Fig. 4.1
Comparison of acute and chronic graft-versus-host disease (GVHD)
3.1 Pathophysiology of GVHD
Physiologic causes and effects of acute GVHD have been well characterized (Fig. 4.2) [18, 21, 24]. The complete understanding of this multistage process allows for ease in identification of novel therapeutic targets.


Fig. 4.2
Pathophysiology of GVHD. Phase I. Prior to transplantation the patient undergoes conditioning (radiation and/or chemotherapy) which leads to damage and activation of host tissue. Release of proinflammatory cytokines from activated host tissues increases HLA expression on host cells and enhances their antigen-presenting ability. Phase II. Transplanted donor T cells are activated upon interaction with host antigen resulting in proliferation and differentiation. Cytotoxic T cells produce additional inflammatory cytokines. Phase III. Cytotoxic T cells and cytokines act to damage host tissue
3.2 Stage 1: Host Tissue Damage and APC Activation
To prepare for transplantation, recipients must undergo a conditioning regimen involving immunosuppressive therapy to ablate the host immune response and allow for donor HSC engraftment. This high-dose regimen most often consists of chemotherapy and radiation therapy that causes tissue injury and results in inflammatory cytokine release including tumor necrosis factor alpha (TNF-α) and interleukin (IL)-12 [25–27]. Although critical to achieve successful engraftment, the creation of an inflammatory environment by the conditioning regimen has the potential to start the GVHD process. It is this initial release of proinflammatory cytokines brought about by tissue damage from the conditioning regimen in the recipient that is thought to underlie the initiation of acute GVHD [28]. The inflammatory cytokine release initiates an immune response cascade and results in recipient antigen presenting cell (APC) activation, upregulated MHC and costimulatory molecule expression and enhanced antigen presentation [20].
3.3 Stage 2: Donor T Cell Activation
After transplantation, donor T cells enter the inflammatory environment and recognize alloantigens presented by APCs. This recognition activates donor T cells causing them to expand, produce additional inflammatory cytokines including interferon gamma (IFN-γ) and recruit more immune cells to mount an attack against the host [27]. Donor T cell inflammatory cytokine release triggers a proinflammatory cytokine cascade, which further drives donor T cell proliferation. Tissue damage caused by donor T cells creates a cytotoxic environment and leads to recruitment of additional effector cells including NK cells and neutrophils, which leads to additional damage and immune cell recruitment, creating a cycle that is difficult to break.
3.4 Stage 3: Cytokine and Cell Mediated Tissue Damage
Finally, activated immune cells mediate the destruction of host tissues and result in multiple organ GVHD. Inflammatory cytokines enhance the cytotoxic effect of T cells in GVHD by attracting additional immune cells and by directly damaging the target tissue [29]. GVHD associated tissue damage is brought on by an influx of graft immune cells that includes T cells and NK cells and results in a dramatic increase of inflammatory cytokines including TNF-α, IFN-γ and reactive oxygen species [30]. Cytotoxic T cells and NK cells respond and stimulate monocytes to produce IL-1 and TNF-α, directly resulting in tissue damage. TNF-α acts by activating host APCs, which leads to enhanced antigen presentation to donor T cells [31]. Active tissue damage causes release of additional proinflammatory cytokines and perpetuates the cascade.
It is clear that proinflammatory cytokines play a critical role in initiation and perpetuation of the GVHD. However, anti-inflammatory cytokines including IL-10, IL-1 receptor antagonist (IL-1ra) and transforming growth factor beta (TGF-β) may also play a role in GVHD pathogenesis. Evidence suggests that increased expression of IL-10 leads to a decreased risk of GVHD development following allogeneic transplantation [32]. TGF-β has also been shown to mediate the immune response and has been shown to diminish acute GVHD [33] but may lead to chronic GVHD [34].
3.5 Current Therapeutics for GVHD
Current treatment for GVHD is based on high-dose corticosteroids for global immunosuppression. However, this therapy is not without significant drawbacks. Although it works to decrease GVHD, corticosteroid treatment also increases the risk of infection and relapse, major causes of death following HSCT [1]. In addition, a subset of GVHD termed steroid-refractory GVHD does not respond to conventional corticosteroid therapy [35]. The relatively complete understanding of the initiation and perpetuation of GVHD allows for identification of new pathways that may be exploited to prevent or treat GVHD.
4 Cell-Based Immunomodulation for Prevention and Treatment of GVHD
Cell-based therapeutics are of particular interest in the treatment of GVHD. As described, the initiation and perpetuation of GVHD is a very involved process and includes many different cells types and mediators at various stages. It is the complexity of this system that makes the development of specific pharmacological interventions challenging. Cells have the unique ability to respond to the environment around them and can produce varying types and amounts of trophic factors depending on the circumstance. The ability to adjust based on the situation makes cells an excellent therapeutic option for intricate processes such as GVHD.
4.1 Bone Marrow Mesenchymal Stromal Cells
A promising cell-based immunomodulatory source that is currently being examined in clinical trials of GVHD is MSCs [36, 37]. MSCs are most often derived from bone marrow (BM-MSC) or adipose tissue and can give rise to differentiated cells of the mesodermal lineage including bone, fat, cartilage, tendon, and muscle [38–40] MSCs have a proven ability to evade detection by the immune system after transplantation and suppress the immune response [41, 42]. MSCs have become an increasingly promising form of cellular therapy for a range of inflammatory and immune disorders including GVHD due to their ability to produce significant amounts of trophic factors, ability to modulate the activity of immune cells, and predisposition to home to sites of injury [42, 43].
The immunophenotype of MSCs has been well characterized and they exhibit low levels of HLA-I along with complete absence of HLA-II and costimulatory molecules, a profile that suggests potential immune privilege [44]. BM-MSCs are able to suppress T cell proliferation and activation in response to allogeneic activation or chemical stimulation in vitro and in vivo [5, 45, 46]. Immunoregulation by BM-MSCs is thought to result from both direct interactions between the stromal and immune cells [47–49] and the actions of anti-inflammatory soluble factors released by the stromal cells [50, 51]. The secretion of these factors occurs in response to proinflammatory signals from the local environment, including IFN-γ, TNF-α, IL-1α, and IL-1β [52–54].
Clinical applications for which the trophic action of MSCs may prove valuable include support of hematopoietic transplantation and the treatment of GVHD [55–58]. BM-MSCs display remarkable immunomodulatory properties and are able to mediate function of the major populations of immune cells. MSCs modulate the activation, proliferation, and function of effector and regulatory T cells [59]. In this capacity, MSCs are able to suppress the formation of cytotoxic T cells and to inhibit the expansion and inflammatory cytokine production of activated helper T cells [49, 51, 60, 61]. At the same time, MSCs have the ability to induce expansion of protective regulatory T cells [62]. MSCs inhibit B cell proliferation, differentiation toward plasma cells and antibody production [63]. In addition, MSCs suppress cytokine driven NK cell proliferation and inhibit their ability to produce the proinflammatory cytokine IFN-γ [64]. Finally, MSCs affect APC populations by reducing the formation and maturation of DCs [65, 66], reducing costimulatory molecule expression [67] on DCs and altering cytokine production [68].
The first trial to show the therapeutic effect of immunomodulatory BM-MSCs was in the accelerated recovery in patients with acute GVHD [58]. In addition, MSCs have proven to be effective in enhancing engraftment and reducing steroid-resistant GVHD after HSCT [58, 69, 70]. Possible mechanisms of MSC-mediated immunomodulation in the successful treatment of GVHD include reducing generation and maturation of DCs increasing regulatory T cell production by producing TGF-β, promoting generation of regulatory DCs which produce IL-10 and suppressing effector T cells by release of growth factors, inducible nitric oxide synthase (iNOS), heme oxygenase (HO)-1, prostaglandin or IDO [68]. Soluble factors are able to suppress the activity of immune cells by inhibiting their proliferation [71], decreasing their proinflammatory cytokine production [63], and conversion toward an anti-inflammatory phenotype [72]. MSCs may also act by downregulating immunoglobulin production by B cells and by inhibiting natural killer (NK) cell cytotoxicity and proliferation [18, 63].
The combination of regenerative and immunomodulatory properties in MSCs may prove beneficial in tissue and organ transplantation. While these results show great promise in the treatment of GVHD, MSCs have several considerable downfalls that must be addressed prior to widespread clinical use including significant amounts of donor-to-donor heterogeneity in immunomodulatory function and their relatively limited proliferation ability under standard culture conditions.
5 AFS Cells for Treatment of GVHD
Human amniotic fluid stem (AFS) cells are a population of well-characterized, broadly multipotent fetal-derived stem cells [7]. AFS cells share many characteristics with MSCs including surface marker profile and lineages to which they most easily differentiate. However, AFS cells have several distinct advantages over MSCs for clinical use.
c-kit-selected AFS cells are a particularly attractive therapeutic cell source because of their extensive capacity for self-renewal, their broad differentiation capabilities, immunomodulatory potential, and lack of teratoma formation [7]. Clonal lines of AFS cells have been shown to expand extensively in culture (greater than 80 population doublings) and without a change in phenotype [7]. In addition, AFS cells have been shown to express markers associated with pluripotency (Oct4 and SSEA4) as well as markers of adult stem cells (CD29, CD44, CD73, CD90, and CD105) [7]. AFS cells also have the ability to differentiate into cell types representative of each of the three germ layers including adipogenic, osteogenic, myogenic, endothelial, neurogenic, and hepatic cells [7]. AFS cells have been shown to possess immunomodulatory capabilities both in vitro and in vivo [73–75]. The combination of these properties makes AFS cells and ideally suited cell source to be used in the prevention and treatment of GVHD.
5.1 AFS Cells Evade Immune Recognition
The immunomodulatory capabilities of AFS cells are of great interest because these cells are derived from an immunoprivileged site and are able to evade recognition and destruction by the maternal immune system [76].The fetus represents a site of immune privilege where there is a complete lack of maternal immune response against fetal tissues expressing paternally inherited alloantigens. Fetal-derived AFS cells do not express surface markers associated with rejection and therefore are likely able to evade immune recognition [73]. The HLA molecules are a critical part of the immune response and while AFS cells demonstrate HLA-I expression they show a complete lack of expression of HLA-II and costimulatory molecules (CD80, CD86, CD40) [7, 73]. Stimulation with IFN-γ upregulated expression of MHC Class I and induced expression MHC Class II [73]. The immunophenotype of AFS cells is very similar to that of BM-MSCs and indicates that they may not strongly activate immune responses in allogeneic hosts. The ability to evade the allogeneic immune recognition is critical for a cell-based therapeutic to be used in GVHD.
5.2 AFS Cells and AFS Derived Cell-Free Supernatant Suppress T cell Activation
In allogeneic transplantation resulting in GVHD, T cells are activated upon exposure to nonself HLA molecules and to foreign antigens [66]. In turn, T cell activation results in proliferation, maturation, and cytokine secretion, which induces effector cell function and leads to host tissue destruction [65]. One of the goals of GVHD therapeutics is to inhibit T cell activation and block effector cell function, which would result in decreased tissue destruction. Activated T cells produce the proinflammatory cytokine IFN-γ, among others [68]. In vitro culture systems can stimulate T cells and use IFN-γ production as a measure for the amount of T cell activation [67]. AFS cells are able to inhibit in vitro T cell activation in a dose-dependent manner to levels comparable to that of BM-MSCs [73]. These studies prove that cell-to-cell contact between AFS cells and immune cells results in inhibition of the in vitro immune response as measured by IFN-γ production of activated T cells [73]. Suppression of T cell activation is a critical factor for the treatment of GVHD because it is the activated, cytotoxic T cells that induce injury. Blocking this activation process may lead to reduced inflammatory cytokine release and a reduction in host tissue damage.
In addition, AFS cells have been shown to secrete cytokines that are able to inhibit T cell activation in vitro. Cell-free supernatants derived from AFS cells under normal expansion conditions are unable to significantly inhibit T cell activation. However, it is known that immunomodulatory cell sources, including BM-MSCs, must be exposed to proinflammatory stimuli to evoke production of protective, anti-inflammatory cytokines [52]. AFS cells activated by co-culture with either total peripheral blood mononuclear cells (PBMCs), meant to mimic exposure to the immune system or IL-1β, a proinflammatory cytokine released by immune cells, release immunomodulatory factors that reduce T cell activation and IFN-γ production [73]. AFS cells respond to the inflammatory environment by producing immunomodulatory mediators capable of inhibiting the immune response. This is a factor critical in the treatment for GVHD because initiation and perpetuation of GVHD is dependent on proinflammatory cytokines including IL-1.
5.3 Cytokine Secretion of Activated AFS Cells
Cytokines are critical mediators of the immune response and elucidating the factors being produced by AFS cells is critical for their future clinical use in treatment of GVHD. Studies examining cytokines, chemokines, and growth factors reveal that proliferating AFS cells produce low levels of very few cytokines. However, upon activation with immune cells or proinflammatory cytokine IL-1, AFS cells produced highly elevated levels of GRO, GRO-α, monocyte chemotactic protein-1 (MCP-1), IL-2, IL-6, and granulocyte macrophage colony stimulating factor (GM-CSF), and lower levels of MIP-3α, IL-2, regulated upon activation, normal T cell expressed, and secreted (RANTES) and epithelial neutrophil-activating protein-78 (ENA-78). Importantly, these factors were also upregulated in stimulated BM-MSCs that had proven immunomodulatory capabilities [73]. However, BM-MSCs produced several additional cytokines that were not generated by AFS cells including monocyte chemotactic protein-2 (MCP-2), macrophage inflammatory protein 1-alpha and 3-alpha (MIP-1α, MIP-3α) and Activin revealing the potential for alternative molecular mechanisms of AFS cell immune response modulation.
The mechanisms involved in the inhibitory effect of AFS cells have not yet been completely elucidated. In response to activation, the most highly upregulated protein in AFS cells was interleukin 6 (IL-6). IL-6 is a broad-acting cytokine involved in the control of the immune response as well as stem cell development and regulation [55, 77]. Mesenchymal stem cells derived from cord blood (CB-MSCs) and BM-MSCs have also been reported to secrete high amounts of IL-6 when activated with IL-1β [78]. The biological relevance of this IL-6 response likely lies in both local and systemic protection against inflammation [69, 70]. AFS cells also released high levels of growth related oncogene family members GRO and GRO-α as well as MCP-1 upon IL-1β activation. These chemokines have well-known effects on cells of the immune system and are important in inflammation and wound healing. GRO acts on neutrophils and MCP-1 acts mainly on macrophages, recruiting them to sites of inflammation [79].
In conclusion, the mechanism of immunosuppression by AFS cells involves both direct cell–cell contact between the stem cells and the immune cells and indirect interaction through immunosuppressive factors secreted by the stem cells in response to proinflammatory cytokines [73]. A proposed model showing the immunosuppressive capabilities of AFS cells is shown in Fig. 4.3. These AFS-mediated immunomodulatory actions may prove beneficial in the treatment of GVHD.


Fig. 4.3
Model of proposed mechanism by which human amniotic fluid stem (AFS) cells interact within the confines of the immune response. Secretion of IL-1β and other cytokines from monocytes causes an activation of the immunodulatory properties in AFS cells. In response, AFS cells secrete a battery of soluble factors including IL-6, MCP-1 and others which inhibit T cell activation
5.4 In Vivo Evidence Suggesting a Role for AFS Cells in GVHD
AFS cells also possess immunomodulatory capabilities in experimental in vivo models of inflammation. Recent studies prove that rat AFS (rAFS) cells significantly improve survival and enhance repair of the damaged intestine in a rat experimental model of necrotizing enterocolitis (NEC) [80]. Similar results have been achieved using human AFS cells to repair the kidney and restore its function in an immunodeficient mouse model of acute tubular necrosis (ATN) [74]. In this model AFS cells appear to possess immunomodulatory function, initiating the release of several murine anti-inflammatory cytokines and reducing the release of proinflammatory cytokines [74].
Several studies have attributed favorable outcomes observed after transplantation of fetal-derived stem cells to the release of protective or reparative trophic factors by the stem cells. Indeed, introduction of cell-free conditioned medium from amniotic fluid has proven beneficial in restoring blood flow in injury models [81] and in stimulation of repair in wound models [82]. The mechanisms behind these effects remain under investigation but immunomodulatory capabilities of these cells may be involved in limiting damage and stimulating repair of injured tissue. Based on these studies we have developed a proposed model demonstrating the potential mechanism of action of AFS cells in treating GVHD (Fig. 4.4).


Fig. 4.4
Proposed mechanism of human AFS cells in the treatment of GVHD. Human AFS cells may act to inhibit T cell activation in Phase II of GVHD. Prevention of T cell activation may decrease the proliferation, differentiation, and inflammatory cytokine release of T cells, leading to a decrease in host tissue damage
6 Advantages of AFS Cells in the Treatment of GVHD
The main characteristic of AFS cells that makes them an ideal cell type for therapeutic applications in the treatment of GVHD is their proven immunomodulatory capacity [73, 74, 80]. However, AFS cells have additional features that make them an attractive therapeutic cell source including their ability to expand extensively in culture and their inability to form tumors when implanted into immune deficient mice.
AFS cells are able to proliferate extensively in culture without acquiring chromosomal abnormalities while maintaining their initial properties [7]. This is in stark contrast to MSCs which have such limited in vitro expansion capabilities that cells from several donors must be pooled to achieve one therapeutic dose [83]. The capacity for uninhibited growth in vitro is critical to allow for the extensive expansion and full characterization of clonally derived AFS cell lines with immunomodulatory capabilities. Not surprisingly, donor-to-donor variability has been revealed in the ability of MSC extracts to mediate the immune response [84]. The ability to fully define the potentially unique immunomodulatory characteristics of individual AFS cell lines is a huge benefit in GVHD treatment. Following expansion and characterization, each AFS cell line can be cryopreserved and banked for future use as a reproducible “off the shelf” cell-based therapy for the treatment of GVHD. A summary of the advantages of using AFS cells for the treatment of GVHD is found in Fig. 4.5.


Fig. 4.5
Advantages of using human AFS cells for prevention and treatment of GVHD
7 Future Considerations
The immunomodulatory effects of AFS cells that have been documented thus far are promising and suggest a role for these fetal-derived cells in the treatment and prevention of GVHD. However, it is critical to consider issues such as dosing of AFS cells and timing of the AFS cell transplantation before their clinical use is considered.
One important issue to address is the dose of AFS cell that will be required for optimal reduction of GVHD. In vitro assays demonstrated a dose-dependent T cell response to AFS cells and showed that even at the lowest dose tested (1 AFS cell to 32 T cells), AFS cells were able to inhibit T cell activation [73]. However, performing a similar dosing experiment in an in vivo model of GVHD to prove safety and efficacy of AFS cells for GVHD treatment is critical. Timing of AFS cell administration is another important consideration in their potential treatment of GVHD. It is likely that AFS cells would be infused at the time of allogeneic transplantation to prevent initiation of acute GVHD. However, another option to consider is waiting until the observation of GVHD symptoms before administration of AFS cells.
AFS cells also have the potential to serve as a tool for identification and isolation of soluble factors involved in immunomodulation that may be used in cell-free therapeutics for GVHD. Cell-free supernatant derived from IL-1β stimulated AFS cells has the ability to suppress T cell activation. In vitro characterization of the soluble factors secreted by AFS cells in response to stimulation with IL-1β was performed by protein array. Application of the factors identified including IL-6, MCP-1, GRO and GRO-α, individually or in combination, to systems of inflammation including GVHD would advance knowledge on the effects of these morphogens. Further characterization of the complex profile of soluble molecules secreted by AFS cells is critical and may lead to the development of novel strategies for direct application of these factors for therapeutic approaches.
In the future, it is critical to make additional efforts to classify the immune response to AFS cells in vitro and in vivo. It is known that AFS cells or cell-free supernatant are able to inhibit T cell activation as measured by IFN-γ release. However, T cells represent only one part of the immune response; many additional cell types and responses make up the complete immune system. Dendritic cells (DCs) are an APC type critical in the immune response. It is critical to examine the ability of AFS cells to inhibit of upregulation of costimulatory molecules and chemokine receptors and their ability to decrease in the formation of dendrites. B cells and natural killer (NK)-cells are also important in the immune response and it is important to examine the ability of AFS cells to inhibit their proliferation [63, 85]. Identification of immune cell/stem cell interactions and further characterizing the mechanisms of AFS-mediated immunomodulation is essential in determining their ideal therapeutic use.
8 Conclusions
GVHD is a major complication of allogeneic transplantation and primary cause of transplant-related mortality. Discovery and development of novel GVHD therapeutics is critical, especially for cases of steroid-refractory GVHD. Human AFS cells are an immunomodulatory cell source derived from an immunoprivileged site that can be expanded indefinitely in culture. AFS cell therapy may selectively inhibit the immune response in GVHD as opposed to the current global immunosuppressive medications. These properties make AFS cells an ideally suited cell source to be used in efforts to prevent and treat GVHD.
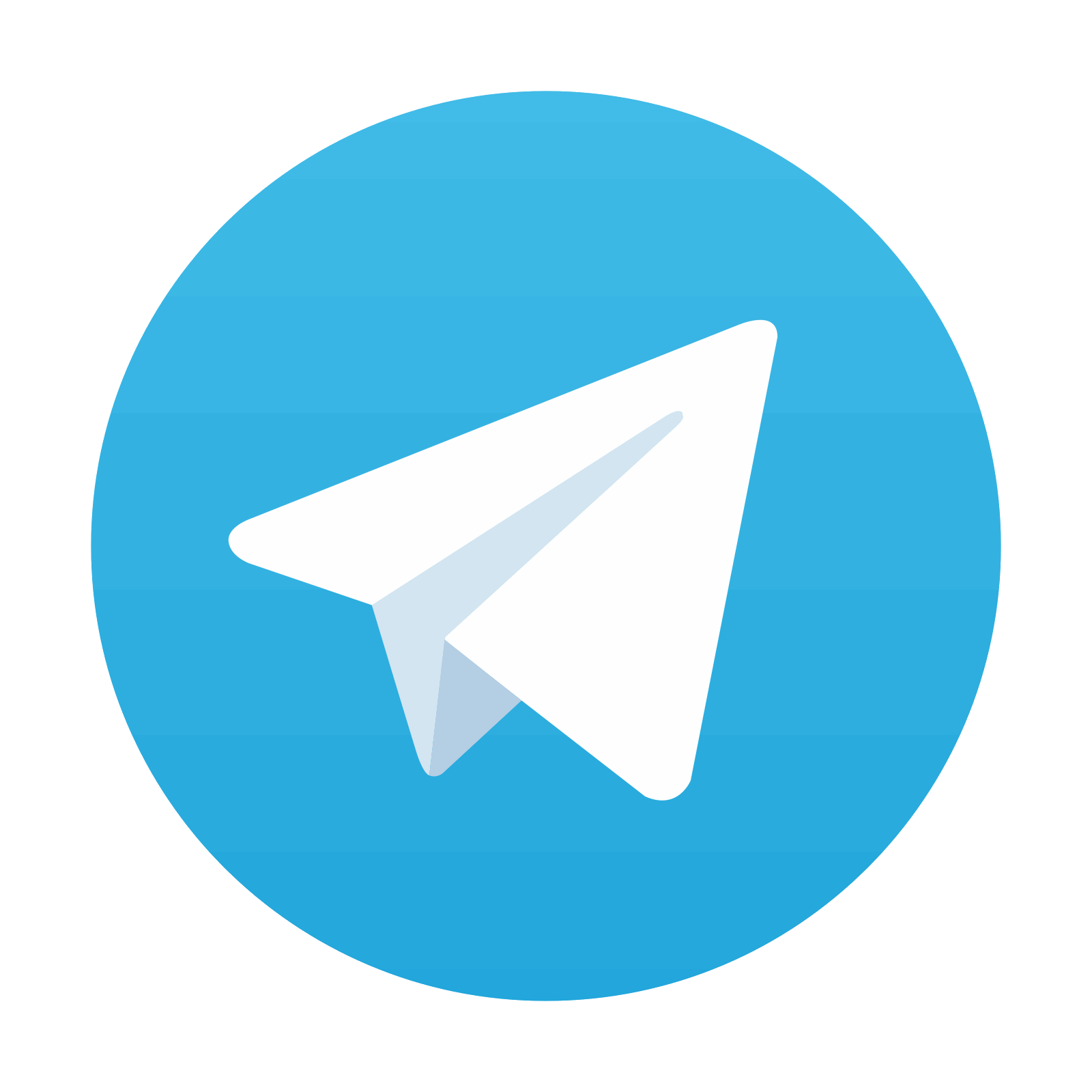
Stay updated, free articles. Join our Telegram channel

Full access? Get Clinical Tree
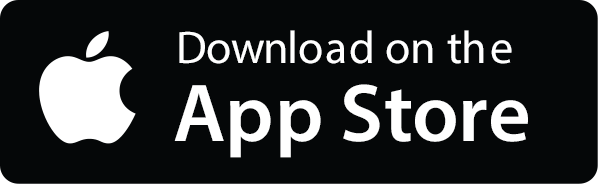
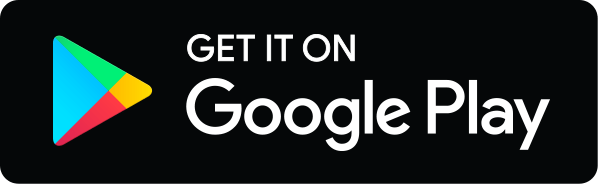