43
Tumor Viruses
CHAPTER CONTENTS
INTRODUCTION
Viruses can cause benign or malignant tumors in many species of animals (e.g., frogs, fishes, birds, and mammals). Despite the common occurrence of tumor viruses in animals, only a few viruses are associated with human tumors, and evidence that they are truly the causative agents exists for very few.
Tumor viruses have no characteristic size, shape, or chemical composition. Some are large, and some are small; some are enveloped, and others are naked (i.e., nonenveloped); some have DNA as their genetic material, and others have RNA. The factor that unites all of them is their common ability to cause tumors.
Tumor viruses are at the forefront of cancer research for two main reasons:
(1) They are more rapid, reliable, and efficient tumor producers than either chemicals or radiation. For example, many of these viruses can cause tumors in all susceptible animals in 1 or 2 weeks and can produce malignant transformation in cultured cells in just a few days.
(2) They have a small number of genes compared with a human cell (only three, four, or five for many retroviruses), and hence their role in the production of cancer can be readily analyzed and understood. To date, the genomes of many tumor viruses have been cloned and sequenced and the number of genes and their functions have been determined; all of this has provided important information.
MALIGNANT TRANSFORMATION OF CELLS
The term malignant transformation refers to changes in the growth properties, shape, and other features of the tumor cell (Table 43–1). Malignant transformation can be induced by tumor viruses not only in animals but also in cultured cells. In culture, the following changes occur when cells become malignantly transformed.
Altered Morphology
Malignant cells lose their characteristic differentiated shape and appear rounded and more refractile when seen in a microscope. The rounding is due to the disaggregation of actin filaments, and the reduced adherence of the cell to the surface of the culture dish is the result of changes in the surface charge of the cell.
Altered Growth Control
(1) Malignant cells grow in a disorganized, piled-up pattern in contrast to normal cells, which have an organized, flat appearance. The term applied to this change in growth pattern in malignant cells is loss of contact inhibition. Contact inhibition is a property of normal cells that refers to their ability to stop their growth and movement upon contact with another cell. Malignant cells have lost this ability and consequently move on top of one another, continue to grow to large numbers, and form a random array of cells.
(2) Malignant cells are able to grow in vitro at a much lower concentration of serum than are normal cells.
(3) Malignant cells grow well in suspension, whereas normal cells grow well only when they are attached to a surface (e.g., a culture dish).
(4) Malignant cells are easily cloned (i.e., they can grow into a colony of cells starting with a single cell), whereas normal cells cannot do this effectively.
(5) Infection of a cell by a tumor virus “immortalizes” that cell by enabling it to continue growing long past the time when its normal counterpart would have died. Normal cells in culture have a lifetime of about 50 generations, but malignantly transformed cells grow indefinitely.
Altered Cellular Properties
(1) DNA synthesis is induced. If cells resting in the G1 phase are infected with a tumor virus, they will promptly enter the S phase (i.e., synthesize DNA and go on to divide).
(2) The karyotype becomes altered (i.e., there are changes in the number and shape of the chromosomes as a result of deletions, duplications, and translocations).
(3) Antigens different from those in normal cells appear. These new antigens can be either virus-encoded proteins, preexisting cellular proteins that have been modified, or previously repressed cellular proteins that are now being synthesized. Some new antigens are on the cell surface and elicit either circulating antibodies or a cell-mediated response that can kill the tumor cell. These new antigens are the recognition sites for immune surveillance against tumor cells.
(4) Agglutination by lectins is enhanced. Lectins are plant glycoproteins that bind specifically to certain sugars on the cell membrane surface (e.g., wheat germ agglutinin). The increased agglutination of malignant cells may be due to the clustering of existing receptor sites rather than to the synthesis of new ones.
Altered Biochemical Properties
(1) Levels of cyclic adenosine monophosphate (AMP) are reduced in malignant cells. Addition of cyclic AMP will cause malignant cells to revert to the appearance and growth properties of normal cells.
(2) Malignant cells secrete more plasminogen activator than do normal cells. This activator is a protease that converts plasminogen to plasmin, the enzyme that dissolves the fibrin clot.
(3) Increased anaerobic glycolysis leads to increased lactic acid production (Warburg effect). The mechanism for this change is unknown.
(4) There is a loss of high-molecular-weight glycoprotein called fibronectin. The effect of this loss is unknown.
(5) There are changes in the sugar components of glycoproteins and glycolipids in the membranes of malignant cells.
ROLE OF TUMOR VIRUSES IN MALIGNANT TRANSFORMATION
Malignant transformation is a permanent change in the behavior of the cell. Must the viral genetic material be present and functioning at all times, or can it alter some cell component and not be required subsequently? The answer to this question was obtained by using a temperature-sensitive mutant of Rous sarcoma virus. This mutant has an altered transforming gene that is functional at the low, permissive temperature (35°C) but not at the high, restrictive temperature (39°C). When chicken cells were infected at 35°C they transformed as expected, but when incubated at 39°C, they regained their normal morphology and behavior within a few hours. Days or weeks later, when these cells were returned to 35°C, they recovered their transformed phenotype. Thus continued production of some functional virus-encoded protein is required for the maintenance of the transformed state.
Although malignant transformation is a permanent change, revertants to normality do appear, albeit rarely. In the revertants studied, the viral genetic material remains integrated in cellular DNA, but changes in the quality and quantity of the virus-specific RNA occur.
PROVIRUSES & ONCOGENES
The two major concepts of the way viral tumorigenesis occurs are expressed in the terms provirus and oncogene. These contrasting ideas address the fundamental question of the source of the genes for malignancy.
(1) In the provirus model, the genes enter the cell at the time of infection carried by the tumor virus.
(2) In the oncogene model, the genes for malignancy are already present in all cells of the body by virtue of being present in the initial sperm and egg. These oncogenes encode proteins that encourage cell growth (e.g., fibroblast growth factor). In the oncogene model, carcinogens such as chemicals, radiation, and tumor viruses activate cellular oncogenes to overproduce these growth factors. This initiates inappropriate cell growth and malignant transformation (Figure 43–1).
FIGURE 43–1 Effect of cellular oncogenes and tumor suppressor genes on the cell cycle. The oncoproteins encoded by cellular oncogenes activate the cell cycle by allowing passage from the G1 phase into the S phase. The proteins encoded by tumor suppressor genes, notably p53 and RB, inhibit the cell cycle in the G1 phase. Inactivation of these proteins activates the cell cycle by allowing passage from the G1 phase into the S phase. G1, gap 1; G2, gap 2; M, mitosis; S, synthesis of DNA. (Modified with permission from Murray RK et al. Harper’s Illustrated Biochemistry. 29th ed. New York: McGraw-Hill, 2012.)
Both proviruses and oncogenes may play a role in malignant transformation. Evidence for the provirus mode consists of finding copies of viral DNA integrated into cell DNA only in cells that have been infected with the tumor virus. The corresponding uninfected cells have no copies of the viral DNA.
1. Role of Cellular Oncogenes in Tumorigenesis
The first direct evidence that oncogenes exist in normal cells was based on results of experiments in which a DNA copy of the onc gene of the chicken retrovirus Rous sarcoma virus was used as a probe. DNA in normal chicken embryonic cells hybridized to the probe, indicating that the cells contain a gene homologous to the viral gene. It is hypothesized that the cellular oncogenes may be the precursors of viral oncogenes. Proto-oncogenes are the normal precursors of the cellular oncogenes. Proto-oncogenes encode normal cellular proteins and are under regulatory control. Cellular oncogenes have acquired mutations that cause them to escape regulatory control and overproduce altered proteins. Figure 43–2 shows the functions of important oncoproteins encoded by the cellular oncogenes.
FIGURE 43–2 Functions of cellular oncoproteins. Cellular oncogenes encode proteins with a variety of functions that are shown in the figure. These oncoproteins activate the cell cycle and cause cell to grow in an unregulated manner. Used with permission from Murray RK et al. Harper’s Illustrated Biochemistry. 29th ed. New York: McGraw-Hill, 2012.)
Although cellular oncogenes and viral oncogenes are similar, they are not identical. They differ in base sequence at various points; and cellular oncogenes have exons and introns, whereas viral oncogenes do not. It seems likely that viral oncogenes were acquired by incorporation of cellular oncogenes into retroviruses lacking these genes. Retroviruses can be thought of as transducing agents, carrying oncogenes from one cell to another. (See Chapter 4 for a discussion of transduction).
Since this initial observation, more than 20 cellular oncogenes have been identified by using either the Rous sarcoma virus DNA probe or probes made from other viral oncogenes. Table 43–2 describes the function of several important cellular oncogenes and their relationship to various human cancers. Many cells contain several different cellular oncogenes. In addition, the same cellular oncogenes have been found in species as diverse as fruit flies, rodents, and humans. Such conservation through evolution suggests a normal physiologic function for these genes. Some are known to be expressed during normal embryonic development.
A marked diversity of viral oncogene function has been found. Some, such as the src gene, encode a protein kinase that specifically phosphorylates the amino acid tyrosine, in contrast to the commonly found protein kinase of cells, which preferentially phosphorylates serine.
Other oncogenes have a base sequence almost identical to that of the gene for certain cellular growth factors (e.g., epidermal growth factor). Several proteins encoded by oncogenes have their effect at the cell membrane (e.g., the ras oncogene encodes a G protein), whereas some act in the nucleus by binding to DNA (e.g., the myc oncogene encodes a transcription factor). These observations suggest that growth control is a multistep process and that carcinogenesis can be induced by affecting one or more of several steps.
On the basis of the known categories of oncogenes, the following model of growth control can be constructed. After a growth factor binds to its receptor on the cell membrane, membrane-associated G proteins and tyrosine kinases are activated. These, in turn, interact with cytoplasmic proteins or produce second messengers, which are transported to the nucleus and interact with nuclear factors. DNA synthesis is activated, and cell division occurs. Overproduction or inappropriate expression of any of the preceding factors in boldface type can result in malignant transformation.
Note that not all tumor viruses of the retrovirus family contain onc genes. How do these viruses cause malignant transformation? It appears that the DNA copy of the viral RNA integrates near a cellular oncogene, causing a marked increase in its expression. This process is called insertional mutagenesis. Overexpression of the cellular oncogene may play a key role in malignant transformation by these viruses.
Although it has been demonstrated that viral oncogenes can cause malignant transformation, it has not been directly shown that cellular oncogenes can do so. However, as described in Table 43–3, the following evidence suggests that they do:
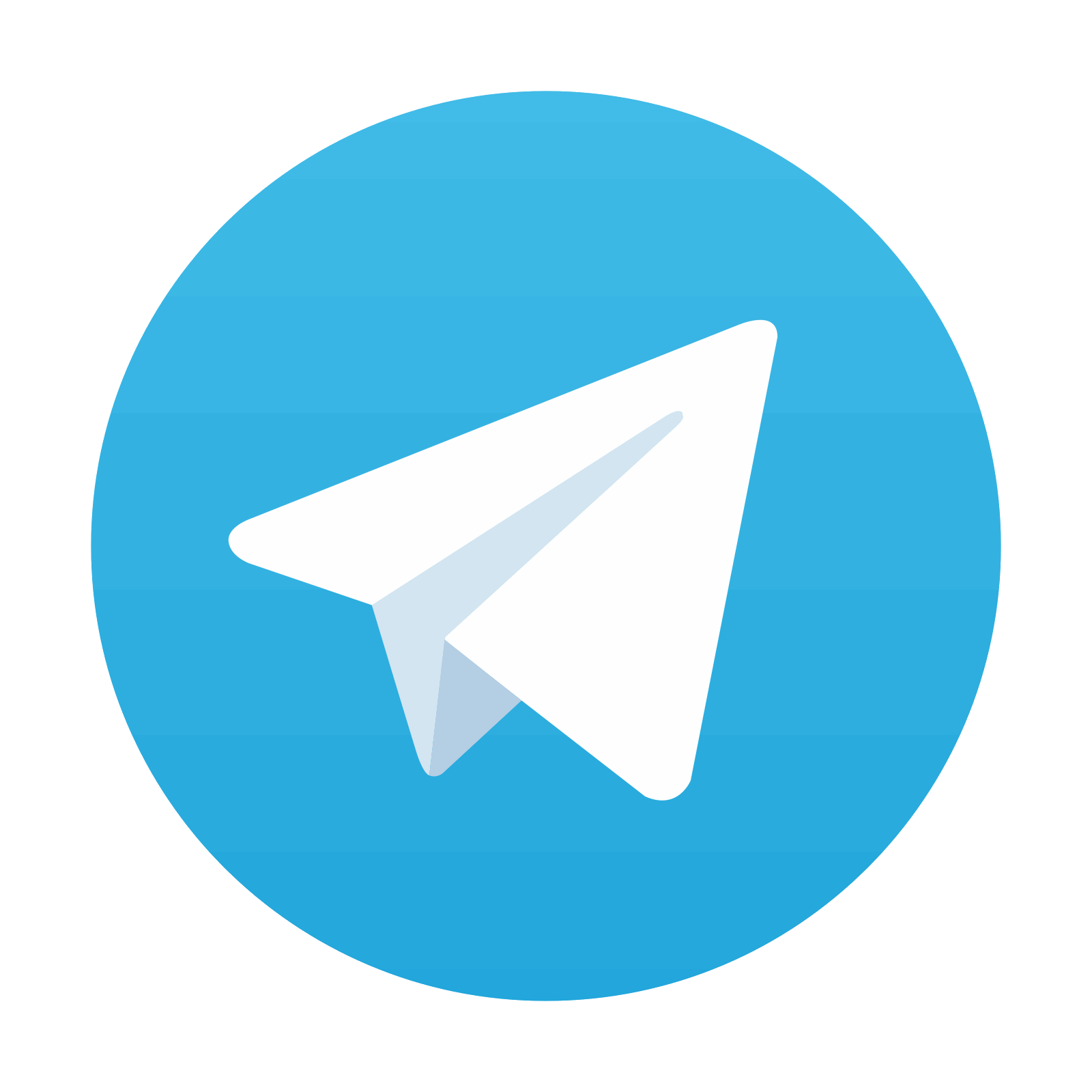
Stay updated, free articles. Join our Telegram channel

Full access? Get Clinical Tree
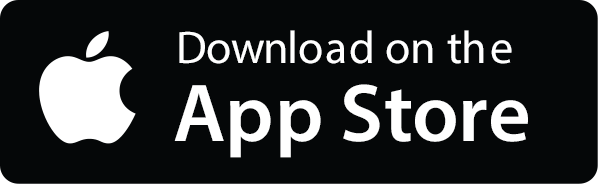
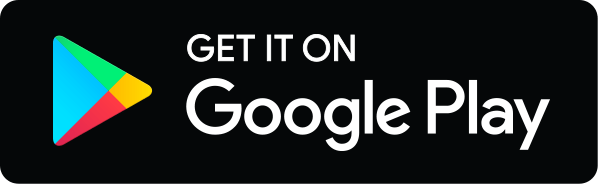