Key Terms
Bioaccumulation
Body burden
Dose–response relationship
Drugs of abuse
ED50
Individual dose–response relationship
LD50
Poisons
Quantal dose–response relationship
TD50
Therapeutic index
Toxicokinetics
Toxicology
Toxins
Xenobiotics
Toxicology is the study of the adverse effects of xenobiotics in humans. Xenobiotics are chemicals and drugs that are not normally found in or produced by the body. The scope of toxicology is very broad and includes three major disciplines: mechanistic, descriptive, and regulatory toxicology. Mechanistic toxicology elucidates the cellular, molecular, and biochemical effects of xenobiotics within the context of a dose–response relationship between the xenobiotic and its adverse effect(s). Mechanistic studies provide a basis for rational therapy design and the development of laboratory tests to assess the degree of exposure in individuals. Descriptive toxicology uses the results from animal experiments to predict what level of exposure will cause harm in humans. This process is known as risk assessment. In regulatory toxicology, combined data from mechanistic and descriptive studies are used to establish standards that define the level of exposure that will not pose a risk to public health or safety. Typically, regulatory toxicologists work for, or in conjunction with, government agencies. The Food and Drug Administration (FDA) oversees human safety issues associated with therapeutic drugs, cosmetics, and food additives. The U.S. Environmental Protection Agency (EPA) has regulatory oversight with regard to pesticides, fungicides, rodenticides, and industry-related chemicals that may threaten safe drinking water and clean air. The Occupational Safety and Health Administration (OSHA) is responsible for ensuring safe and healthy work environments. The Consumer Product Safety Commission (CPSC) regulates household chemicals, while the Department of Transportation (DOT) oversees transportation of hazardous chemicals.
There are also a number of specialties within toxicology, including forensic, clinical, and environmental toxicology. Forensic toxicology is primarily concerned with the medical and legal consequences of exposure to chemicals or drugs. A major focus of forensic toxicology is establishing and validating the analytic performance of test methods used to generate evidence in legal situations, including cause of death. Clinical toxicology focuses on the relationships between xenobiotics and disease states. This area emphasizes not only diagnostic testing but also therapeutic intervention. Environmental toxicology includes the evaluation of environmental chemical pollutants and their impact on human health. This is a growing area of concern as we learn more about the mechanisms of action of these chemicals and their adverse effects on human health. Another goal of environmental toxicology is to monitor occupational health issues and to increase public health biomonitoring efforts nationwide.
Within the organizational structure of a typical clinical laboratory, toxicology is usually considered a specialty of clinical chemistry because the qualitative and quantitative methodologies used to measure xenobiotics overlap with this discipline. However, appropriate diagnosis and management of patients with acute poisoning or chronic exposure to xenobiotics requires an integrated approach from all sections of the clinical laboratory.
XENOBIOTICS, POISONS, AND TOXINS
The terms, xenobiotic, poison, and toxin are often used interchangeably; however, there are some important distinctions that should be made between them. Xenobiotics, as previously mentioned, are defined as exogenous agents that can have an adverse effect on a living organism. This term is more often used to describe environmental exposure to chemicals or drugs. Examples of environmental drug exposures include antibiotics and antidepressants; chemical exposures might include perfluorinated and brominated compounds. Similarly, poisons are also exogenous agents that have an adverse effect on a biological system; however, this term is more often used when describing substances from an animal, plant, mineral, or gas. Examples include venoms from poisonous snakes or spiders, poison hemlock, arsenic, lead, and carbon monoxide. Toxins, however, are endogenous substances biologically synthesized either in living cells or in microorganisms. Examples include botulinum toxin produced from the microorganism, Clostridium botulinum, hemotoxins produced from venomous snakes, and mycotoxins produced from fungus. The terms toxicant and toxic refer to substances that are not produced within a living cell or microorganism and are more commonly used to describe environmental chemicals.
From a clinical standpoint, almost 50% of poisoning cases are intentional suicide attempts, accidental exposure accounts for about 30%, and the remaining cases are a result of homicide or occupational exposure. Of these, suicide has the highest mortality rate. Accidental exposure occurs most frequently in children; however, accidental drug overdose of either therapeutic or illicit drugs is relatively common in adolescents and adults. Occupational exposure primarily occurs in industrial and agricultural settings, but is an expanding area of concern as we learn more about the role of various chemical agents and their contribution to disease.
ROUTES OF EXPOSURE
Toxins can enter the body via several routes, with ingestion, inhalation, and transdermal absorption being the most common. Of these, ingestion is most often observed in the clinical setting. For most toxins to exert a systemic effect, they must be absorbed into circulation. Absorption of toxins from the gastrointestinal tract occurs via several mechanisms. Some toxins are taken up by processes intended for dietary nutrients; however, most are passively absorbed through diffusion. Diffusion requires that the substance be able to cross the cellular barriers of the gastrointestinal tract. Hydrophobic substances do have the ability to diffuse across cell membranes and, therefore, can be absorbed anywhere along the gastrointestinal tract. Ionized substances, however, cannot passively diffuse across the membranes. Weak acids can become protonated in gastric acid resulting in a nonionized species, which can be absorbed in the stomach. In a similar manner, weak bases can be absorbed in the intestine where the pH is largely neutral or slightly alkaline. Other factors that influence the absorbance of toxins from the gastrointestinal tract include the rate of dissolution, gastrointestinal motility, resistance to degradation in the gastrointestinal tract, and interaction with other substances. Toxins that are not absorbed from the gastrointestinal tract do not produce systemic effects, but may produce local effects, such as diarrhea, bleeding, and malabsorption, which may cause systemic effects secondary to toxin exposure.
DOSE–RESPONSE RELATIONSHIP
The concept that all substances have the potential to cause harm, even water, is a central theme in toxicology. Paracelsus (1493 to 1591) pioneered the use of chemicals in medicine and coined the term “the dose makes the poison.” Understanding this, dose–response relationship is fundamental and essential to modern toxicology. To enable assessment of substances’ potential to cause pathologic effects, it is necessary to establish an index of the relative toxicities of the substances. Several systems are available, but most correlate the dose of a xenobiotic that will result in harmful effects. One such system correlates a single acute oral dose range with the probability of a lethal outcome in an average 70-kg man (Table 31.1). This is a useful system to compare the relative toxicities of substances as the predicted response is death, which is valid. However, most xenobiotics can express pathologic effects other than death at lower degrees of exposure; therefore, other indices have been developed.
TABLE 31.1 Toxicity Rating System
Adapted from Klaassen CD. Principles of toxicology. In: Klaassen CD, Amdur MO, Doull J, eds. Toxicology: the Basic Science of Poisons. 3rd ed. New York, NY: Macmillan; 1986:13.
A more in-depth characterization can be acquired by evaluating data from a cumulative frequency histogram of toxic responses over a range of doses. This experimental approach is typically used to evaluate responses over a wide range of concentrations. One response monitored is the toxic response or the response associated with an early pathologic effect at lower than lethal doses. This response has been determined to be an indicator of the toxic effects specific for that toxin. For a substance that exerts early toxic effects by damaging liver cells, the response monitored may be increases in serum alanine aminotransferase (ALT) or γ-glutamyltransferase (GGT) activity. The dose–response relationship implies that there will be an increase in the toxic response as the dose is increased. It should be noted that not all individuals display a toxic response at the same dose. The population variance can be seen in a cumulative frequency histogram of the percentage of people producing a toxic response over a range of concentrations (Fig. 31.1). The TD50 is the predicted dose that would produce a toxic response in 50% of the population. If the monitored response is death, the LD50 is the predicted dose that would result in death in 50% of the population. Similar experiments can be used to evaluate the doses of therapeutic drugs. The ED50 is the dose that would be predicted to be effective or have a therapeutic benefit in 50% of the population. The therapeutic index is the ratio of the TD50 (or LD50) to the ED50. Drugs with a large therapeutic index demonstrate fewer toxic adverse effects when the dose of the drug is in the therapeutic range.
FIGURE 31.1 Dose–response relationship. Comparison of responses of a therapeutic drug over a range of doses. The ED50 is the dose of drug in which 50% of treated individuals will experience benefit. The TD50 is the dose of drug in which 50% of individuals will experience toxic adverse effects. The LD50 is the dose of drug in which 50% of individuals will result in mortality.
Dose–response relationships may apply to an individual or a population. The individual dose–response relationship relates to the individual’s health status as well as changes in xenobiotic exposure levels. A quantal dose–response relationship describes the change in health effects of a defined population based on changes in the exposure to the xenobiotic.
Acute and Chronic Toxicity
Acute toxicity and chronic toxicity are terms used to relate the duration and frequency of exposure to observed toxic effects. Acute toxicity is usually associated with a single, short-term exposure to a substance in which the dose is sufficient to cause immediate toxic effects. Chronic toxicity is generally associated with repeated and frequent exposure for extended periods of time (months to years) at doses that are insufficient to cause an immediate acute response. In many instances, chronic exposure is related to an accumulation of the toxicant or the toxic effects within the individual. Chronic toxicity may affect different systems than those associated with acute toxicity; therefore, dose–response relationships may differ for acute and chronic exposures for the same xenobiotic.
ANALYSIS OF TOXIC AGENTS
Toxicology testing may be performed to screen for the presence of a number of agents that might be present (e.g., drug screens, heavy metal panels) or as targeted testing. Targeted testing might be performed when an environmental risk of exposure is known (e.g., industrial workers, chemical plants), to support the investigation of an exposure (e.g., chemical spill, suicide attempt), to comply with occupational regulations or guidelines (e.g., OSHA), or to confirm clinical suspicions of poisoning (e.g., arsenic, cyanide). Due to nonspecific signs and symptoms of toxicity and that frequently the duration and extent of exposure are unknown, diagnosis of most toxic element exposures depends heavily on laboratory testing.
In general, toxicology testing is performed on urine or blood specimens. In selecting the best specimen for a specific test, it is important to recognize that toxic agents exhibit unique absorption, distribution, metabolism, and elimination kinetics or toxicokinetics. As such, the predicted toxicokinetics of the individual element(s) being tested must be coordinated with the selection of specimen type and timing of collection relative to the time of exposure. An exposure could be missed entirely if testing is performed on an inappropriate specimen. For example, exposure to methylmercury could be missed if testing is performed on urine as methylmercury is primarily excreted in fecal material. An exposure to arsenic could be missed if testing is performed with blood collected a few days after the exposure due to the short half of arsenic in blood.
Preanalytical variables such as elimination patterns, analyte stability, and specimen collection procedures must be considered. For urine testing, 24-hour collections are preferred in order to compensate for variable elimination patterns throughout the day. Reporting results per gram of creatinine is also common to account for variable excretion and renal function. Random urine collections may not provide the most accurate profile of exposure when compared to a 24-hour collection, but they are useful for screening and qualitative detection of exposure to several potentially toxic agents. Any elevated result that is inconsistent with clinical expectations should be confirmed by testing a second specimen collection or a second specimen type.
One challenge of specimen collection for toxicological studies is that several aspects of the collection, handling, and storage can introduce external contamination into the sample. Common sources of external contamination include patient clothing, skin, hair, collection environment (e.g., dust, aerosols, antiseptic wipes), and specimen handling variables (e.g., container, lid, preservatives). Concentrated acids are commonly used as urine preservatives; however, contaminants may also be introduced in either the acid itself or in the process used to add the acid to the urine (e.g., pipette tips). Specimen containers and lids should also be devoid of contaminating organic and inorganic agents that may interfere with analytical testing. For example, certified “trace element-free” blood collection tubes are available; these tubes commonly have a royal blue top and can be used for most trace elements testing though a tan-top tube is manufactured specifically for lead determinations. Another consideration when handling biological specimens for metals testing is the use of acid-washed pipette tips, containers, and other supplies to prevent contamination. Laboratories may also need to exercise precautions to prevent loss of toxic agents due to in vitro volatilization and metabolism. For instance, mercury and arsenic are particularly vulnerable to loss and metabolism, respectively, during sample processing and storage. These scenarios represent only a fraction of the many specimen handling considerations necessary to reduce preanalytical error in toxicology testing.
Analysis of toxic agents in a clinical setting is typically a two-step process. The first step is a screening test, which is a rapid, simple, qualitative procedure intended to detect the presence of specific substances or classes of toxicants. In general, these procedures have good analytic sensitivity but lack specificity. A negative result can rule out a drug or toxicant; however, a positive result should be considered a presumptive positive until confirmed by a second, more specific method, which is the second step of the test process, or a confirmatory test. Confirmatory tests are generally quantitative and report the concentration of the substance in the specimen in contrast to qualitative screening tests that provide a result of positive (drug is present) or negative (drug is absent). A variety of analytical methods can be used for screening and confirmatory testing though immunoassays are the commonly used for drug screens. In some instances, these assays are specific for a single drug (e.g., tetrahydrocannabinol [THC]), but in most cases, the assay is to detect drugs within a general class (e.g., barbiturates and opiates). Thin-layer chromatography (TLC) is a relatively simple, inexpensive method for detecting various drugs and other organic compounds; gas chromatography (GC) is widely used and a well-established technique for qualitative and quantitative determination of many volatile substances. The reference method for quantitative identification of most organic compounds is gas chromatography coupled with a mass spectrometer (GC–MS) as the detector. Inorganic compounds, including speciation, may be quantitated using inductively coupled plasma-mass spectrometry (ICP-MS) or atomic absorption (AA) methods.
TOXICOLOGY OF SPECIFIC AGENTS
Many chemical agents encountered on a regular basis have potential for toxicity. The focus of this section is to discuss some of the most commonly encountered nondrug toxins seen in a clinical setting, as well as those that present as medical emergencies with acute exposure.1
Alcohols
The toxic effects of alcohol are both general and specific. Exposure to alcohol, like exposure to most volatile organic solvents, initially causes disorientation, confusion, and euphoria, but can progress to unconsciousness, paralysis, and, with high-level exposure, even death. Most alcohols display these effects at about equivalent molar concentrations. This similarity suggests a common depressant effect on the central nervous system (CNS) that appears to be mediated by changes in membrane properties. In most cases, recovery from CNS effects is rapid and complete after cessation of exposure.
Distinct from the general CNS effects are the specific toxicities of each type of alcohol, which are usually mediated by biotransformation of alcohols to toxic products. There are several pathways by which short-chain aliphatic alcohols can be metabolized. Of these, hepatic conversion to an aldehyde, by alcohol dehydrogenase (ADH), and further conversion to an acid, by hepatic aldehyde dehydrogenase (ALDH), is the most significant.

Ethanol
Ethanol exposure is common, and excessive consumption, with its associated consequences, is a leading cause of economic, social, and medical problems throughout the world.2 The economic impact is estimated to exceed $100 billion per year in terms of lost wages and productivity. Many social and family problems are associated with excessive ethanol consumption, and the burden to the health care system is significant. Ethanol-related disorders are consistently one of the top ten causes of hospital admissions, and approximately 20% of all hospital admissions have some degree of alcohol-related problems. It is estimated that 80,000 Americans die each year, either directly or indirectly, as a result of abusive alcohol consumption. This correlates to about a fivefold increase in premature mortality. In addition, consumption of ethanol during pregnancy may lead to fetal alcohol syndrome or fetal alcohol effects, both of which are associated with delayed motor and mental development in children.
Correlations have been established between blood alcohol concentration and the clinical signs and symptoms of acute intoxication. A blood alcohol concentration of 80 mg/dL has been established as the statutory limit for operation of a motor vehicle in the United States as this concentration is associated with a diminution of judgment and motor function. Determinations of blood ethanol concentration by the laboratory may be used in litigation of drunken driving cases and requires appropriate chain-of-custody procedures for specimen collection and documentation of acceptable quality control performance, instrument maintenance procedures, and proficiency testing records. Approximately 50% of the 40,000 to 50,000 annual automobile-related fatalities in the United States involve alcohol as a factor.
Besides the short-term effects of ethanol, most pathophysiologic consequences of ethanol abuse are associated with chronic consumption. In an average adult, this correlates to the consumption of about 50 g of ethanol per day for about 10 years. This pattern of consumption has been associated with compromised function of various organs, tissues, and cell types; however, the liver appears to be affected the most. The pathologic sequence starts with the accumulation of lipids in hepatocytes. With continued consumption, this may progress to alcoholic hepatitis. In about 20% of individuals with long-term, high-level alcohol intake, this develops into a toxic form of hepatitis. Of those who do not progress to toxic hepatitis, progression to liver cirrhosis is common. Cirrhosis of the liver can be characterized as fibrosis leading to functional loss of the hepatocytes. Progress through this sequence is associated with changes in many laboratory tests related to hepatic function including liver enzymes. Several laboratory indicators have the required diagnostic sensitivity and specificity to identify excessive ethanol consumption and most correlate well to the progression of ethanol-induced liver disease. Table 31.2 lists common laboratory indicators of prolonged ethanol consumption.
TABLE 31.2 Common Indicators of Ethanol Abuse
GGT, γ-glutamyltransferase; AST, aspartate aminotransferase; ALT, alanine aminotransferase; HDL, high-density lipoprotein; MCV, mean cell volume.
Several mechanisms have been proposed to mediate the pathologic effects of long-term ethanol consumption. Of these, adduct formation with acetaldehyde appears to play a key role. Hepatic metabolism of ethanol is a two-step enzymatic reaction with acetaldehyde as a reactive intermediate. Most ethanol is converted to acetate, or acetic acid, in this pathway; however, a significant portion of the acetaldehyde intermediate is released in the free state.

Extracellular acetaldehyde is a transient species as a result of rapid adduct formation with amine groups of proteins. Many of the pathologic effects of ethanol have been correlated with the formation of these adducts, and formation of acetaldehyde adducts has also been shown to change the structure and function of various proteins.
Methanol
Methanol is a common laboratory solvent that is also found in many household cleaners. It may be ingested accidentally as a component of many commercial products or as a contaminant of homemade liquors. Methanol is initially metabolized by hepatic ADH to the intermediate formaldehyde. Formaldehyde is then rapidly converted to formic acid by hepatic ALDH. The formation of formic acid causes severe metabolic acidosis, which can lead to tissue injury and possible death. Formic acid is also responsible for optic neuropathy that can lead to blindness.
Isopropanol
Isopropanol, also known as rubbing alcohol, is also commercially available. It is metabolized by hepatic ADH to acetone, which is its primary metabolic end product. Both isopropanol and acetone have CNS depressant effects similar to ethanol; however, acetone has a long half-life, and intoxication with isopropanol can therefore result in severe acute-phase ethanol-like symptoms that persist for an extended period.
Ethylene Glycol
Ethylene glycol (1,2-ethanediol) is a common component of hydraulic fluid and antifreeze. Ingestion by children is relatively common because of its sweet taste. The immediate effects of ethylene glycol ingestion are similar to those of ethanol; however, metabolism by hepatic ADH and ALDH results in the formation of several toxic species including oxalic acid and glycolic acid, which result in severe metabolic acidosis. This is complicated by the rapid formation and deposition of calcium oxalate crystals in the renal tubules. With high levels of consumption, calcium oxalate crystal formation in the kidneys may result in renal tubular damage.
Determination of Alcohols
From a medicolegal perspective, determinations of blood ethanol concentrations must be accurate and precise. Serum, plasma, and whole blood are acceptable specimens, and correlations have been established between ethanol concentration in these specimens and impairment of psychomotor function. Because ethanol uniformly distributes in total body water, serum, which has greater water content than whole blood, has a higher concentration per unit volume. Because of this difference in distribution, most states have standardized the acceptable specimen types admissible as evidence, and some jurisdictions even mandate a specific method (often GC) be used for legal ethanol determination.
When acquiring a specimen for ethanol determination, several preanalytical issues must be considered to ensure the integrity of the sample. One of these requirements is that the venipuncture site should only be cleaned with an alcohol-free disinfectant. Also, because of the volatile nature of short-chain aliphatic alcohols, specimens must be capped at all times to avoid evaporation. Sealed specimens can be refrigerated or stored at room temperature for up to 14 days without loss of ethanol. Nonsterile specimens or those intended to be stored for longer periods of time should be preserved with sodium fluoride to avoid increases in ethanol content resulting from contamination due to bacterial fermentation.
CASE STUDY 31.1
A patient with a provisional diagnosis of depression was sent to the laboratory for a routine workup. The complete blood cell count was unremarkable except for an elevated erythrocyte mean cell volume. Results of the urinalysis were unremarkable. The serum chemistry testing revealed slightly increased aspartate aminotransferase (AST), total bilirubin, and high-density lipoproteins (HDL). All other chemistry results, including glucose, urea, creatinine, cholesterol, pH, bicarbonate/carbon dioxide (pCO2), alanine aminotransferase (ALT), sodium, and potassium, were within the reference intervals. The physician suspects ethanol abuse; however, the patient denies an alcohol use. Subsequent testing revealed a serum γ-glutamyltransferase (GGT) three times the upper limit of the reference interval. No ethanol was detected in serum, and screening tests for infectious forms of hepatitis were negative.
questions
1. Are these results consistent with a patient who is consuming hazardous quantities of ethanol?
2. What additional testing would you recommend to rule ethanol abuse in or out?
Several analytic methods can be used to determine the concentration of ethanol in serum. Among these, osmometric, chromatographic, and enzymatic methods are the most commonly used. When osmolality is measured by freezing point depression, increases in serum osmolality correlate well with increases in serum ethanol concentration. The degree of increase in osmolality due to the presence of ethanol is expressed as the difference between the measured and the calculated osmolality otherwise referred to as the osmolal gap. It has been established that serum osmolality increases by approximately 10 mOsm/kg for each 60 mg/dL increase in serum ethanol; therefore, the osmolal gap is useful for estimating the amount of ethanol present in the serum. The osmolal gap can be calculated as follows:

This relationship, however, is not specific to ethanol, and increases in the osmolal gap also occur with certain metabolic imbalances. Therefore, use of the osmolal gap for determination of serum or blood ethanol concentration lacks analytic specificity; however, it is a useful screening test.
GC is the established reference method for ethanol determinations and is quite useful as it can simultaneously quantitate other alcohols as well, such as methanol and isopropanol. Analysis begins with dilution of the serum or blood sample with a saturated solution of sodium chloride in a closed container. Volatiles within the liquid specimen partition into the airspace (head space) of the closed container. Sampling of the head space provides a clean specimen with little or no matrix effect. Quantitation can be performed by constructing a standard curve or calculating the concentration based on relative changes to an internal standard (n-propanol) as shown in Figure 31.2.
FIGURE 31.2 Headspace gas chromatography of alcohol. The concentration of each alcohol can be determined by comparison to the response from the internal standard n-propanol.
Enzymatic methods for ethanol determination use a nonhuman form of ADH to oxidize ethanol in the specimen to acetaldehyde with simultaneous reduction of NAD+ to NADH.

The NADH produced can be monitored directly by absorbance at 340 nm or can be coupled to an indicator reaction. This form of ADH is relatively specific for ethanol (Table 31.1), and intoxication with methanol or isopropanol produces a negative or low result; therefore, a negative result by this method does not rule out ingestion of other alcohols. There is good agreement between the enzymatic reactions of ethanol and GC. The enzymatic reactions can be fully automated and do not require specialized instrumentation.
Carbon Monoxide
Carbon monoxide is produced by incomplete combustion of carbon-containing substances. The primary environmental sources of carbon monoxide include gasoline engines, improperly ventilated furnaces, and wood or plastic fires. Carbon monoxide is a colorless, odorless, and tasteless gas that is rapidly absorbed into circulation from inspired air.
When carbon monoxide binds to hemoglobin, it is called carboxyhemoglobin (COHb). The affinity of carbon monoxide for hemoglobin is 200 to 225 times greater than for oxygen.3,4 Air is approximately 20% oxygen by volume. If inspired air contained 0.1% carbon monoxide by volume, this would result in 50% carboxyhemoglobinemia at equilibrium. Because both carbon monoxide and oxygen compete for the same binding site, exposure to carbon monoxide results in a decrease in the concentration of oxyhemoglobin, and, for this reason, carbon monoxide is considered a very toxic substance.
Carbon monoxide expresses its toxic effects by causing a leftward shift in the oxygen–hemoglobin dissociation curve resulting in a decrease in the amount of oxygen delivered to the tissue.3 The net decrease in the amount of oxygen delivered to the tissue results in hypoxia. The major toxic effects of carbon monoxide exposure are seen in organs with high oxygen demand, such as the brain and heart. The concentration of COHb, expressed as the percentage of COHb, presents relative to the capacity of the specimen to form COHb, and the corresponding symptoms are detailed in Table 31.3. The only treatment for carbon monoxide poisoning is 100% oxygen therapy. In severe cases, hyperbaric oxygen may be used to promote distribution of oxygen to the tissues. The half-life of COHb is roughly 60 to 90 minutes in a patient with normal respiratory function breathing 100% oxygen.
TABLE 31.3 Symptoms of Carboxyhemoglobinemia
COHb, carboxyhemoglobin.
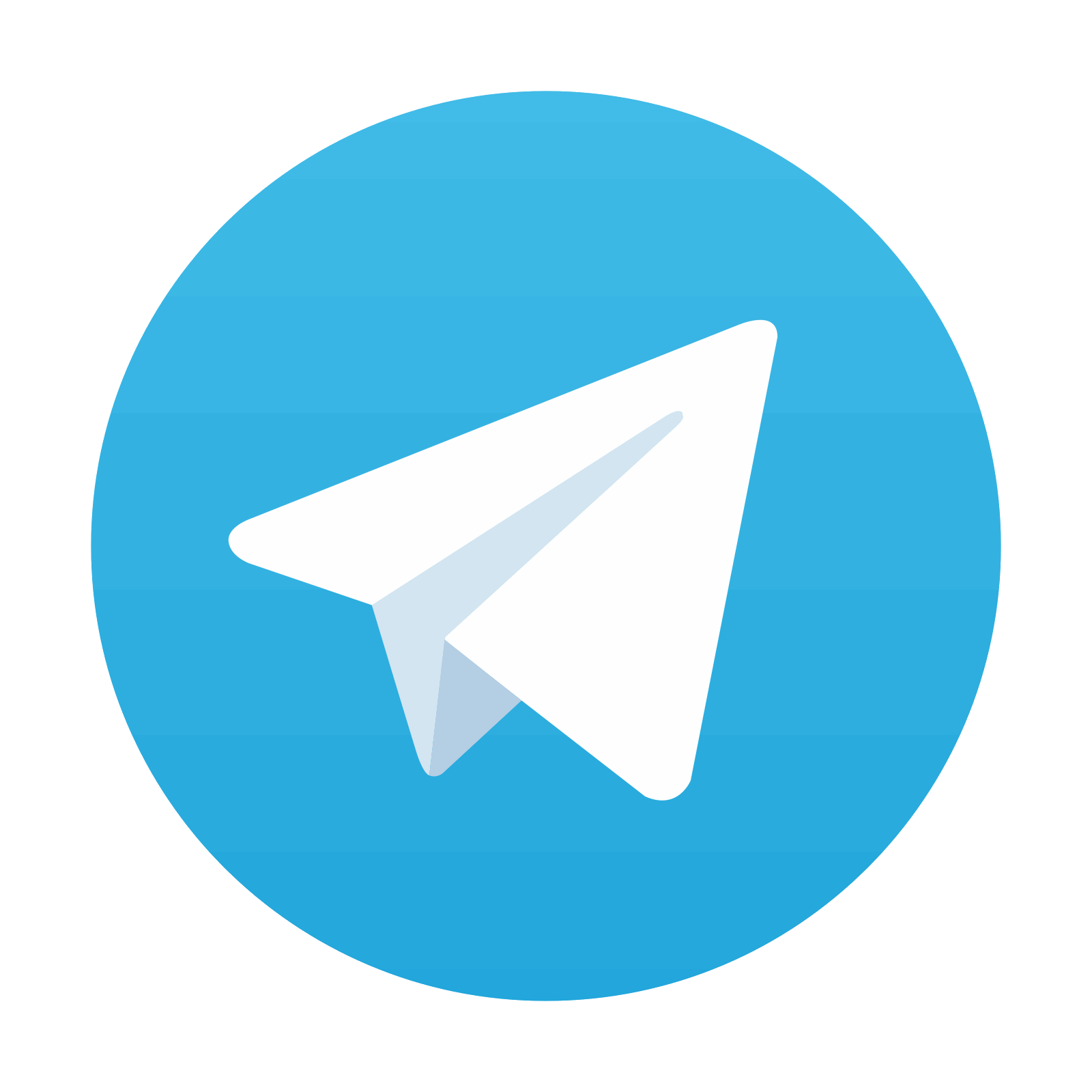
Stay updated, free articles. Join our Telegram channel

Full access? Get Clinical Tree
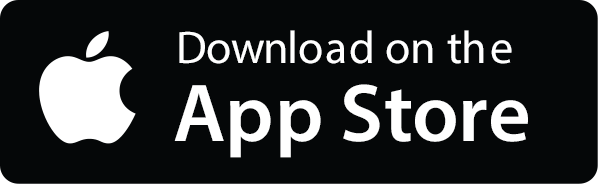
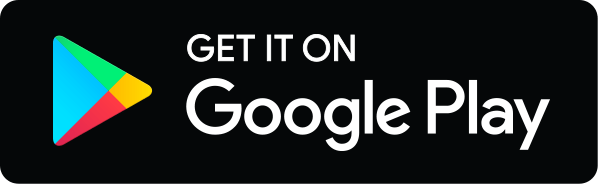