CHAPTER 29 The Small Intestinal Phase of the Integrated Response to a Meal
The small intestine is the critical portion of the intestinal tract for assimilation of nutrients. In this site the meal is mixed with a variety of secretions that permit its digestion and absorption, and motility functions serve to ensure adequate mixing and exposure of the intestinal contents (chyme) to the absorptive surface. The small intestine has many specializations that enable it to perform its functions efficiently. One of the most obvious specializations is the substantial surface area of the mucosa. This is achieved in a number of different ways: the small intestine is essentially a long tube that is coiled inside the abdominal cavity, there are folds of the full thickness of the mucosa and submucosa, the mucosa has finger-like projections called villi, and finally, each epithelial cell has microvilli on its apical surface. Thus, a large surface area exists over which digestion and absorption occur.
GASTRIC EMPTYING IN THE SMALL INTESTINAL PHASE
Immediately after a meal, the stomach may contain up to a liter of material, which will empty slowly into the small intestine. The rate of gastric emptying is dependent on the macronutrient content of the meal and the amount of solids contained in the meal. Thus, solids and liquids of similar nutritional composition will empty at different rates. Liquids empty rapidly but solids do so only after a lag phase, which means that after a solid meal, there is a period of time during which little or no emptying occurs (Fig. 29-1).
Regulation of gastric emptying is achieved by alterations in motility of the proximal part of the stomach (fundus and corpus) and distal part of the stomach (pylorus and duodenum). Motor function in these regions is highly coordinated. Recall that during the esophageal and gastric phase of the meal, the predominant reflex response is receptive relaxation. At the same time, peristaltic movements in the more distal part of the stomach (antrum) mix the gastric contents with gastric secretions. The pyloric sphincter is largely closed. Even if it opens periodically, little emptying will occur because the proximal portion of the stomach is relaxed and the antral pump (antral contractions) is not very strong. Subsequently, gastric emptying is brought about by an increase in tone (intraluminal pressure) in the proximal portion of the stomach, increased strength of antral contractions (increased strength of the antral pump), opening of the pylorus to allow the contents to pass, and simultaneous inhibition of duodenal segmental contractions. Liquids and the semiliquid chyme flow down the pressure gradient from the stomach to the duodenum.
Pancreatic Secretion
As in the salivary glands, the pancreas has a structure that consists of ducts and acini. The pancreatic acinar cells line the blind ends of a branching ductular system that eventually empties into the main pancreatic duct and from there into the small intestine under control of the sphincter of Oddi. Also in common with salivary glands, a primary secretion arises in the acini and is then modified as it passes through the pancreatic ducts. In general, the acinar cells supply the organic constituents of the pancreatic juice in a primary secretion whose ionic composition is comparable to that of plasma, whereas the ducts dilute and alkalinize the pancreatic juice while reabsorbing chloride ions (Fig. 29-2). The major constituents of pancreatic juice, which amounts to approximately 1.5 L/day in adult humans, are listed in Table 29-1. This list also outlines the functions of pancreatic secretory products. Many of the digestive enzymes produced by the pancreas, particularly the proteolytic enzymes, are produced as inactive, precursor forms. Storage in these inactive forms appears to be critically important in preventing the pancreas from digesting itself.
Table 29-1 Products of Pancreatic Acinar Cells
Characteristics and Control of Ductular Secretion
In this section we consider how the pancreatic ductular cells contribute to the flow and composition of pancreatic juice in the postprandial period. The ducts of the pancreas can be considered the effector arm of a pH regulatory system designed to respond to luminal acid in the small intestine and secrete just enough bicarbonate to restore pH to neutrality (Fig. 29-3). This regulatory function also requires mechanisms to sense luminal pH and convey this information to the pancreas, as well as other epithelia (e.g., biliary ductules and the duodenal epithelium itself) capable of secreting bicarbonate. The pH-sensing mechanism is embodied in specialized endocrine cells localized within the small intestinal epithelium, known as S cells. When luminal pH falls below approximately 4.5, S cells are triggered to release secretin, presumably in response to protons. The components of this regulatory loop constitute a self-limited system. Thus, as secretin evokes secretion of bicarbonate, pH in the small intestinal lumen will rise and the signal for release of secretin from S cells will be terminated.
At the cellular level, secretin directly stimulates epithelial cells to secrete bicarbonate ions into the ductular lumen, with water following via the paracellular route to maintain osmotic equilibrium. Secretin increases cAMP in the ductular cells and thereby opens CFTR Cl− channels (Fig. 29-4) and causes an outflow of Cl− into the duct lumen. This secondarily drives the activity of an adjacent antiporter that exchanges the chloride ions for bicarbonate. There is also emerging evidence that CFTR itself may be permeable to some extent to bicarbonate ions when opened. In either case, the bicarbonate secretory process is dependent on CFTR, which provides a rationale for the defects in pancreatic function that are seen in the disease of cystic fibrosis, in which CFTR is mutated. The bicarbonate needed for this secretory process is derived from two sources. Some is taken up across the basolateral membrane of the ductular epithelial cells via the symporter NBC-1 (for sodium-bicarbonate cotransporter type 1). Recall that the process of gastric acid secretion results in an increase in circulating bicarbonate ions, which can serve as a source of bicarbonate to be secreted by the pancreas. However, bicarbonate can also be generated intracellularly via the activity of the enzyme carbonic anhydrase. The net effect is to move HCO3− into the lumen and thereby increase pH and the volume of pancreatic juice.
Characteristics and Control of Acinar Secretion
CCK is the product of I cells, which are also localized to the small intestinal epithelium. These classic enteroendocrine cells release CCK into the interstitial space when specific food components are present in the lumen, particularly free fatty acids and certain amino acids. Release of CCK from I cells may occur as a result of a direct interaction of fatty acids or amino acids, or both, specifically with the I cells themselves. Release of CCK is also regulated by two luminally acting releasing factors that can stimulate the I cell. The first of these, referred to as CCK-releasing factor (or peptide), is secreted by paracrine cells within the epithelium into the small intestinal lumen, probably in response to products of fat or protein digestion (or both). The second releasing factor, likewise a peptide, is called monitor peptide and is released by pancreatic acinar cells into pancreatic juice. Both CCK-releasing factor and monitor peptide can also be released in response to neural input, which is probably particularly important in initiating pancreatic secretion during the cephalic and gastric phases, thereby preparing the system to digest the meal as soon as it enters the small intestine.
What is the significance of these peptide-releasing factors? Their primary role appears to be to match CCK release, as well as the resulting availability of pancreatic enzymes, to the need for these enzymes to digest the meal in the small intestinal lumen (Fig. 29-5). Because the releasing factors are peptides, they will be subject to proteolytic degradation by enzymes such as pancreatic trypsin in exactly the same way as dietary protein. However, when dietary protein is ingested, it is present in much greater amounts in the lumen than the releasing factors and thus “competes” with the releasing factors for proteolytic degradation. The net effect is that the releasing factors will be protected from breakdown while the meal is in the small intestine and are therefore available to continue stimulation of CCK release from I cells. However, once the meal has been digested and absorbed, the releasing factors are degraded and the signal for release of CCK is shut off.
The secretory products of pancreatic acinar cells are largely presynthesized and stored in granules that cluster toward the apical pole of acinar cells (Fig. 29-6). The most potent stimuli of acinar cell secretion, including CCK itself, acetylcholine, and gastrinreleasing peptide, act by mobilizing intracellular Ca++. Stimulation of acinar cells results in phosphorylation of a series of regulatory and structural proteins within the cell cytosol that serve to move the granules closer to the apical membrane, where fusion of granule and plasma membranes can occur. The contents of the granule are then discharged into the acinar lumen and subsequently washed out of the pancreas by an exudate of plasma crossing the tight junctions linking the acinar cells together and ultimately by ductular secretions. In the period between meals, in contrast, the granule constituents are resynthesized by the acinar cells and then stored until needed to digest the next meal. The signals that mediate granule resynthesis are less well understood, but resynthesis may be stimulated by the same agonists that evoke the initial secretory response.
< div class='tao-gold-member'>
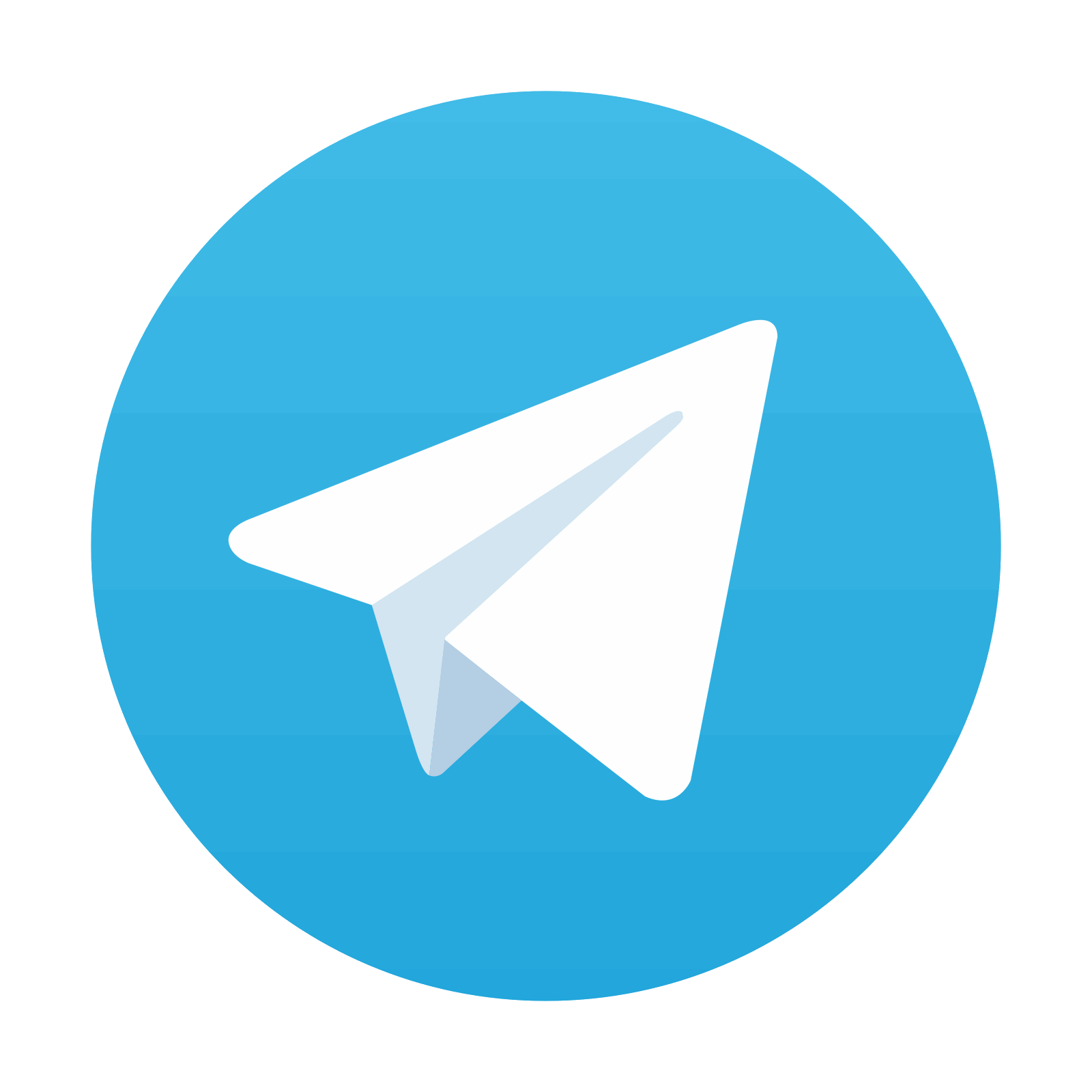
Stay updated, free articles. Join our Telegram channel

Full access? Get Clinical Tree
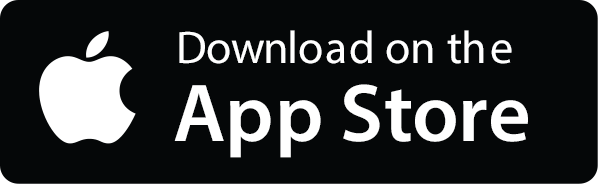
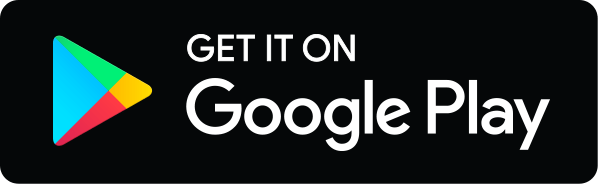