Thyroid and Anti-Thyroid Drugs
Thyroid hormone is essential for normal development, especially of the central nervous system (CNS). In the adult, thyroid hormone maintains metabolic homeostasis and influences the function of virtually all organ systems. Thyroid hormone contains iodine that must be supplied by nutritional intake. The thyroid gland contains large stores of thyroid hormone in the form of thyroglobulin. These stores maintain systemic concentrations of thyroid hormone despite variations in iodine availability and nutritional intake. The thyroidal secretion is predominantly the prohormone thyroxine, which is converted in the liver and other tissues to the active form, triiodothyronine. Local activation of thyroxine also occurs in target tissues (e.g., brain and pituitary) and is increasingly recognized as an important regulatory step in thyroid hormone action. Serum concentrations of thyroid hormones are precisely regulated by the pituitary hormone, thyrotropin (TSH), in a negative-feedback system. The predominant actions of thyroid hormone are mediated via nuclear thyroid hormone receptors (TRs) and modulating transcription of specific genes.
Overt hyperthyroidism and hypothyroidism, thyroid hormone excess or deficiency, are usually associated with dramatic clinical manifestations. Milder disease often has a more subtle clinical presentation and is identified based on abnormal biochemical tests of thyroid function. Maternal and neonatal hypothyroidism, due to iodine deficiency, remains the major preventable cause of mental retardation worldwide. Treatment of the hypothyroid patient consists of thyroid hormone replacement. Treatments for hyperthyroidism include anti-thyroid drugs to decrease hormone synthesis and secretion, destruction of the gland by the administration of radioactive iodine, or surgical removal. In most patients, disorders of thyroid function can be either cured or have their diseases controlled. Likewise, thyroid malignancies are most often localized and resectable. Metastatic disease often responds to radioiodide treatment but may become highly aggressive and unresponsive to conventional treatment.
THYROID
The thyroid gland produces 2 fundamentally different types of hormones. The thyroid follicle produces the iodothyronine hormones thyroxine (T4) and 3,5,3′-triiodothyronine (T3). The thyroid’s parafollicular cells (C cells) produce calcitonin (see Chapter 44).
BIOSYNTHESIS OF THYROID HORMONES. The thyroid hormones are synthesized and stored as amino acid residues of thyroglobulin, a complex glycoprotein made up of 2 apparently identical subunits (330 kDa each) and constituting the vast majority of the thyroid follicular colloid. The thyroid gland is unique in storing great quantities of potential hormone in this way, and extracellular thyroglobulin can represent a large portion of the thyroid mass. The major steps in the synthesis, storage, release, and interconversion of thyroid hormones are summarized in Figure 39–1 and described as follows:
Figure 39–1 Major pathways of thyroid hormone biosynthesis and release. Abbreviations: Tg, thyroglobulin; DIT, diiodotyrosine; MIT, monoiodotyrosine; TPO, thyroid peroxidase; HOI, hypoiodous acid; EOI, enzyme-linked species; D1 and D2, deiodinases; PTU, propylthiouracil; MMI, methimazole.
A. Uptake of Iodide. Iodine ingested in the diet reaches the circulation in the form of iodide ion (I–). Under normal circumstances, the I– concentration in the blood is very low (0.2-0.4 μg/dL; ~15-30 nM), but the thyroid actively transports the ion via a specific membrane-bound protein, termed the sodium-iodide symporter (NIS). As a result, the ratio of thyroid to plasma iodide concentration is usually between 20 and 50 and can exceed 100 when the gland is stimulated. Iodide transport is inhibited by a number of ions such as thiocyanate and perchlorate. Thyrotropin (thyroid-stimulating hormone [TSH]) stimulates NIS gene expression and promotes insertion of NIS protein into the membrane in a functional configuration. Thus, decreased stores of thyroid iodine enhance iodide uptake, and the administration of iodide can reverse this situation by decreasing NIS protein expression. Iodine accumulation throughout the body is mediated by a single NIS gene. Individuals with congenital NIS gene mutations have absent or defective iodine concentration in all tissues known to concentrate iodine.
B. Oxidation and Iodination. The oxidation of iodide to its active form is accomplished by thyroid peroxidase. The reaction results in the formation of monoiodotyrosyl (MIT) and diiodotyrosyl (DIT) residues in thyroglobulin just prior to its extracellular storage in the lumen of the thyroid follicle.
C. Formation of Thyroxine and Triiodothyronine from Iodotyrosines. The remaining synthetic step is the coupling of 2 diiodotyrosyl residues to form thyroxine (T4) or of a monoiodotyrosyl and a diiodotyrosyl residue to form triiodothyronine (T3). These oxidative reactions also are catalyzed by thyroid peroxidase. Intrathyroidal and secreted T3 are also generated by the 5′-deiodination of thyroxine.
D. Resorption; and Proteolysis of Colloid; E. Deiodination of DIT/MIT; F. deiodination of T4; G. Secretion of Thyroid Hormones. Because T4 and T3 are synthesized and stored within thyroglobulin, proteolysis is an important part of the secretory process. This process is initiated by endocytosis of colloid from the follicular lumen at the apical surface of the cell, with the participation of a thyroglobulin receptor, megalin. This “ingested” thyroglobulin appears as intracellular colloid droplets, which apparently fuse with lysosomes containing the requisite proteolytic enzymes. TSH enhances the degradation of thyroglobulin by increasing the activity of thiol endopeptidases of the lysosomes, which selectively cleave thyroglobulin, yielding hormone-containing intermediates that subsequently are processed by exopeptidases. The liberated hormones then exit the cell mostly as T4, some as T3, via a process of deiodination (Figure 39–2) that also occurs peripherally (Figure 39–3).
Figure 39–2 Pathways of iodothyronine deiodination.
Figure 39–3 Deiodinase isozymes. D1, type I iodothyronine 5′-deiodinase; D2, type II iodothyronine 5′-deiodinase; D3, type III iodothyronine 5-deiodinase; BAT, brown adipose tissue.
CONVERSION OF T4 TO T3 IN PERIPHERAL TISSUES. The normal daily production of T4 is estimated to range between 80 and 100 μg; that of T3 is between 30 and 40 μg. Although T3 is secreted by the thyroid, metabolism of T4 by 5′, or outer ring, deiodination in the peripheral tissues accounts for ~80% of circulating T3. In contrast, removal of the iodine on position 5 of the inner ring produces the metabolically inactive 3,3′,5′-triiodothyronine (reverse T3 or rT3; Figure 39–2). Under normal conditions, ~40% of T4 is converted to each of T3 and rT3, and ~20% is metabolized via other pathways, such as glucuronidation in the liver and excretion in the bile. Normal circulating concentrations of T4 in plasma range from 4.5-11 μg/dL; those of T3 are ~1/100 of that (60-180 ng/dL). Triiodothyronine has a much higher affinity for the nuclear thyroid hormone receptor compared with thyroxine and is much more potent biologically on a molar basis.
There are 3 iodothyronine deiodinases. Forms 1 and 2 (D1, D2) convert T4 to T3. D1 is expressed primarily in the liver and kidney, and also in the thyroid and pituitary (Figure 39–3). It is upregulated in hyperthyroidism; downregulated in hypothyroidism, and inhibited by the anti-thyroid drug propylthiouracil. D2 is expressed primarily in the CNS (including the pituitary and hypothalamus) and brown adipose tissue, also in the thyroid, and at very low levels in skeletal muscle. The activity of D2 is unaffected by propylthiouracil. D2 localizes to the endoplasmic reticulum, which facilitates access of D2-generated T3 to the nucleus. Hence organs that express D2 tend to use the locally generated T3. D2 is regulated by T4 such that elevated levels of the enzyme are found in hypothyroidism and suppressed levels are found in hyperthyroidism. Type 3 deiodinase (D3) catalyzes inner ring- or 5-deiodination, the main inactivating pathway of T3 metabolism; D1 performs this function to some extent. D3 is found at highest levels in the CNS and placenta, and is also expressed in skin and uterus. The 3 deiodinases contain the rare amino acid selenocysteine in their active sites. Mutations in 1 such protein, SECIS binding protein 2, are associated with abnormal circulating thyroid hormone levels.
TRANSPORT OF THYROID HORMONES IN THE BLOOD. The thyroid hormones are transported in the blood in strong but noncovalent association with certain plasma proteins.
Thyroxine-binding globulin (TBG) is the major carrier of thyroid hormones. It is a glycoprotein that binds 1 molecule of T4 per molecule of protein with a very high affinity (Kd’ is ~10–10 M). T3 is bound less avidly. T4, but not T3, also is bound by transthyretin (thyroxine-binding prealbumin), a retinol-binding protein. This protein is present in higher concentration than is TBG and primarily binds T4 with a Kd ~10–7 M. Albumin also can bind T4 when the more avid carriers are saturated, but its physiological importance is unclear. Binding of thyroid hormones to plasma proteins protects the hormones from metabolism and excretion, resulting in their long half-lives in the circulation. The free (unbound) hormone is a small percentage (~0.03% of T4 and ~0.3% of T3) of the total hormone in plasma. The differential binding affinities for serum proteins also contribute to establishing the 10- to 100-fold differences in circulating hormone concentrations and half-lives of T4 and T3.
Only the unbound hormone has metabolic activity. Because of the high degree of binding of thyroid hormones to plasma proteins, changes in either the concentrations of these proteins or the binding affinity of the hormones for the proteins has major effects on the total serum hormone levels. Certain drugs and a variety of pathological and physiological conditions can alter both the binding of thyroid hormones to plasma proteins and the amounts of these proteins (Table 39–1).
Table 39–1
Factors that Alter Binding of Thyroxine to Thyroxine-Binding Globulin
DEGRADATION AND EXCRETION. T3 and T4 can deiodinated but can also be metabolized by ether cleavage, conjugation, and oxidative decarboxylation.
T4 is eliminated slowly from the body, with a t1/2 of 6-8 days. In hyperthyroidism, the t1/2 is shortened to 3-4 days, whereas in hypothyroidism it may be 9-10 days. In conditions associated with increased binding to TBG, such as pregnancy, clearance is retarded. The opposite effect is observed when binding to protein is inhibited by certain drugs (see Table 39–1). T3, which is less avidly bound to protein, has a t1/2 of 1 day.
The liver is the major site of non-deiodinative degradation of thyroid hormones; T4 and T3 are conjugated with glucuronic and sulfuric acids and excreted in the bile. Some thyroid hormone is liberated by hydrolysis of the conjugates in the intestine and reabsorbed. A portion of the conjugated material reaches the colon unchanged, where it is hydrolyzed and eliminated in feces as the free compounds.
FACTORS REGULATING OF THYROID HORMONE SECRETION. Thyrotropin or TSH is a glycoprotein hormone with α and β subunits analogous to those of the gonadotropins (see Table 38–2). TSH is secreted in a pulsatile manner and circadian pattern (levels are highest during sleep at night). TSH secretion is controlled by the hypothalamic peptide thyrotropin-releasing hormone (TRH) and by the concentration of free thyroid hormones in the circulation. Extra thyroid hormone inhibits transcription of both the TRH gene and the genes encoding the α and β subunits of TSH, which suppresses the secretion of TSH and causes the thyroid to become inactive and regress. Any decrease in the normal rate of thyroid hormone secretion by the thyroid evokes an enhanced secretion of TSH. Additional mechanisms mediating the effect of thyroid hormone on TSH secretion appear to be a reduction in TRH secretion by the hypothalamus and a reduction in the number of TRH receptors on pituitary cells (Figure 39–4).
Figure 39–4 Regulation of thyroid hormone secretion. Myriad neural inputs influence hypothalamic secretion of thyrotropin-releasing hormone (TRH). TRH stimulates release of thyrotropin (TSH, thyroid-stimulating hormone) from the anterior pituitary; TSH stimulates the synthesis and release of the thyroid hormones T3 and T4. T3 and T4 feed back to inhibit the synthesis and release of TRH and TSH. Somatostatin (SST) can inhibit TRH action, as can dopamine and high concentrations of glucocorticoids. Low levels of I– are required for thyroxine synthesis, but high levels inhibit thyroxin synthesis and release.
THYROTROPIN-RELEASING HORMONE. TRH is a tripeptide (L-pyroglutamyl-L-histidyl-L-proline amide) synthesized by the hypothalamus and released into the hypophyseal-portal circulation where it interacts with TRH receptors on thyrotropes in the anterior pituitary. The binding of TRH to its receptor, a GPCR, stimulates the Gq-PLC-IP3-Ca2+ pathway and activates PKC, ultimately stimulating the synthesis and release of TSH. Two TRH receptors have now been identified, TRH-R1 and TRH-R2, as well as selective analogs for these receptors. Somatostatin, dopamine, and glucocorticoids inhibit TRH-stimulated TSH secretion.
ACTIONS OF TSH ON THE THYROID. TSH increases the synthesis and secretion of thyroid hormone. These effects follow the binding of TSH to its receptor (a GPCR) on the plasma membrane of thyroid cells. Binding of TSH to its receptor stimulates the Gs-adenylyl cyclase–cyclic AMP pathway. Higher concentrations of TSH activate the Gq-PLC pathway. Multiple mutations of the TSH receptor result in clinical thyroid dysfunction.
IODINE AND THYROID FUNCTION. Normal thyroid function requires an adequate intake of iodine; without it, normal amounts of hormone cannot be made, TSH is secreted in excess, and the thyroid becomes hyperplastic and hypertrophic. The enlarged and stimulated thyroid becomes remarkably efficient at extracting the residual traces of iodide from the blood, developing an iodine gradient that may be 10 times normal; in mild-to-moderate iodine deficiency, the thyroid usually succeeds in producing sufficient hormone and preferentially secreting T3. In more severe iodine deficiency, adult hypothyroidism and cretinism may occur. High levels of iodine inhibit thyroxine synthesis and release. In some areas of the world, simple or nontoxic goiter is prevalent because of insufficient dietary iodine. The addition of iodate to table salt (NaCl) provides a convenient iodine supplement. In the U.S., iodized salt provides 100 μg of iodine per gram. The recommended daily allowances for iodine range from 90-120 μg for children, 150 μg for adults, 220 μg for pregnancy, and 290 μg for lactation. Vegetables, meat, and poultry contain minimal amounts of iodine, whereas dairy products and fish are relatively high in iodine.
THYROID HORMONE TRANSPORT. Transmembrane passage of thyroid hormones appears to be mediated by monocarboxylic acid transporter 8 (MCT8, SLC16A2; see Table 5–2). MCT8 is widely expressed, including in liver, heart, and brain. MCT10 also transports T4 and T3 and is widely expressed, but its physiological importance for thyroid hormone transport in vivo is unknown. The organic anion transporter OATP1C1 preferentially transports T4 rather than T3, is highly expressed in brain capillaries, and has been hypothesized to be responsible for the transport of T4 across the blood-brain barrier (see Chapter 5).
MEDIATION OF EFFECTS BY NUCLEAR RECEPTORS. Thyroid hormone action is mediated largely by the binding of T3 to TRs, which are members of the nuclear receptor superfamily of transcription factors.
T3 binds to TRs with ~10-fold greater affinity than does T4, and T4 is not thought to be biologically active in normal physiology. TRs bind to specific DNA sequences (thyroid hormone response elements [TREs]) in the promoter/regulatory regions of target genes. The transcription of most target genes is repressed by unliganded TRs and induced following the binding of T3. In the unliganded state, the TR ligand-binding domain interacts with a corepressor complex that includes histone deacetylases and other proteins. The binding of T3 causes replacement of the corepressor complex by a coactivator complex that includes histone acetyltransferases, methyltransferases, and other proteins. Other thyroid hormone target genes, such as those encoding TRH and the TSH subunits, are negatively regulated by T3. The mechanism is not well defined, but these genes tend to be induced by the unliganded TR in addition to being repressed by T3.
Two genes encode TRs: THRA and THRB. THRA encodes the receptor TRα1. TRα1 is expressed in most cell types, but its major activities are in the regulation of heart rate, body temperature, skeletal muscle function, and the development of bone and small intestine. Alternative splicing of the TRα primary transcript results in the production of TRα2, which lacks part of the ligand-binding domain (LBD), does not bind T3, and has not known function. A cryptic promoter within intron 7 of THRA drives the production of small proteins that contain only a portion of the TRα LBD and appear to play a role, along with TRα1, in GI development. The THRB gene has 2 promoters that lead to the production of TRβ1 and TRβ2. These receptors have unique amino terminal domains but otherwise are identical. TRβ1 is ubiquitous; TRβ2 has a highly restricted pattern of expression. Mutations in THRB cause the syndrome of resistance to thyroid hormone. TRβ1 mediates specific effects in liver metabolism (including the hypocholesterolemic effect of T3); TRβ2 has roles in the negative feedback by T3 on hypothalamic TRH and pituitary TSH and in the development of retinal cones and the inner ear.
NONGENOMIC EFFECTS OF THYROID HORMONE. TRs are found outside the nucleus where they can exert biological effects via rapid nongenomic mechanisms.
TRs associate in a T3
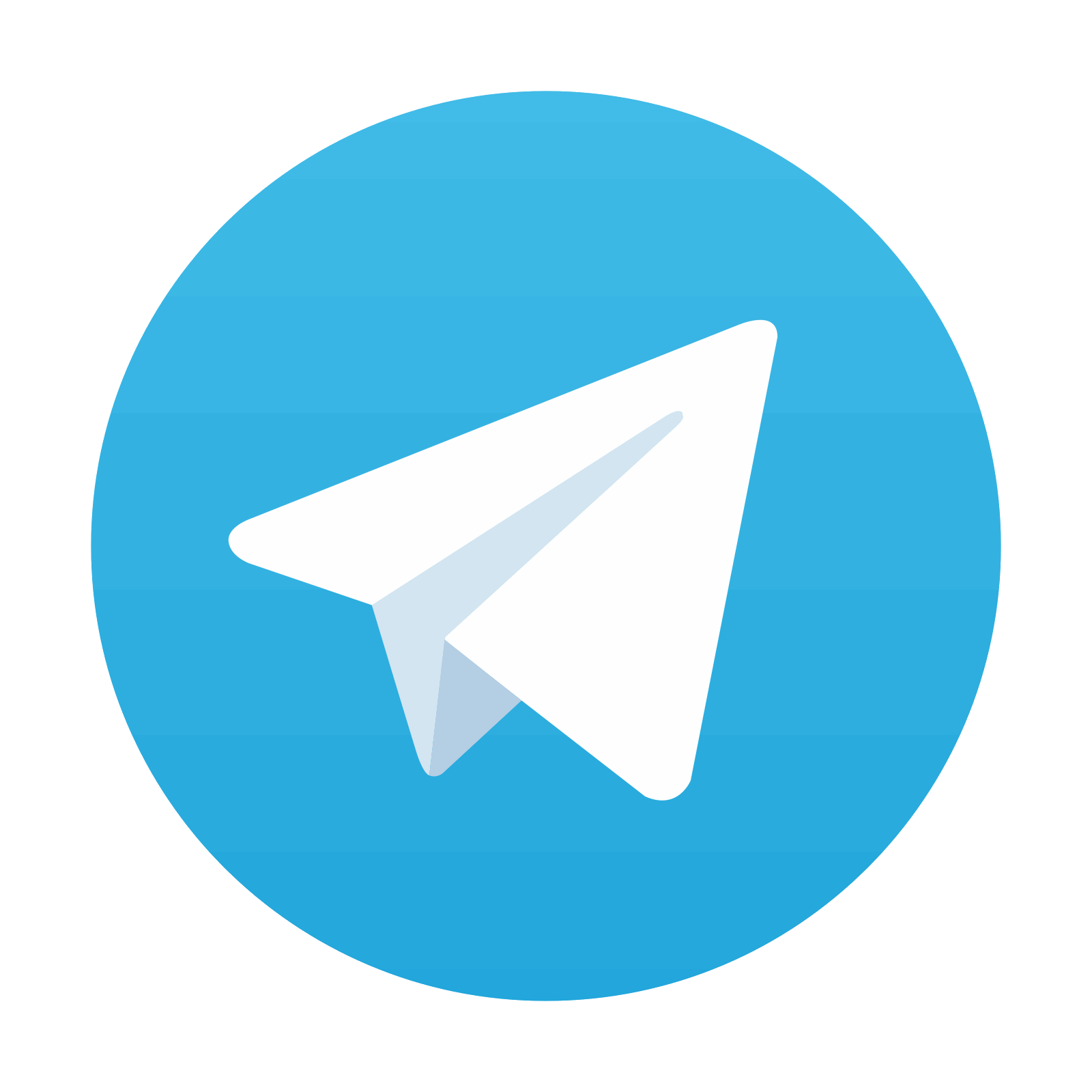
Stay updated, free articles. Join our Telegram channel

Full access? Get Clinical Tree
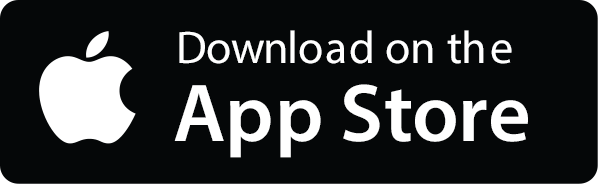
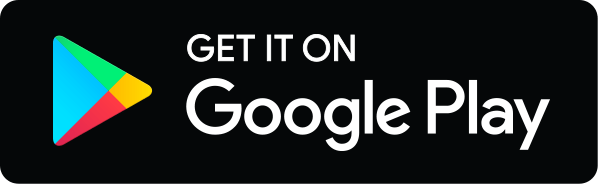