UC is a rich source of a perinatal, potent population of MSCs. More specifically, WJ derived MSCs have immediate therapeutic benefit in potentially increasing the engraftment efficiency of UCB—to the levels currently observed with bone marrow. This hypothesis rests on the principle that UCB possesses a rich supply of HSCs, but lacks a significant population of MSCs [10, 51, 55]. In the human bone marrow cavity, MSCs produce and secrete various factors that provide support and promote HSC mitotic activity, survival, expansion, differentiation, self-renewal, as well as maintaining multilineage differentiation of mesodermal cells (e.g., osteocytes, adipocytes, chondrocytes). The simple absence of significant numbers of MSCs in UCB may be the biological key in increasing the engraftment efficiency and potential use of UCB derived HSCs without the dependence of any of the aforementioned ex vivo manipulation technologies or need for multiple unit infusions. Although a significant population of MSCs are absent from UCB, the cytokines and factors that are produced and secreted by MSCs are visible to HSCs in the UCB, since MSCs exist in the tissue surrounding the vessels within the umbilical cord (i.e., Wharton’s Jelly). It is hypothesized that these factors produced by MSCs in the Wharton’s Jelly are available to the UCB in utero, primarily by way of diffusion from the Wharton’s Jelly to the blood. Additionally, MSCs exist in the placenta and amnion and provide for further local UCB maintenance [55].
To take full advantage of the paracrine factors secreted by MSCs and their functional effects on UCB HSCs, currently, there is great interest and effort to bank and cryopreserve native MSC populations from the Wharton’s Jelly of umbilical cord tissue. Native MSCs are primary MSCs residing within the umbilical cord, minimally procured without ex vivo manipulation and expansion. Native MSCs derived from UC tissue can be thawed and, subsequently, used to increase engraftment potential of UCB units. In fact, several groups have investigated the in vivo engraftment effects of co-transplanting MSCs from the Wharton’s Jelly of UC tissue, along with UCB, derived from both native WJ-MSCs [56] and ex vivo culture expanded WJ-MSCs [10]. In pre-clinical trials, where human umbilical cord MSCs are co-transplanted with human UCB into NOD/SCID (non-obese diabetic/severe combined immunodeficient) IL2 receptor (IL2R) gamma null mice, a dose-dependent increase in human HSC engraftment of three- to six-fold is observed [10, 56]. These results suggest that the derived, cryopreserved MSCs maintain post-processing, post-cryopreservation functional activity—for both native MSCs and ex vivo expanded umbilical cord MSCs. Notably, a greater number of transplanted ex vivo expanded Wharton’s Jelly derived MSCs were necessary to achieve the same level of engraftment observed with transplanted native WJ-MSCs. This observation suggests that even though ex vivo expansion results in an overall increase in the numbers of MSCs, it does so at the expense of MSC potency [10, 55–57].
Clinical trials have exhibited the safety and potential efficacy of MSCs in increasing hematopoietic engraftment. In one clinical trial, autologous bone marrow was procured from 28 breast cancer patients. MSCs were cultured and expanded ex vivo under GMP (good manufacturing practice) compliance under an FDA (Food & Drug Administration)-approved IND (investigational new drug) application. The autologous BM derived MSCs were infused into 28 breast cancer patients receiving MPB (mobilized peripheral blood) grafts—another source of adult HSCs—in an effort to augment hematopoietic engraftment [21]. No toxicity related to the intravenous infused expanded MSCs was observed. Furthermore, rapid hematopoietic engraftment was observed in all patients, with median neutrophil recovery (>500 μL−1) in 8 (range: 6–11) days and platelet count recovery of between 20,000 and 50,000 μL−1 unsupported in 8.5 days (range: 4–19) and 13.5 days (range: 7–44), respectively [21]. This study was not designed to determine the efficacy or mechanism of action of the infused MSCs with respect to hematopoietic engraftment. It did, however, justify further clinical studies to investigate the effect of co-transplanted MSCs on engraftment of hematopoietic grafts, such as UCB, in controlled, randomized, multicenter clinical trials. Additional clinical studies have further established the safety and efficacy of infused MSCs and in the setting of hematopoiesis, have exhibited accelerated engraftment, positive outcomes and potential treatments for graft-versus-host disease (GvHD) [27–29].
More recently, in a pilot study clinical trial, ex vivo expanded MSCs from the Wharton’s Jelly of donor umbilical cords were co-transplanted into five patients undergoing UCB transplantation. Compared with nine patients receiving cord blood transplantation alone, the five patients receiving Wharton’s Jelly MSCs, together with UCB had significantly faster engraftment of neutrophils and platelets (p = 0.02 and 0.01, respectively). The time to achieve neutrophil and platelet engraftment ranged from 7 to 13 days (median, 11 days) and 22 to 41 days (median, 32 days), respectively, for the five co-transplanted patients. The nine patients receiving cord blood alone achieved neutrophil and platelet recovery in 19–39 days (median, 25 days) and 55–113 days (median, 69 days), respectively. Although no adverse effects were observed in the co-transplanted cohort of five patients, further clinical studies need to be conducted in order to ensure safety and efficacy of Wharton’s Jelly derived MSCs [65].
Although the exact mechanism(s) of action of MSCs in accelerating hematopoietic engraftment of UCB is currently unknown, there are several postulations of the potential mode of action of MSCs in this setting. For one, MSCs may be promoting greater survival of HSCs during homing, thus, enabling quicker engraftment. With greater HSC survival, more HSCs can reach the bone marrow and, subsequently, engraft. This is the same mechanism observed in the bone marrow microenvironment and in in vitro co-culture experiments [39, 40, 47]. MSCs may also be increasing the homing capacity of UCB HSCs, directly effecting the sensitivity of the SDF-1 (stromal cell derived factor-1) homing receptors (i.e., CXCR4; C-X-C motif receptor 4), believed to be responsible for HSC homing and engraftment in the BM [66–68]. Alternatively, MSCs may be indirectly increasing homing capacity by suppressing the initial, local immune response by the host, as an initial immune response has been observed with UCB injections intravenously (IV) [45, 46]. Cells that function in innate immunity—specifically natural killer (NK) cells—quickly destroy infused UCB cells transplanted IV in NOD/SCID mice, presumably resulting from residual “leakiness” of NK cells in the animal model. However, when UCB cells are IV transplanted into NOD/SCID IL2R gamma null mice—where no residual NK cells exist, a six-fold increase in hematopoietic engraftment is observed [45, 46], demonstrating the critical role of the initial host immune response in transplanted allogeneic grafts. MSCs may suppress this initial immune response, thereby, enabling greater numbers of viable HSCs to home and engraft into the BM microenvironment [57, 63]. MSCs may also be promoting local expansion of the UCB HSCs, much in the same way that is observed in the bone marrow microenvironment. In fact, there is evidence that co-cultured MSCs with UCB HSCs can promote expansion of HSCs [39, 40, 47]. However, this ex vivo expansion results in significantly less HSC activity, when compared to un-manipulated, native HSCs possibly due to the loss of HSC survival during the ex vivo culture expansion [54–57].
2.1 Umbilical Cord Tissue MSC Banking
Due to the potential clinical applications of MSCs, in both hematopoietic and non-hematopoietic disease indications, MSCs from the umbilical cord are currently being banked, alongside UCB, for future clinical transplantations. The same native MSCs, which are trapped in the umbilical cord during fetal development, can be easily collected and harvested from the Wharton’s Jelly at delivery. Otherwise, if not collected, the cells and tissue are discarded as medical waste. This ease of collection has obvious advantages over the collection of ASCs from bone marrow and adipose tissue, for which the donor has to undergo an invasive surgical procedure [55]. This factor, coupled with the great expansion capabilities of umbilical cord derived MSCs enables WJ-MSCs to represent a virtually inexhaustible supply of MSCs for both autologous and allogeneic cellular and regenerative medicine therapies [50, 55, 57, 64].
At the time of delivery, after the collection of the donor’s UCB, the entire umbilical cord is clipped, and placed in a sterile collection jar. The separately collected UCB and umbilical cord tissue are placed in a kit and shipped to the respective cord blood bank, whereby the UCB is processed using either a validated manual or automated method to process and cryopreserve the entire collected cord blood cell population (including poly nucleated and enucleated blood cells) or an enriched population of mononuclear cells—which contains the HSC population. For the latter product, semiautomated systems such as Thermogenesis’ AXP® and BioSafe’s Sepax® system utilize density centrifugation to enrich for the buffy-coat layer (mononuclear blood cell fraction). The mononuclear blood fraction containing the HSCs is cryopreserved in the vapor phase of liquid nitrogen at temperatures below −196 °C in a two-chamber 25-mL cryopreservation bag for later therapeutic use [57].
Currently, there are two main approaches to bank MSCs from the umbilical cord tissue. One is to mechanically chop and mince the umbilical cord tissue into small pieces and cryopreserve the minced tissue for later processing. The advantage of this method for the processing laboratory is that it is relatively simple, quick, and inexpensive to process umbilical cord tissue. The disadvantage is that more processing on the back end would need to be performed—post-cryopreservation—before the cells could be used in a patient, since the MSCs remain within the tissue pieces. The time to process and collect the cells before infusion into a patient will take weeks to months, since the cells from the tissue would need to be cultured and grown in order to attain the final cell product [57].
Another method—referred to as the AuxoCell method—takes advantage of extracting the native MSCs from within the umbilical cord tissue without significantly changing the biological properties of the native cells within the tissue. This method uses mechanical mincing and/or an enzymatic digestion to “melt” away the tissue, to release the native MSCs. The liberated native MSCs can be easily collected and cryopreserved as a single cell suspension, much like UCB. The advantage of this method is the derivation of native MSCs with high potency that can be directly transplanted into the patient right away—in the same manner as UCB—without any significant manipulation. The disadvantage of this method is that it is relatively more expensive, time-consuming, and complex, than just freezing the minced tissue. The final homogenous single-cell product from the umbilical cord tissue is cryopreserved in a 25-mL cryopreservation bag, similar to the one used for the associated umbilical cord blood unit. Umbilical cord tissue MSCs are then cryopreserved at a controlled rate, and then transferred to the vapor phase of nitrogen for long-term storage, once the units have passed all quality controls and are found to be free of pathogens and contaminants [57].
Although both methods render MSC products, these cell products are quite different. The final transplanted product derived from the cryopreserved minced tissue is biologically different than the native MSCs collected prior to cryopreservation. Since tissue, and not cells, are cryopreserved in the former method, when needed for transplantation, the product would need to be derived by growing cells out of the tissue for a period of several weeks to months in an artificial environment outside the body (e.g, incubator). This manipulation significantly reduces the potency of the derived ex vivo expanded cells relative to their native state. To that end, although theoretically practical to extract native MSCs from frozen minced umbilical cord tissue, in reality, the efficiency in MSC recovery post thaw is approximately 10 % to that of native MSC extraction from fresh (non-cryopreserved) umbilical cord tissue [3]. Taghizadeh RR, Mrowiec ZR, Cetrulo KJ, Cetrulo CL. Unpublished data. 2010.
UCB processing is performed such that the innate, native mononuclear cell population, for instance, is extracted from the whole UCB in a timeframe that would not alter the biological properties of the cells and without any significant ex vivo manipulation (e.g., ex vivo expansion). This same framework can be used to extract native MSCs from the umbilical cord tissue after collection of the UCB, without changing the native biological properties of the derived MSC population. Once the extracted MSCs are collected and cryopreserved, they represent the native MSCs that were originally found in the Wharton’s Jelly between day 4 and 12 of embryonic development [56, 61]. Once the native MSCs are placed in in vitro culture flasks or bioreactors, the resultant cell products have biologically changed—in that they no longer represent the same parent population, but are, rather, cell progenies procured under artificial biological conditions (i.e., incubators, plastic-coated flask, bioreactors, medium) [57].
Although, it is the aim of ex vivo culture expansion to maintain the native properties of the derived MSCs, this is not actually the case. MSCs are classically defined as cells that adhere to coated plastic surfaces, express MSC markers such as CD90, CD73, CD105, CD44, CD29, lack expression of hematopoietic markers CD45, CD14, CD34, and have the capacity for multilineage differentiation into osteocytes, chondrocytes, and adipocytes [9]. The challenge with this artificial definition is that it defines MSCs prospectively, rather than defining the properties of the actual native population of MSCs in vivo. The native MSCs in adipose tissue, dental pulp, umbilical cord tissue, or bone marrow, do not adhere to plastic in vivo, although as soon as native MSCs are derived and placed into culture-treated plastic flasks, they selectively adhere to the coated plastic and expand. Culture expansion of MSCs results in loss of MSC potency, as native MSC activity is gradually lost (and not replenished) and found to lead to accelerated aging and senescence in vitro [5, 10, 13, 14, 56]. Culture expanded Wharton’s Jelly derived MSCs exhibit lower rates of hematopoietic engraftment compared to un-manipulated, native WJ-MSCs—when co-transplanted at the same UCB and WJ-MSC dose [10, 55–57]. Therefore, for these reasons, methods have been developed to collect native HSCs and MSCs from the UCB and tissue, respectively, as these native cell populations possess the most potent stem cell activity. This way the full therapeutic benefit of each respective stem cell population can be harnessed without the risk of changing the innate biological and/or genetic properties of each stem cell population.
2.1.1 Graft-Versus-Host Disease
Additional properties of MSCs make them useful stem cell candidates for use in various regenerative medicine therapies, beyond UCB hematopoietic engraftment. Recent evidence suggests that WJ-MSCs have the potential to be used to treat GvHD. GvHD, a disease where the immune cells of a transplanted graft initiate an immunological response to the host tissue, causes debilitating illness in patients who have previously received a bone marrow transplant. The complications include skin rashes and abdominal symptoms with diarrhea and liver problems. Bone marrow MSCs have been the most extensively studied for treatment of GvHD. Osiris Therapeutics Inc., has utilized the immunosuppressive ability of MSCs in a multicenter, randomized, and controlled placebo studies for GvHD [18]. In this pivotal phase 3 clinical study, patients with grade II–IV GvHD were randomized to receive two treatments of an off-the-shelf allogeneic BM derived MSC product, Prochymal®, along with corticosteroids. Although 94 % of patients had an initial response to Prochymal®, the trial failed, however, due to not meeting the trials primary clinical endpoint. Prochymal® did not significantly increase complete remission of steroid refractory GvHD lasting 28 days, when compared to placebo controls [11, 35]. Interestingly, in a similar phase 2 clinical trial study, patients with severe GvHD were treated with BM-MSCs derived using an ex vivo expansion procedure based on the European Group of Blood and Marrow Transplantation. The results of this study demonstrated that nearly half of patients with steroid-refractory, acute GvHD, treated with BM-MSC responded to the treatment, irrespective of HLA-matching to donor MSCs. At 2 years posttreatment, nearly half of the patients with a complete response to the BM-MSC treatment were alive [28]. Although these two specific studies had different clinical endpoints, it is clear that the age of the donor, as well as the ex vivo culture method and length, are important variables that need to be better understood and controlled for future clinical studies for GvHD and other diseases.
Since donor age and ex vivo manipulation are significant variables for BM derived MSCs, a possible source that may dampen the effects of these variables are MSCs derived from the Wharton’s Jelly. WJ-MSCs have yet to be tested in the clinic for GvHD, but since these MSCs are derived from the earliest ethically feasible point in human development, they may have reduced donor-to-donor associated variability. Furthermore, since WJ-MSCs have relatively longer telomeres, faster doubling times, greater pluripotency, and take longer to reach senescence, WJ-MSCs may be a more suitable starting MSC source for GvHD treatment [10, 59].
Both hematopoietic and MSCs maintain great therapeutic potential. HSCs, especially those derived from perinatal sources, such as UCB, are currently being investigated in clinical trials in the treatment of numerous hematopoietic and non-hematopoietic diseases. Much effort has been placed in showing the safety and efficacy of MSCs in the clinic, especially those derived from bone marrow and adipose tissue. As the field moves forward, additional clinical studies will investigate the safety and efficacy of MSCs derived from the Wharton’s Jelly of the umbilical cord tissue, in not only the setting of accelerating hematopoietic engraftment with UCB but also in regulating tissue repair/renewal, immunosuppression, and mesodermal tissue formation in the treatment of various diseases from GvHD to wound healing to even sports-related injuries.
3 Regenerative Medicine and Tissue Engineering Applications of Umbilical Cord Derived Mesenchymal Stem Cells
Although MSCs have been studied extensively from basic research to the clinic, for uses such as the augmentation of HSC engraftment and the treatment of GvHD, MSCs have been examined most extensively for their utility in tissue engineering and regenerative medicine. This section will extensively review the use of MSCs in regenerative medicine application. Concise reviews of their applicability for wound healing, functional hepatocyte development, lung repair, musculoskeletal tissue engineering applications, to include tendon and cartilage, as well as delivery options currently being pursued in surgical suites will be discussed. Although the presented publications may utilize MSCs from adult sources, they do provide proof-of-concept for use with perinatal sources of MSCs, namely those derived from the Wharton’s Jelly of the umbilical cord. Although further studies need to be conducted, it is conceivable that the use of perinatal MSCs from cord tissue, in lieu of adult BM-MSCs, may result in greater regenerative capabilities and, therefore, superior clinical outcomes, mainly due to the inherent relative infancy of perinatal MSCs.
Although MSCs have been derived from diverse tissue sources, the umbilical cord is a readily accessible source for procuring MSCs. For many reasons, umbilical cord MSCs represent a promising agent in the field of regenerative and transplant medicine. Obtained from the Wharton’s Jelly region of umbilical cords, these Wharton’s Jelly MSCs hold specific potential in regenerative medicine, serving as differentiating cells shown to grow on synthetic scaffolds and compliant cultures. In addition to their advantages of improved accessibility and reduced ethical concerns, WJ-MSCs have also been shown to be non-immunogenic and possess potent immunosuppressive effects. Studies examining immune-related genes have indicated that WJ-MSCs express numerous immune tolerance genes while, concurrently, not expressing the deleterious immune response genes.
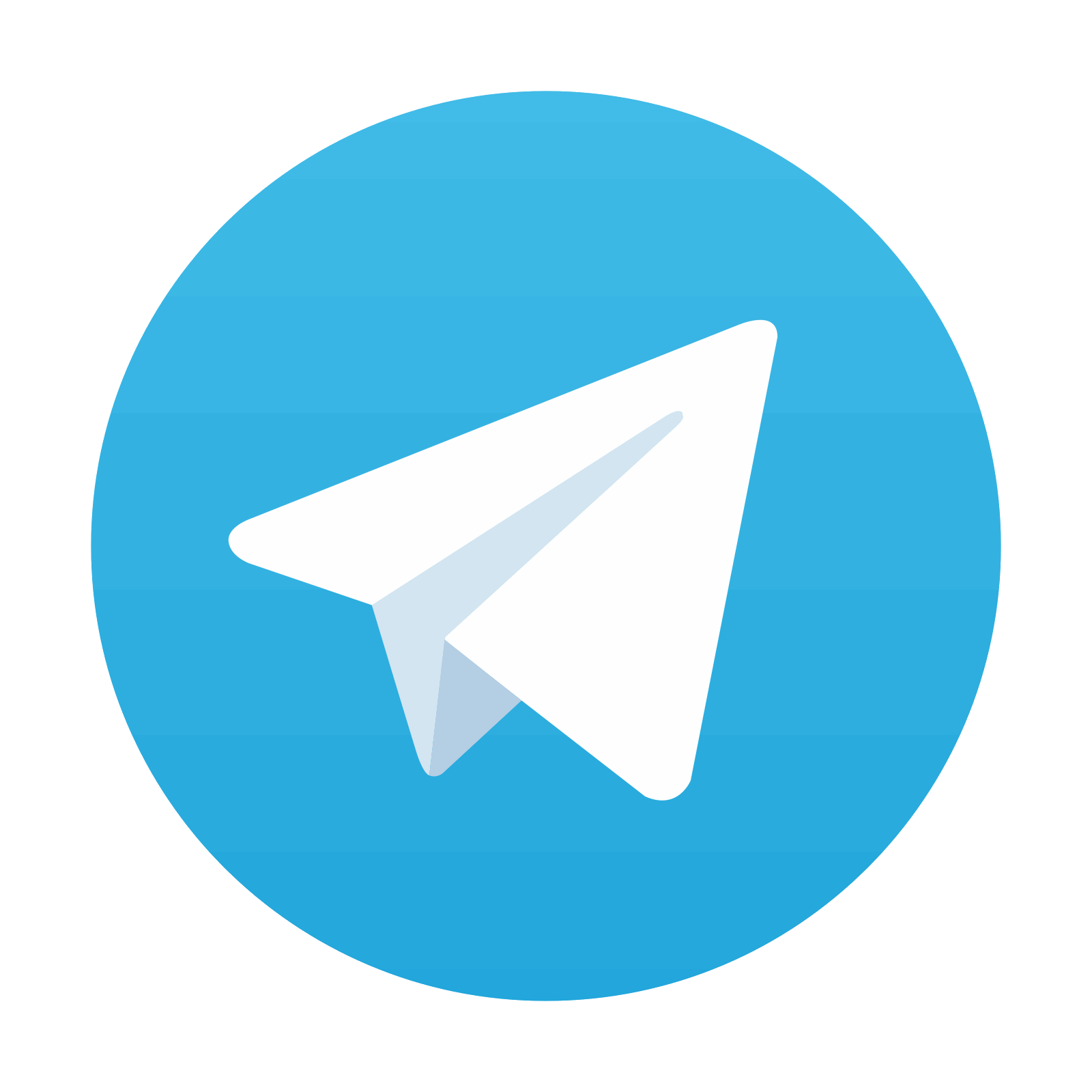
Stay updated, free articles. Join our Telegram channel

Full access? Get Clinical Tree
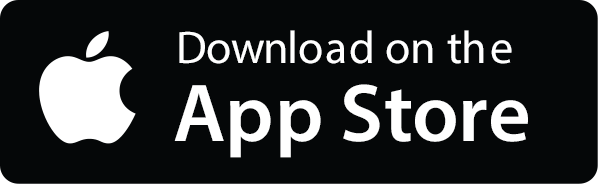
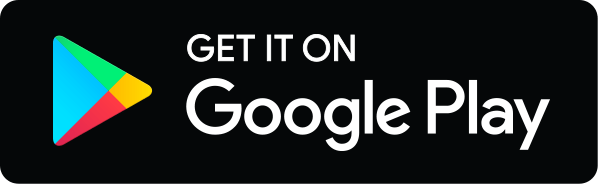