Figure 19-1. Ten leading causes of death by age group in the United States (2014). Source: Centers for Disease Control and Prevention.
Figure 19-2. Ten leading causes of death by age group in the United States (2014), highlighting unintentional causes. Source: Centers for Disease Control and Prevention.
Table 19-1 Death Rates by Mechanisms (United States 2013)a
6 Despite obvious differences in the mechanisms of injuries, hemorrhage and TBI remain the leading causes of death not only in civilian but also in combat trauma,28 and effective treatment strategies have the potential to save many lives in the battlefield.29 Traditionally, management of a bleeding patient focuses on the replacement of lost blood, along with standard cardiopulmonary resuscitative strategies. The type of fluid used for resuscitation has evolved since it was introduced nearly a century ago. Toward the end of World War I and during World War II, transfusion of whole blood was the primary approach in the treatment of military traumatic hemorrhage. However, after World War II, the separation of whole blood into its components became widely accepted and replaced the whole-blood transfusion for logistical and financial reasons. During the Vietnam War era, use of salt-based solutions (crystalloids) became popular. Over the next 3 decades we saw a major rise in the volume of salt-water administration in trauma patients. Although it is cheap, easy to store and administer, from the very start there were concerns about the suitability of crystalloid fluids as a substitute for the lost blood. Blood is a complex fluid that contains thousands of proteins, cells that carry oxygen to the tissues (red blood cells) and generate clot to stop bleeding (red blood cells and platelets), various clotting factors, buffers, enzymes, and hormones. As crystalloids lack all of these, their administration results in rapid dilution of cells, clotting factors, and proteins, which can worsen bleeding. Thus, aggressive crystalloid resuscitation, especially in the absence of early hemorrhage control, is impractical, inadequate, and potentially harmful in many circumstances.30–33 Not only it lacks any specific pro-survival properties, large volume crystalloid infusion can exacerbate bleeding and worsens cellular injury.34 It should be emphasized that survival in these patients requires definitive control of bleeding, and most deaths today are caused by delays in controlling the source of bleeding, and not due to inadequate delivery of resuscitation fluids. These challenges are compounded in austere circumstances such as a battlefield, where 87% of deaths occur before patients reach a medical facility,28 yet nearly a quarter of these injuries are considered potentially survivable (PS). Not surprisingly, the PS category is largely (91%) made up of hemorrhage-related deaths, with most being truncal (67%).28 In the injured that live long enough to reach a medical facility, the percentage of PS deaths increases to 51%, with hemorrhage accounting for 80%.29 Thus, novel therapies that could improve hemorrhage control, and keep the injured alive long enough to get to higher echelons of care, have the potential to make the biggest difference.
Figure 19-3. Motor vehicle-related fatalities over time in the United States. From US Department of Transportation. NHTSA. 2014 Motor Vehicle Crashes: Overview. http://www-nrd.nhtsa.dot.gov/Pubs/812246.pdf.
Figure 19-4. Age and sex distribution of the United Sates population: 2010, 2030, and 2050. From Vincent GK, Velkoff VA. The next four decades, the older population in the United States: 2010 to 2050. Current Population Reports, pages 25–1138. Washington, DC: US Census Bureau; 2010.
Figure 19-5. Death rates from unintentional falls. The figure is a line chart showing that during 2000–2013, age-adjusted death rates from unintentional falls increased steadily for both men and women aged ≥65 years, with consistently higher rates observed among men. During this period, death rates from falls increased from 38.2 per 100,000 population in 2000 to 67.9 in 2013 among men and from 24.6 to 49.1 among women. From National Vital Statistics System mortality data. Available at http://www.cdc.gov/nchs/deaths.htm.
Figure 19-6. Death Rates for Three Selected Causes of Injury – National Vital Statistics System, United States, 1979–2012. In 2012, a total of 41,502 drug poisoning deaths, 34,935 motor vehicle traffic deaths, and 33,563 firearm deaths occurred. The age-adjusted death rate for drug poisoning more than quadrupled from 3 per 100,000 in 1979 to 13.1 in 2012. In contrast, the age-adjusted rate dropped from 22.1 to 10.9 for motor vehicle traffic deaths and from 14.7 to 10.5 for firearm deaths during this period. The age-adjusted drug poisoning death rate exceeded the motor vehicle traffic death rate beginning in 2009. From CDC WONDER, compressed mortality file, underlying cause-of-death, available at http://wonder.cdc.gov/mortsql.html.
BIOMECHANICS OF INJURY
It is important to realize that at its most basic level injuries result from deformation of tissues that results in damage. In general, injuries can be categorized as penetrating (sharp objects or firearms) or blunt. There are clearly differences between these mechanisms that must be kept in mind while evaluating the patient. Penetrating trauma causes injury to the objects that are in the path of the foreign body, whereas blunt trauma causes damage either through crush or shear forces. The diagnostic and management approach is therefore very different.
Blunt trauma is best understood by applying the rules of kinetic energy transfer: KE = (M × V)/2, where KE is kinetic energy, M is mass of the object, and V is velocity. Thus, a head on collision between two large sport utility vehicles (∼6,000 lb each) traveling at 65 mph can generate enormous amounts of forces. Various safety mechanisms in the modern vehicles direct this force away from the occupants of the vehicle, but the clinicians have a tendency to underappreciate the forces and the vectors involved in the collision. With frontal impact, the vehicle stops abruptly and the occupants continue to move forward (if not belted) and either hit the steering wheel or slide under to hit their knees against the dashboard resulting in classic injury patterns (Fig. 19-7). In a rear impact, the kinetic injury generated depends upon the differences between the velocities of the two vehicles, and the occupant of the front vehicle abruptly accelerates forward resulting in cervical spine hyperextension (“whiplash”). Lateral impact imparts rotational forces and often results in significant protrusion of the passenger compartment. In addition to limb trauma, this can result in major torso (chest, abdomen, and pelvis) injuries. The rotational forces are also more likely to cause torsion injury where the mobile areas join relatively fixed structures (e.g., aortic laceration, cervical spinal ligamentous injuries). Rollover crashes are especially dangerous as the vehicle is impacted from various angles repeatedly during the process. The occupants of the vehicle are thrown around and may even be ejected from the vehicle. Ejection increases the chances of death by ∼10 fold. Similarly, death of another occupant is considered a high-risk event, as it is a surrogate for the severity of impact or the complexity of the forces involved. The clinician also must specifically look for clinical evidence of energy transfer. For example, a “seat belt” sign across the abdomen must raise suspicion for energy transfer across the bowel, mesentery, pancreas, and the lumbar spine. Similarly, wedge fracture of L1 vertebra in this setting mandates ruling out a pancreatic injury as the pancreatic body drapes across the anterior aspect of L1. In fact, orthopedic injuries should be considered markers of kinetic energy transfer and make the clinicians proactively look for injuries to adjacent organs, nerves, vessels, and tendons (Table 19-2).
Penetrating trauma should broadly be considered in two categories based upon the mechanism. Sharp objects can cause lacerations or puncture wounds. Lacerations are relatively more straightforward as the structures in the depth of the wound can be examined and injuries to critical organs ruled out by careful inspection. Puncture wounds (or stabs), on the other hand, are challenging when it comes to ruling out occult injury to deeper structures. For example, stab wound to the neck can cause injuries to the airway, esophagus, blood vessels, thyroid gland, or intrathoracic organs. Similarly, stab wound to the abdomen, chest, flank, and so forth must be evaluated for potential intracavity vascular, solid organ or hollow viscus injuries. Historically, most of these patients used to get surgical explorations but now with better imaging techniques, carefully selected stable patients are often evaluated with advanced imaging studies and/or endoscopy, and managed nonoperatively.35–39 Clearly, there has been a recent increase in the use of computerized tomography (CT) scans in the evaluation of trauma patients,40 and careful use of CT scan can reveal additional findings that may not be otherwise apparent.41 This, however, does not apply to stab wounds to the abdomen, where CT scan has been shown to add no value to the serial physical examinations and close observation.42 In this prospective study of 249 patients with stab wounds to the abdomen, the CT scan findings did not alter the clinical decision making. Forty-five patients (18.1%) underwent immediate laparotomy, 27 (10.8%) had superficial injuries allowing immediate discharge, and the remaining 177 (71.1%) underwent CT. Of these, 154 (87.0%) were successfully observed, with 20 (11.3%) requiring laparotomy, 2 (1.1%) thoracotomy, and 1 (0.6%) sternotomy. Of the 20 laparotomies, 16 (80.0%) were therapeutic. All patients who underwent therapeutic laparotomy did so based on their physical examination alone. The sensitivity and specificity of physical examination were 100% and 98.7%, respectively, while those of CT were 31.3% and 84.2%, respectively. Thus, even in this era of ubiquitous radiographic imaging, the burden is on the clinician to carefully evaluate the patient and make a clinical decision in the trauma bay. One reason CT scans can be misleading in stab wounds is because unlike the gunshot wounds where the track is easier to appreciate due to surrounding tissue injury and cavitation effect, the depth of stab wounds is notoriously difficult to determine on the CT scan. Often, the tissues come back together when the knife is withdrawn, making it difficult to determine the precise depth of penetration (often the knife has penetrated much deeper than the track that is visible on the scan). Thus, in stable patients, nonoperative management of penetrating abdominal trauma is not unreasonable, even if they have solid organ injuries,43 but it is not a simple radiographic decision. In fact, it remains a clinical decision that should be made by an experienced trauma surgeon. These patients also require close observation, serial examinations, and monitoring of laboratory parameters for the next 12 to 24 hours. In settings where this is not possible (e.g., lack of 24/7 clinician availability), the safer option would be to explore the injured body cavity (either laparoscopic or open). Bullet wounds differ from stab wounds not only in the fact that the bullets impart significant kinetic energy depending upon their velocity and weight (Table 19-3) but can also cause “cavitation” effect. Cavitation occurs as tissues impacted by the bullet recoil and transmit the kinetic energy outward, creating a cavity due to the rapid acceleration and deceleration. This can injure organs/tissues that are adjacent to the bullet track even if not hit directly by the projectile. In addition to the kinetic energy, the actual area of injury is influenced by factors such as profile of the bullet, tumble (spin and yaw), and fragmentation. A jacketed bullet does not deform much whereas a hollow point bullet fragments, spreads, and deforms (increasing the area of damage). Similarly, tumbling of the bullet increases the area of energy exchange and much larger zone of damage. Generally, low-velocity (<1,200 ft/s) or medium velocity (1,200 to 2,000 ft/s) firearms are more common in urban trauma, with high-velocity wounds seen typically in combat settings. The cavitation effect is significantly more pronounced in high-velocity injuries, and the kinetic energy differences translate into a much larger area of destruction, more devitalized tissues, and contamination (cavitation can pull in debris, clothes etc. into the wound). Injuries to multiple organs and structures, even in different body cavities, is possible with bullet wounds. In the operating room, it is important to set clear priorities, and to approach the injuries in a logical fashion. The first priority should be to control the hemorrhage quickly (clamps, catheters, packing, etc.), followed by a quick assessment to identify and treat other life-threatening injuries (pneumothorax, cardiac tamponade, etc.). This should be followed by control of contamination. Then the physiologic status of the patient should be assessed to determine whether definitive repair of all the injuries is feasible versus a “damage control approach” (Table 19-4) where only an abbreviated operation is done and patient brought back to the operating room later for additional interventions (after full resuscitation). While looking for injured organs in penetrating trauma, topography for anatomic proximity must be kept in mind. For example, penetrating injury to the carotid mandates that we exclude injury to adjacent organs such as jugular, trachea, esophagus, and the spine. Similarly, one hole in the bowel should prompt us to look for another hole (through and through injury) that may not be obvious, as well as examining other adjacent organs. For example, a bullet injury to the anterior wall of the stomach can easily pass through the posterior wall, through the pancreas, duodenum, vena cava, and the kidney, depending upon the trajectory. While trying to determine the trajectory, it is important to not assume that the subject was in a “normal” anatomical position (standing straight front facing with arms by his side) when shot. Typically, victims assume a variety of odd positions in an effort to avoid getting hit. In addition, bullets do not necessarily travel in a linear track and can bend and bounce off in atypical directions. Thus, gunshot wound to the chest can easily result in injuries not only in the chest (ipsilateral or contralateral), but also in the abdomen, pelvis, or the neck depending upon the course of the projectile. It is also wrong to notice two holes and assume that they represent a through and through gunshot wound. It is equally likely to be two separate gunshot wounds with both bullets still inside the body. As a general rule, the number of gunshot wounds and the number of retained bullets should add up to an even number; otherwise, you are either missing a hole or a bullet. Even in critically ill patients, quick x-rays of the chest/abdomen/pelvis, as a “bullet survey” can be very helpful before rushing to the operating room. This information can be critically important in planning the surgical approach, and in deciding which body cavity to tackle in what sequence.
Figure 19-7. A, B: Frontal deceleration impact.
Table 19-2 Patterns of Injury to the Head, Neck, Trunk, and Extremities Associated with Orthopedic Injuries
Table 19-3 Muzzle Velocity, Kinetic Weight of Projectile, and Approximate Maximum Kinetic Energy of Frequently Used Firearms
Table 19-4 List of Proposed Indications for Damage Control, Including Range of Values Reported in Various Articles
FIELD TRIAGE DECISIONS AND EARLY PRIORITIES
Broadly speaking, the trauma patients can be divided into three categories: patients with (a) rapidly fatal injuries, (b) potentially fatal injuries, and (3) all others. A key component of effective prehospital triage is to properly place the injured into these categories based on the available data (mechanism of injury, age of the patient, co-morbid diseases, physiologic status, and injury patters), and then make a rapid decision about what type of facility is the most appropriate for the patient. Committee on Trauma for the American College of Surgeons (ACS-COT) has developed detailed criteria for designating hospitals into different levels depending upon the resource availability, from the highest (level I) to the lowest (level IV). These criteria can easily be found in the Resources for Optimal Care of the Injured Patient – 2014.44 Working with the Centers for Disease Control and Prevention, ACS-COT has also developed a guideline for the prehospital triage of the injured patients (Algorithm 19-1).45 The document also contains very detailed guidelines about which patients should be transferred from lower-level to higher-level trauma centers (Table 19-5).44 The key priorities during the prehospital phase are the ABCs of trauma care (airway, breathing, and circulation), with a major focus on hemorrhage control (direct compression, advanced hemostatic dressings, and tourniquets etc.). In patients that are in hemorrhagic shock but are breathing and maintaining adequate airway/oxygenation, intubation and mechanical ventilation should be avoided (especially for short prehospital times) as administration of sedating drugs and an increase in the intrathoracic pressure (due to positive pressure ventilation) further decreases the preload, which could precipitate an arrest. Judicial administration of crystalloid fluids is acceptable, but aggressive fluid resuscitation in the absence of hemorrhage control can worsen the outcomes. The proof of this concept in humans was published in the New England Journal of Medicine in 1994.33 In that study, hypotensive patients with penetrating injury to the torso were randomized to routine fluid resuscitation, or resuscitation was delayed until bleeding had been surgically controlled. The results showed a survival advantage in the delayed resuscitation group (70% vs. 62%, p = 0.04). In fact, a systematic analysis of all the published data failed to find any support in favor of (or against) large volume crystalloid resuscitation in bleeding trauma patients.46 As these studies had focused on young patients with penetrating trauma, the question remains whether these findings can be generalized to the blunt trauma population. The blunt trauma patients are typically older, may have a higher incidence of coronary and carotid stenosis, and about 20% to 30% have associated TBI (which can worsen due to hypotension). Furthermore, as opposed to penetrating trauma, the sources of bleeding can be multiple and not always surgically fixable in the blunt trauma victims. Because of all these reasons, limited volume (“controlled”) resuscitation rather than no resuscitation is logical in this population. This is also supported by an early pilot prospective randomized trial where 192 patients with an out-of-hospital systolic blood pressure (SBP) of 90 mm Hg or lower were randomized to receive controlled resuscitation (CR) or standard resuscitation (SR).47 CR patients received 250 mL of fluid if they had no radial pulse or an SBP of lower than 70 mm Hg, and additional 250-mL boluses were given only to maintain a radial pulse or an SBP of 70 mm Hg or greater. The SR group patients received 2 L initially and additional fluid as needed to maintain an SBP of 110 mm Hg or greater. This protocol was maintained until hemorrhage control or 2 hours after arrival in the hospital. The results showed that among the cohort of patients with blunt trauma, 24-hour mortality was 3% and 18% in CR and SR groups, respectively, with an adjusted odds ratio of 0.17(0.03 to 0.92).47 At present, published data only support careful small volume crystalloid resuscitation to keep the injured alive during transport, and aggressive crystalloid resuscitation should be avoided. In the future, preserved blood products or specialized life-saving drugs may become available, but for now rapid transport to a specialized trauma center (“scoop and run”) is the most reasonable strategy in urban environments where the transport times are relatively short. In fact, a prospective comparison between Advanced Life Support (ALS) and Basic Life Support (BLS) transport48 showed that patients transported by ALS units more often underwent prehospital interventions (97% vs. 17%; p <0.01), including endotracheal intubation, needle thoracostomy, cervical collar, IV placement, and crystalloid resuscitation. While ALS ambulance on-scene time was significantly longer than that of BLS (p <0.01), and had more prehospital interventions (1.8 ± 1.0 per ALS patient vs. 0.2 ± 0.5 per BLS patient; p <0.01), 69.5% ALS patients and 88.4% of BLS patients (p <0.01) survived to hospital discharge. The message is clear – less is more in the prehospital arena, and time to definitive care is the most critical variable. Others studies also support the argument that rapid transport to the trauma center is more important than prehospital interventions in urban victims of penetrating trauma.49
Table 19-5 Criteria for Consideration of Transfer from Level III Centers to Level I or II Centers
Algorithm 19-1. 2011 Guidelines for the Field Triage of the Injured Patients. Source: Sasser SM, Hunt RC, Faul M, et al. Centers for Disease Control and Prevention. Guidelines for field triage of injured patients: recommendations of the National Expert Panel on Field Triage, 2011. MMWR Recomm Rep 2012;61(RR-1):1–20. Available at: https://stacks.cdc.gov/view/cdc/23038/Share.
EARLY HOSPITAL CARE AND PRIORITIES
The Advanced Trauma Life Support (ATLS) program has put together a systematic, concise approach to the care of a trauma patient. ATLS was developed by the American College of Surgeons, Committee on Trauma (COT) and was first introduced in 1980, and is now taught in 60 countries across the world. The ATLS program provides participants with a safe, reliable method for immediate management of the injured patient and the basic knowledge necessary to:
1. Assess the patient’s condition rapidly and accurately
2. Resuscitate and stabilize the patient according to priority
3. Determine if the patient’s needs exceed a facility’s capacity
4. Arrange appropriately for the patient’s interhospital transfer (who, what, when, and how)
5. Assure that optimum care is provided and that the level of care does not deteriorate at any point during the evaluation, resuscitation, or transfer process.
The basic concepts of ATLS include a primary survey focusing on the ABCDE (airway, breathing, circulation, disability, and exposure) to identify and address the life-threating issues, followed by a secondary survey to identify all the injuries. The specific treatments of various injuries have been addressed in the different chapters that follow. However, the key points to emphasize are that the two main killers during the early hospital period (“golden hour”) are the same as the prehospital setting – bleeding and TBI. As treatments for TBI remain mostly supportive, bleeding remains the number 1 cause of preventable deaths during the first 6 hours following trauma. In a bleeding patient, the priorities are to identify and control the source of bleeding in the shortest possible period of time. Sometimes the bleeding source is obvious and can be controlled by direct compression (e.g., stab wound to the femoral artery in the groin), tourniquets (e.g., mangled extremity), or sutures (e.g., scalp laceration), but often it is inside a body cavity. Localization of the hemorrhage source is relatively easy for penetrating trauma that is limited to a specific body region, but is notoriously difficult in the blunt polytrauma patients. In these patients, it helps to remember that a person can only lose significant volumes of blood in five locations: (1) pleural space, (2) intra-abdominal, (3) pelvis/retroperitoneal space, (4) soft tissues at the site of long bone fractures/sheer injuries, and (5) externally. With a quick examination and a chest x-ray, three of these possibilities can be easily ruled out, and the choice typically comes down to deciding between the abdomen and the pelvis. If the pelvic x-ray shows a significant pelvic fracture then the possibility of pelvic bleeding source is significant, and a pelvic binder should be applied promptly (or pelvis wrapped tightly with a sheet) for appropriate fracture patterns (e.g., suitable for open book fracture but potentially harmful for severe lateral compression). Focused assessment with sonography for trauma (FAST) can also be helpful in determining the source of hemorrhage. If it shows free fluid in the abdomen in a hypotensive patient then emergent laparotomy for hemorrhage control is justified. However, FAST has been reported to have a significant false negative rate even in experienced hands.50 Although diagnostic peritoneal lavage has largely been abandoned, a bedside diagnostic peritoneal aspiration (DPA) can be very helpful in these patients. Percutaneous DPA can be performed in 1 minute, and it has been shown to be accurate, safe, and superior to FAST for the diagnosis of abdominal blood as the source of hemodynamic instability in multitrauma patients.51 In addition to hemorrhage control, massive transfusion protocol (MTP) should be activated in patients that are actively bleeding to avoid development of trauma associated coagulopathy (TAC). Normal coagulation homeostasis depends on a delicate balance between clot formation and breakdown. Normally, trauma tilts the balance in favor of clot formation at the site of injuries to stop the bleeding. However, major injuries, excessive blood loss, prolonged tissue hypoperfusion, and TBI with disruption of the blood–brain barrier have all been shown to upset the normal coagulation homeostasis, resulting in TAC.52,53 This can manifest as abnormal clot formation and/or excessive or rapid clot breakdown (fibrinolysis). Unless treated promptly, this coagulopathy leads to further bleeding, which sets up a vicious cycle resulting in the “lethal triad” of coagulopathy, acidosis, and hypothermia that is associated with an extremely high mortality.54 An analysis of trauma-associated coagulopathy suggests that depletion coagulopathy results in abnormalities of traditional coagulation parameters (international normalized ratio, partial thromboplastin time) and predicts mortality, whereas fibrinolytic coagulopathy predicts infection, end-organ failure, and mortality, without a detectable difference in international normalized ratio or partial thromboplastin time.55
Damage Control Resuscitation (DCR) or Hemostatic Resuscitation has recently emerged as a potential solution for the prevention and reversal of trauma-associated coagulopathy.30,56,57 This approach advocates early hemorrhage control, permissive hypotension (until control of hemorrhage), avoidance of crystalloids, and early use of blood components such as fresh frozen plasma (FFP) and platelets (in 1:1 or 1:2 ratios). A recent prospective randomized multi-institutional trial enrolled 680 patients with severe trauma and major bleeding to test two different blood component ratios.22 In this study, early administration of plasma, platelets, and red blood cells in a 1:1:1 ratio compared with a 1:1:2 ratio did not result in significant differences in mortality at 24 hours or at 30 days. However, more patients in the 1:1:1 group achieved hemostasis and fewer experienced death due to exsanguination by 24 hours.22 It must be emphasized, however, that high-ratio protocols do not improve survival in patients who do not require massive transfusion (10 U of packed red blood cells [PRBCs] in 24 hours).58 In fact, inappropriate infusion of plasma in patients who are not massively bleeding can worsen their outcomes.59 So, while the exact ratio of PRBCs to FFP and platelets may be debatable, contemporary resuscitation practices have moved to early delivery of component therapy in the setting of massive bleeding, using standardized protocols (see below). Also, it should be emphasized that resuscitation is an adjunct to early hemorrhage control and not a substitute. In a bleeding patient, initiating DCR is a great idea, but the patient’s eventual survival depends on the ability (or inability) to obtain rapid and reliable hemorrhage control. In addition, prevention and active reversal of hypothermia (e.g., use of fluid warmers, body warming devices) and correction of acidosis and hypocalcemia are critically important while resuscitation protocols are carried out.
We also know that development of a clot is just the initial part of the process, and rapid breakdown of the clot may be equally detrimental. It has been shown that development of fibrinolysis is a highly lethal event in severely injured patients,60,61 and treatment with blood products alone is incapable of reversing this process. Despite ongoing debate about precise ratios of blood products,62 there is general agreement that blood products should be administered in the form of a MTP to optimize the processes of care and to improve outcomes. Cotton et al.63 tested the effectiveness of a trauma exsanguination protocol by comparing patients treated with the protocol (n = 94 over 18 months) to a cohort of similar patients admitted during the prior 18 months (n = 117). The study found that implementation of the protocol reduced 30-day mortality (51% vs. 66%, P <0.03), decreased intraoperative crystalloid administration (4.9 L vs. 6.7 L, P = 0.002), and reduced postoperative blood product use (2.8 U of PRBCs vs. 8.7 U, P <0.001; 1.7 U of FFP vs. 7.9 U, P <0.001; 0.9 U of platelets vs. 5.7 U, P <0.001). Dente et al.64 conducted a similar study of an MTP (1:1:1 ratio of platelets, FFP, and PRBCs) by comparing matched patients during a 1-year period before and after implementation of protocol (73 patients in the protocol group and 84 matched controls). Implementation of the protocol was found to reduce mortality in the first 24 hours (17% with MTP vs. 36% pre-MTP, P = 0.008) and at 30 days (34% vs. 55%, P = 0.04), with a more pronounced impact in the patients with blunt trauma.64 This study also showed that MTP patients required fewer overall transfusions of PRBCs and FFP after the first 24 hours (2.7 vs. 9.3 U of PRBCs, P <0.0001; 3 vs. 7.5 U of FFP, P <0.05). The University of Michigan MTP is provided for reference (Fig. 19-8).65 It should be emphasized that to be effective these protocols should not only incorporate the best available evidence, which is constantly changing, but also reflect the resources and realities of the individual institutions.
Figure 19-8. Massive transfusion protocol (MTP). BB, blood bank; BUN, serum urea nitrogen; CBC, complete blood count; Cryo, cryoprecipitate; FFP, fresh frozen plasma; INR, international normalized ratio; PCC, prothrombin complex concentrates; Plts, platelets; PRBCs, packed red blood cells; PT, prothrombin time; PTT, partial thromboplastin time; rFVIIa, recombinant factor VIIa. T&S, type and screen; VS, vital signs; U/O, urine output; CVCOR, cardiovascular center operating room; DDAVP, Desmopressin (1-desamino-8-D-arginine vasopressin); PRN, as needed (Pro re nata); ABG, arterial blood gas; D/C, discontinue. Reproduced with permission from the University of Michigan Hospitals and Health Centers.65
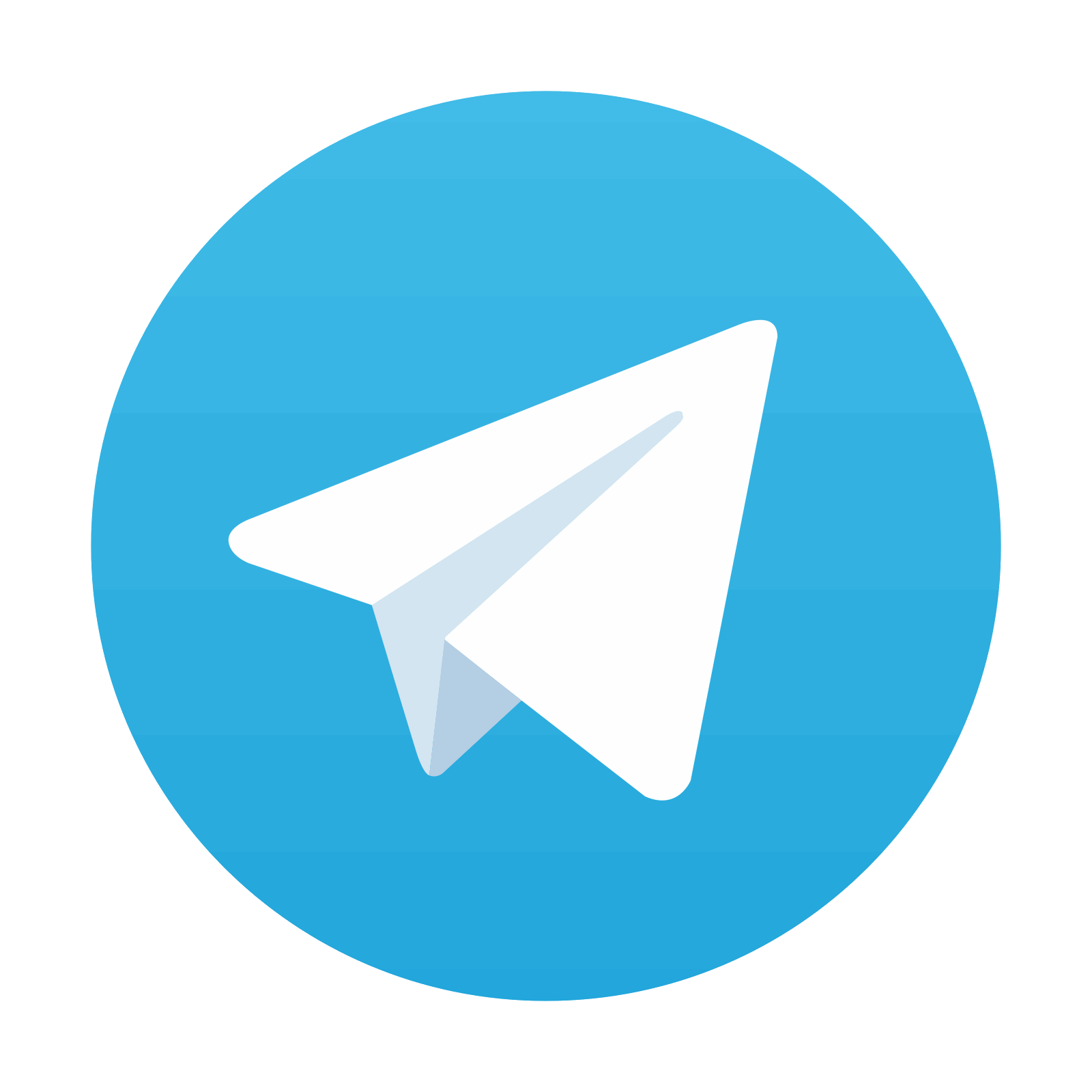
Stay updated, free articles. Join our Telegram channel

Full access? Get Clinical Tree
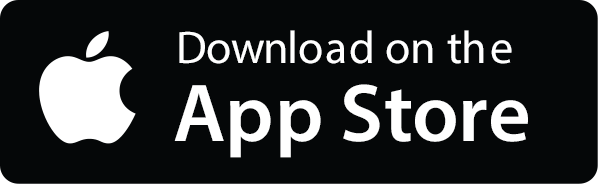
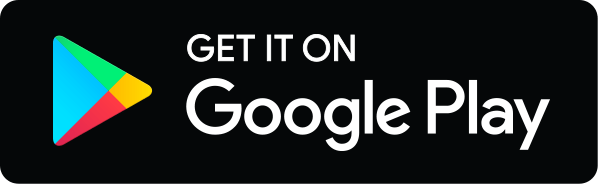