THE THYROID
The thyroid gland is responsible for the production of two hormones: thyroid hormone and calcitonin. Calcitonin is secreted by parafollicular C cells and is involved in calcium homeostasis. Thyroid hormone is critical in regulating body metabolism, neurologic development, and numerous other body functions. Clinically, conditions affecting thyroid hormone levels are much more common than those affecting calcitonin and are the major focus of this chapter.
Thyroid Anatomy and Development
The thyroid gland is positioned in the lower anterior neck and is shaped like a butterfly. It is made up of two lobes resting on each side of the trachea, bridged by the isthmus, with a band of thyroid tissue running anterior to the trachea. Posterior to the thyroid gland lie the parathyroid glands—which regulate serum calcium levels—and the recurrent laryngeal nerves innervating the vocal cords. The locations of these structures become important during thyroid surgery, when injury could lead to hypocalcemia or permanent hoarse voice.
The fetal thyroid develops from an outpouching of the foregut at the base of the tongue that migrates to its final location over the thyroid cartilage in the first 4 to 8 weeks of gestation. By week 11 of gestation, the thyroid gland begins to produce measurable amounts of thyroid hormone.1 Iodine is an essential component of thyroid hormone. In parts of the world where severe iodine deficiency exists, neither the mother nor the fetus can produce sufficient amounts of thyroid hormone and both develop hypothyroidism. As thyroid hormone is critical to fetal neurologic development, hypothyroidism can lead to mental retardation and cretinism. In areas where iodine deficiency is not an issue, other problems with thyroid development may occur, including congenital hypothyroidism, which occurs in 1 of 4,000 live births.2 If the mother has normal thyroid function, small amounts of maternal thyroid hormone crossing the placenta protect the fetus during development. Immediately postpartum, however, these newborns require initiation of thyroid hormone or their further neurologic development will be significantly impaired. In much of the developed world, screening tests are performed on newborns to detect congenital hypothyroidism.
Thyroid Hormone Synthesis
Thyroid hormone is made primarily of the trace element iodine, making iodine metabolism a key determinant in thyroid function.1 Iodine is found in seafood, dairy products, iodine-enriched breads, and vitamins. Significantly, iodine is used in high concentrations in the contrast medium used in many radiologic procedures, including computed tomography (CT) scans and heart catheterization. It is also present in amiodarone, a medication used to treat certain heart conditions. The recommended minimum daily intake of iodine is 150 μg, although most individuals in developed countries ingest far more than this amount. In the United States, the average adult daily intake of iodine has decreased since the 1970s but remains sufficient at an estimated 240 and 740 daily μg, well above the US Institute of Medicine’s recommended adult dietary allowance of 150 μg/day.3,4 If iodine intake drops below 50 μg daily, the thyroid gland is unable to manufacture adequate amounts of thyroid hormone, and thyroid hormone deficiency—hypothyroidism—results.5
Thyroid cells are organized into spheres surrounding a central core of fluid called colloid. These structures are called follicles. The major component of colloid, thyroglobulin, is a glycoprotein manufactured exclusively by thyroid follicular cells and rich in the amino acid tyrosine. Some of these tyrosyl residues will be iodinated, producing the building blocks of thyroid hormone. On the outer side of the follicle, iodine is actively transported into the thyroid cell by the Na+/I− symporter located on the basement membrane. Inside the thyroid cell, iodide diffuses across the cell to the apical side of the follicle, which abuts the core of colloid. Here, catalyzed by a membrane-bound enzyme called thyroid peroxidase (TPO), concentrated iodide is oxidized and bound with tyrosyl residues on thyroglobulin. This results in production of monoiodothyronine (MIT) and diiodothyronine (DIT). This same enzyme also aids in the coupling of two tyrosyl residues to form triiodothyronine (T3) (one MIT residue + one DIT residue) or thyroxine (T4) (two DIT residues). These are the two active forms of thyroid hormone. This thyroglobulin matrix, with branches now holding T4 and T3, is stored in the core of the thyroid follicle. Thyroid-stimulating hormone (TSH) signals the follicular cell to ingest a microscopic droplet of colloid by endocytosis. Inside the follicular cell, these droplets are digested by intracellular lysosomes into T4, T3, and other products. T4 and T3 are then secreted by the thyroid cell into the circulation (Fig. 23.1).
FIGURE 23.1 Biosynthesis of thyroid hormone. Thyroid hormone synthesis includes the following steps: (1) iodide (I−) trapping by thyroid follicular cells; (2) diffusion of iodide to the apex of the cell and transport into the colloid; (3) oxidation of inorganic iodide to iodine and incorporation of iodine into tyrosine residues within thyroglobulin molecules in the colloid; (4) combination of two diiodotyrosine (DIT) molecules to form tetraiodothyronine (thyroxine, T4) or of monoiodotyrosine (MIT) with DIT to form triiodothyronine (T3); (5) uptake of thyroglobulin from the colloid into the follicular cell by endocytosis, fusion of the thyroglobulin with a lysosome, and proteolysis and release of T4 and T3; and (6) release of T4 and T3 into the circulation.
Activity of thyroid hormone depends on the location and number of iodine atoms. Approximately 80% of T4 is metabolized into either T3 (35%) or reverse T3 (rT3) (45%). Outer-ring deiodination of T4 (5′-deiodination) leads to production of 3,5,3′-triiodothyronine (T3). T3 is three to eight times more metabolically active than is T4 and often considered to be the active form of thyroid hormone, with T4 considered the “pre”-hormone (with thyroglobulin being the “prohormone”). In addition to its “pre”-hormone activity, however, inner-ring deiodination of T4 results in the production of metabolically inactive rT3 (Fig. 23.2).
FIGURE 23.2 Metabolism of thyroxine.
There are three forms of iodothyronine 5′-deiodinase. Type 1 iodothyronine 5′-deiodinase, the most abundant form, found mostly in the liver and kidney, is the largest contributor to the circulating T3 pool. Certain drugs (e.g., propylthiouracil, glucocorticoids, and propranolol) slow the activity of this deiodinase and are used in the treatment of severe thyroid hormone excess, or hyperthyroidism. Type 2 iodothyronine 5′-deiodinase, found in the brain and pituitary gland, functions to maintain constant levels of T3 in the central nervous system. Its activity is decreased when levels of circulating T4 are high and increased when levels are low. Activity of the deiodination enzymes gives another level of control of thyroid hormone activity beyond the thyrotropin-releasing hormone (TRH) and thyrotropin (TSH) control of the hypothalamic–pituitary–thyroid axis1 (Fig. 23.2).
Protein Binding of Thyroid Hormone
When released into the circulation, only 0.04% of T4 and 0.4% of T3 are unbound by proteins and available for hormonal activity. The three major binding proteins, in order of significance, are thyroxine-binding globulin (TBG), thyroxine-binding prealbumin (TBPA), and albumin. Alterations to the concentrations of binding proteins significantly affect circulating quantities of T4 and T3. For example, high estrogen levels during pregnancy lead to increased TBG production by the liver, which results in higher levels of bound thyroid hormones, leading to high levels of total T3 and total T4. Typically, however, levels of the unbound active, or free, thyroid hormones remain in the normal range and the individual remains euthyroid. In some instances, however, measurement of free T4 and free T3 may be necessary to eliminate any confusion caused by abnormal binding protein levels.
Control of Thyroid Function
Understanding of the hypothalamic–pituitary–thyroid axis is essential for correctly interpreting thyroid function testing. This axis is central to the regulation of thyroid hormone production. TRH is synthesized by neurons in the supraoptic and supraventricular nuclei of the hypothalamus and stored in the median eminence of the hypothalamus. When secreted, this hormone stimulates cells in the anterior pituitary gland to manufacture and release TSH. TSH, in turn, circulates to the thyroid gland and leads to increased production and release of thyroid hormone. When the hypothalamus and pituitary sense that there is an inadequate amount of thyroid hormone in circulation, TRH and TSH secretion increases and stimulates increased thyroid hormone production. If thyroid hormone levels are high, TRH and TSH release will be inhibited, leading to lower levels of thyroid hormone production. This feedback loop requires a normally functioning hypothalamus, pituitary, and thyroid gland, as well as an absence of any interfering agents or agents that mimic TSH action (Fig. 23.3).
FIGURE 23.3 Hypothalamic–pituitary–thyroid axis. Thyrotropin-releasing hormone (TRH) stimulates the production and release of thyrotropin (TSH). TSH stimulates the thyroid gland to synthesize and secrete thyroid hormone. T4 that is released by the thyroid gland is mostly converted to T3 by the liver and kidney. T3 and T4 feedback inhibit TSH release directly through action at the pituitary and indirectly by decreasing TRH release from the hypothalamus. (Adapted from Surks MI, Sievert R. Drugs and thyroid function. N Engl J Med. 1995;333:1688.)
Actions of Thyroid Hormone
Once released from the thyroid gland, thyroid hormone circulates in the bloodstream where free T4 and T3 are available to travel across the cell membrane. In the cytoplasm, T4 is deiodinated into T3, the active form of thyroid hormone. T3 combines with its nuclear receptor on thyroid hormone-responsive genes, leading to production of messenger RNA that, in turn, leads to production of proteins that influence metabolism and development. Effects of thyroid hormone include tissue growth, brain maturation, increased heat production, increased oxygen consumption, and increased expression of β-adrenergic receptors. Clinically, individuals who have excess thyroid hormone (thyrotoxicosis) will have symptoms of increased metabolic activity such as tachycardia and tremor, while individuals with hypothyroidism note symptoms of lowered metabolic activity like edema and constipation.
TESTS FOR THYROID FUNCTION
Blood Tests
Thyroid-Stimulating Hormone
The most useful test for assessing thyroid function is the TSH, currently in its third generation. All the assays are capable of diagnosing primary hypothyroidism (thyroid gland disease leading to low thyroid hormone production) with elevated levels of TSH. Second-generation TSH immunometric assays, with detection limits of 0.1 mU/L, effectively screen for hyperthyroidism, but the third-generation TSH chemiluminometric assays, with increased sensitivity to detection limits of 0.01 mU/L, give fewer false-negative results and more accurately distinguish between euthyroidism and hyperthyroidism. Although a fourth-generation assay exists, it is used for research purposes and the third-generation TSH assays are the preferred method for monitoring and adjusting thyroid hormone replacement therapy and screening for abnormal thyroid hormone production in the clinical setting.6
The sensitivity of the third-generation TSH assays led to the ability to detect what is termed subclinical disease—or a mild degree of thyroid dysfunction—due to the large reciprocal change in TSH levels seen for even small changes in free T4.7 In subclinical hypothyroidism, the TSH is minimally increased while the free T4 stays within the normal range. Likewise, in subclinical hyperthyroidism, the TSH is low while the free T4 is normal (Table 23.1). The clinical significance of subclinical disease and thresholds for treatment remain somewhat unclear and remain an ongoing topic of investigation.
TABLE 23.1 Interpretation of Thyroid Tests
Serum T4 and T3
Serum total T4 and T3 levels are usually measured by radioimmunoassay (RIA), chemiluminometric assay, or similar immunometric technique. As previously mentioned, because more than 99.9% of thyroid hormone is protein bound, alteration in thyroid hormone–binding proteins frequently leads to total T4 and T3 levels outside of the normal range without representing true clinical thyroid dysfunction. Because of this, assays to measure free T4 and T3, the biologically active hormone forms, were developed. At least partially because of lower processing costs and ease of interpretation, free T4 kits now replace total T4 assessment at the clinical level.8 Currently available assay kits for measuring free T4 levels are not error proof, though, and can still be affected by some binding protein abnormalities.9 When this is suspected, measurement of free T4 levels is performed by dialysis. Kits that estimate free T3 levels have theoretical advantages; however, actual clinical utility is yet to be clearly defined.
Thyroglobulin
Thyroglobulin is a protein synthesized and secreted exclusively by thyroid follicular cells. This prohormone in the circulation is proof of the presence of thyroid tissue. This fact makes thyroglobulin an ideal tumor marker thyroid cancer posttreatment surveillance as patients with well-differentiated thyroid cancer successfully treated with surgery and radioactive iodine ablation should have undetectable thyroglobulin levels.
Thyroglobulin is currently measured by double-antibody RIA, enzyme-linked immunoassay (ELISA), immunoradiometric assay (IRMA), and immunochemiluminescent assay (ICMA) methods. The accuracy of the thyroglobulin assay is primarily dependent on the specificity of the antibody used and the absence of antithyroglobulin autoantibodies. Even with modern assays, antithyroglobulin autoantibodies interfere with measurements and lead to unreliable thyroglobulin results. For this reason, it is critically important to screen for autoantibodies whenever thyroglobulin is measured. If antibodies are present, the value of the thyroglobulin assay is marginal. Approximately 25% of patients with well-differentiated thyroid cancer have antithyroglobulin autoantibodies, compared to 10% of the general population. If a patient with well-differentiated thyroid cancer and antithyroglobulin autoantibodies has been successfully treated with surgery and radioactive iodine ablation, autoantibodies should disappear over time.6
Thyroid Autoimmunity
Many diseases of the thyroid gland are related to autoimmune processes. In autoimmune thyroid disease, antibodies are directed at thyroid tissue with variable responses. The most common cause of hyperthyroidism is an autoimmune disorder called Graves’ disease. The antibodies in this condition are directed at the TSH receptor (TSHR), stimulating the receptor and leading to growth of the thyroid gland and production of excessive amounts of thyroid hormone. This condition can be diagnosed with tests that detect TSHR-stimulating antibodies. Two types of assays exist to detect an autoimmune etiology to hyperthyroidism: competition-based assays that detect TSH receptor antibodies (TRAb) based upon their ability to compete for TSHR with a known ligand and thyroid-stimulating immunoglobulin (TSI) assays detecting cAMP production in patients’ sera. Tests for TSH receptor antibodies (TRAb, TSHRAb) can detect antibodies directed against the TSHR whether they act to stimulate or block the TSHR. Both stimulating and blocking antibody assays will be positive in 70% to 100% of patients with Graves’ disease.10 Chronic lymphocytic thyroiditis—commonly known as Hashimoto’s thyroiditis—is at the other end of the autoimmune continuum. In this condition, antibodies lead to decreased thyroid hormone production by destruction of the thyroid gland, which is the most common cause of hypothyroidism in the developed world. The best test for this condition is the TPO antibody, which is present in 10% to 15% of the general population and 80% to 99% of patients with autoimmune hypothyroidism (Table 23.2).
TABLE 23.2 Prevalence of Thyroid Autoantibodies
OTHER TOOLS FOR THYROID EVALUATION
Nuclear Medicine Evaluation
Radioactive iodine is useful in assessing the metabolic activity of thyroid tissue and assisting in the evaluation and treatment of thyroid cancer. When radioactive iodine is given orally, a percentage of the dose is taken up by the thyroid gland. This percentage is called the radioactive iodine uptake (RAIU). High uptake suggests that the gland is metabolically active and producing significant amounts of thyroid hormone. Low uptake suggests that the gland is metabolically inactive. Because TSH stimulates iodine uptake by the thyroid gland, it is important to interpret the scan in conjunction with an assessment of TSH levels. An undetectably low TSH should turn off the thyroid gland’s uptake of iodine. If the uptake is normal or high despite an undetectably low TSH, the thyroid must be either acting autonomously without regard to the hypothalamus–pituitary–thyroid feedback system or through a TSH surrogate. Such is the case with Graves’ disease, where an immunoglobulin activates the TSHR on the thyroid gland, leading to high rates of thyroid hormone production and a high RAIU. The high level of thyroid hormone in the circulation feeds back on the pituitary and hypothalamus, turning off TSH, but this has no effect on the levels of TSI (the TSH surrogate). Conversely, if the RAIU is low in the presences of an undetectably low TSH, the differential diagnosis includes excess exogenous thyroid hormone ingestion, high iodine intake, or a condition in which stored thyroid hormone is leaking from the thyroid gland (typically in a setting known as subacute thyroiditis).
Radioactive iodine can also be useful in the evaluation of thyroid nodules in the presence of a low or undetectable TSH. Thyroid nodules that take up significant amounts of radioactive iodine on thyroid scans—termed “hot” nodules—are statistically unlikely to be thyroid cancer. The converse, however, does not hold true as nodules that show little or no radioiodine uptake—indeterminate (“warm”) or “cold” nodules, respectively—may be cancerous, but the majority of such nodules are benign.
Thyroid Ultrasound
The significance of thyroid ultrasound in the assessment of thyroid anatomy and characterization of palpable thyroid abnormalities has progressively increased in the last several years. Thyroid ultrasounds are capable of detecting even thyroid nodules of such a small size as to be of unclear or even no clinical significance; depending upon age, in up to 50% of clinically normal thyroid glands, small (<1 cm) thyroid nodules can be seen.11
Fine-Needle Aspiration
Thyroid fine-needle aspiration (FNA) biopsy is often the first step and most accurate tool in the evaluation of thyroid nodules in the absence of hyperthyroidism. The routine use of FNA allows prompt identification and treatment of thyroid malignancies and avoids unnecessary surgery in most individuals with benign thyroid lesions. In this procedure, a small-gauge needle is inserted into the nodule and cells are aspirated for cytologic evaluation. The procedure can be performed using palpation if the nodule is palpable, but is becoming more commonly used with the assistance of ultrasound imaging. FNA biopsy results are reported according to the Bethesda System for Reporting Thyroid Cytopathology as falling into one of six categories: nondiagnostic/unsatisfactory, benign, atypia/follicular lesion of undetermined significance, follicular neoplasm/suspicious for follicular neoplasm, suspicious for malignancy, and malignant. These categories dictate subsequent treatment, ranging from routine ultrasound monitoring to surgical excision.12,13
CASE STUDY 23.1
A 24-year-old woman presents 2 months postpartum with symptoms of hyperthyroidism. She does not have evidence of Graves’ ophthalmopathy. Her TSH level is undetectable, and free T4 is two times the upper limit of normal.
questions
1. What are possible causes for her thyrotoxicosis?
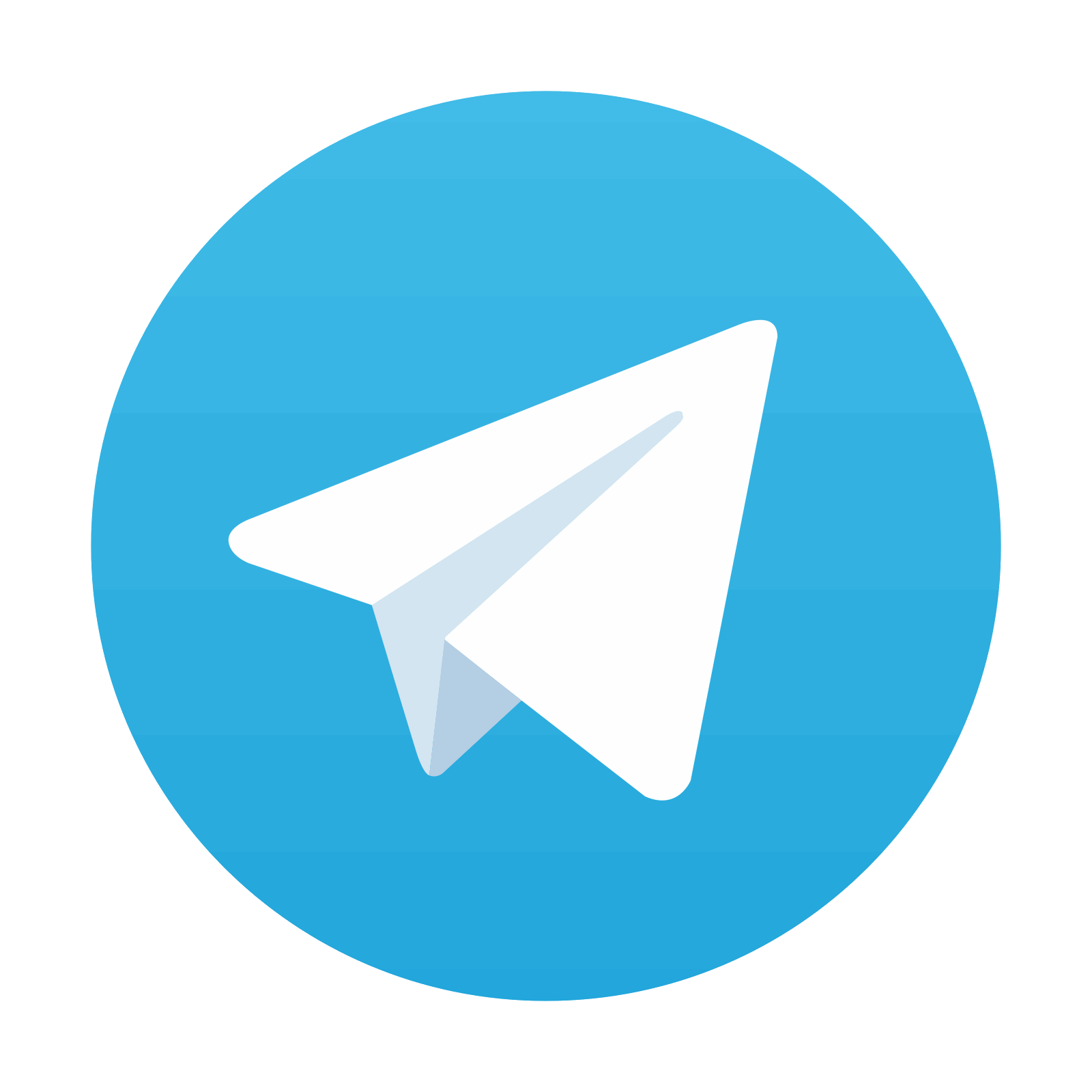
Stay updated, free articles. Join our Telegram channel

Full access? Get Clinical Tree
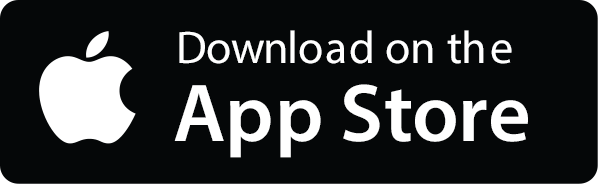
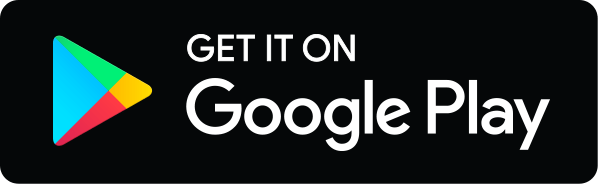
