CHAPTER 42 The Adrenal Gland
In adults, the adrenal glands emerge as fairly complex endocrine structures that produce two structurally distinct classes of hormones: steroids and catecholamines. The catecholamine hormone epinephrine acts as a rapid responder to stresses such as hypoglycemia and exercise to regulate multiple parameters of physiology, including energy metabolism and cardiac output. Stress is also a major secretagogue of the longer-acting steroid hormone cortisol, which regulates glucose utilization, immune and inflammatory homeostasis, and numerous other processes. In addition, the adrenal glands regulate salt and volume homeostasis through the steroid hormone aldosterone. Finally, the adrenal gland secretes large amounts of the androgen precursor dehydroepiandrosterone sulfate (DHEAS), which plays a major role in fetoplacental estrogen synthesis and as a substrate for peripheral androgen synthesis in women.
ANATOMY
The adrenal glands are bilateral structures located immediately above the kidneys (ad, near; renal, kidney) (Fig. 42-1). In humans, they are also referred to as the suprarenal glands because they sit on the superior pole of each kidney. The adrenal glands are similar to the pituitary in that they are derived from both neuronal tissue and epithelial (or epithelial-like) tissue. The outer portion of the adrenal gland, called the adrenal cortex (Fig. 42-2), develops from mesodermal cells in the vicinity of the superior pole of the developing kidney. These cells form cords of epithelial endocrine cells. The cells of the cortex develop into steroidogenic cells (see Chapter 37). In adults, the adrenal cortex is composed of three zones—the zona glomerulosa, the zona fasciculata, and the zona reticularis—that produce mineralocorticoids, glucocorticoids, and adrenal androgens, respectively (Fig. 42-2, B).
Soon after the cortex forms, neural crest-derived cells associated with the sympathetic ganglia, called chromaffin cells, migrate into the cortex and become encapsulated by cortical cells. Thus, the chromaffin cells establish the inner portion of the adrenal gland, which is called the adrenal medulla (Fig. 42-2). The chromaffin cells of the adrenal medulla have the potential to develop into postganglionic sympathetic neurons. They are innervated by cholinergic preganglionic sympathetic neurons and can synthesize the catecholamine neurotransmitter norepinephrine from tyrosine. The enzyme phenylethanolamine N-methyl transferase adds a methyl group to norepinephrine to produce the catecholamine hormone epinephrine, which is the primary hormonal product of the adrenal medulla (Fig. 42-2, B).
ADRENAL MEDULLA
Synthesis of Epinephrine
The enzymatic steps in the synthesis of epinephrine are shown in Figure 42-4. Synthesis begins with transport of the amino acid tyrosine into the chromaffin cell cytoplasm and the subsequent hydroxylation of tyrosine by the rate-limiting enzyme tyrosine hydroxylase to produce dihydroxyphenylalanine (DOPA). DOPA is converted to dopamine by a cytoplasmic enzyme, aromatic amino acid decarboxylase, and is then transported into the secretory vesicle (also called the chromaffin granule). Within the granule, dopamine is completely converted to norepinephrine by the enzyme dopamine β-hydroxylase. In most adrenomedullary cells, essentially all of the norepinephrine diffuses out of the chromaffin granule by facilitated transport and is methylated by the cytoplasmic enzyme phenylethanolamine-N-methyltransferase (PNMT) to form epinephrine. Epinephrine is then transported back into the granule.
Secretion of epinephrine and norepinephrine from the adrenal medulla is regulated primarily by descending sympathetic signals in response to various forms of stress, including exercise, hypoglycemia, and hemorrhagic hypovolemia (Fig. 42-5). The primary autonomic centers that initiate sympathetic responses reside in the hypothalamus and brainstem, and they receive input from the cerebral cortex, the limbic system, and other regions of the hypothalamus and brainstem.
The high local concentration of cortisol in the medulla is maintained by the vascular configuration within the adrenal gland. The outer connective tissue capsule of the adrenal gland is penetrated by a rich arterial supply coming from three main arterial branches (i.e., the inferior, middle, and superior suprarenal arteries; Fig. 42-1). These give rise to two types of blood vessels that carry blood from the cortex to the medulla (Fig. 42-3): (1) relatively few medullary arterioles, which provide high oxygen- and nutrient-laden blood directly to the medullary chromaffin cells, and (2) relatively numerous cortical sinusoids, into which cortical cells secrete steroid hormones (including cortisol). Both vessel types fuse to give rise to the medullary plexus of vessels that ultimately drains into a single suprarenal vein. Thus, secretions of the adrenal cortex percolate through the chromaffin cells and bathe them in high concentrations of cortisol before leaving the gland and entering the inferior vena cava. Cortisol inhibits neuronal differentiation of the medullary cells, so they fail to form dendrites and axons. Additionally, cortisol induces expression of the enzyme phenylethanolamine-N-methyltransferase (PNMT), which converts norepinephrine to epinephrine (Fig. 42-4). Glucocorticoid receptor (see later) knockout mice have an enlarged cortex, but the size of the medulla is decreased and PNMT activity is undetectable.
The chemical signal for secretion of catecholamine from the adrenal medulla is acetylcholine (ACh), which is secreted from preganglionic sympathetic neurons and binds to nicotinic receptors on chromaffin cells (Fig. 42-5). ACh increases the activity of the rate-limiting enzyme tyrosine hydroxylase in chromaffin cells (Fig. 42-4). It also increases the activity of dopamine β-hydroxylase and stimulates exocytosis of the chromaffin granules. Synthesis of epinephrine and norepinephrine is closely coupled to secretion so that levels of intracellular catecholamines do not change significantly, even in the face of changing sympathetic activity.
Mechanism of Action of Catecholamines
Adrenergic receptors are generally classified as α- and β -adrenergic receptors, with the α-adrenergic receptors further divided into α1 and α2 receptors and the β-adrenergic receptors divided into β1, β2, and β3 receptors (Table 42-1). These receptors can be characterized according to (1) Relative potency of endogenous and pharmacological agonists and antagonists. Epinephrine and norepinephrine are potent agonists for α receptors and for β1 and β3 receptors, whereas epinephrine is more potent than norepinephrine for β2 receptors. A large number of synthetic selective and nonselective adrenergic agonists and antagonists now exist. (2) Downstream signaling pathways. Table 42-1 shows the primary pathways that are coupled to the different adrenergic receptors. This is an oversimplification because differences in signaling pathways for a given receptor have been linked to the duration of agonist exposure and cell type. (3) Location and relative density of receptors. Importantly, different receptor types predominate in different tissues. For example, although both α and β receptors are expressed by pancreatic islet beta cells, the predominant response to a sympathetic discharge is mediated by α2 receptors.
Physiological Actions of Adrenomedullary Catecholamines
Because the adrenal medulla is directly innervated by the autonomic nervous system, adrenomedullary responses are very rapid. Furthermore, because of the involvement of several centers in the central nervous system (CNS), most notably the cerebral cortex, adrenomedullary responses can precede onset of the actual stress (i.e., they can be anticipated) (Fig. 42-5). In many cases, the adrenomedullary output, which is primarily epinephrine, is coordinated with sympathetic nervous activity, as determined by the release of norepinephrine from postganglionic sympathetic neurons. However, some stimuli (e.g., hypoglycemia) evoke a stronger adrenomedullary than sympathetic nervous response, and vice versa.
Many organs and tissues are affected by a sympathoadrenal response (Table 42-2). An informative example of the major physiological roles of catecholamines is the sympathoadrenal response to exercise. Exercise is similar to the “fight or flight” response, but without the subjective element of fear, and involves a greater adrenomedullary response (i.e., endocrine role of epinephrine) than a sympathetic nervous response (i.e., neurotransmitter role of norepinephrine). The overall goal of the sympathoadrenal system during exercise is to meet the increased energy demands of skeletal and cardiac muscle while maintaining sufficient oxygen and glucose supply to the brain. The response to exercise includes the following major physiological actions of epinephrine (Fig. 42-6):
Table 42-2 Some Actions of Catecholamine Hormones
β: Epinephrine > Norepinephrine | α: Norepinephrine > Epinephrine |
---|---|
↑ Glycogenolysis | ↑ Gluconeogenesis (α1) |
↑ Gluconeogenesis (β2) | ↑ Glycogenolysis (α1) |
↑ Lipolysis (β3) (β2) | |
↑ Calorigenesis (β1) | |
↓ Glucose utilization | |
↑ Insulin secretion (β2) | ↓ Insulin secretion (α2) |
↑ Glucagon secretion (β2) | |
↑ Muscle K+ uptake (β2) | ↑ Cardiac contractility (α1) |
↑ Cardiac contractility (β1) | |
↑ Heart rate (β1) | |
↑ Conduction velocity (β1) | |
↑ Arteriolar dilation: ↓ BP (β2) (muscle) | ↑ Arteriolar vasoconstriction; ↑ BP (α1) (splanchnic, renal, cutaneous, genital) |
↑ Muscle relaxation (β2) | ↑ Sphincter contraction (α1) |
Gastrointestinal | Gastrointestinal |
Urinary | Urinary |
Bronchial | ↑ Platelet aggregation (α2) |
↑ Sweating (“adrenergic”) | |
↑ Dilation of pupils (α1) |
BP, blood pressure.
Metabolism of Catecholamines
Two primary enzymes are involved in the degradation of catecholamines: monoamine oxidase (MAO) and catechol-O-methyltransferase (COMT) (Fig. 42-7). The neurotransmitter norepinephrine is degraded by MAO and COMT after uptake into the presynaptic terminal. This mechanism is also involved in the catabolism of circulating adrenal catecholamines. However, the predominant fate of adrenal catecholamines is methylation by COMT in nonneuronal tissues such as the liver and kidney. Urinary vanillylmandelic acid (VMA) and metanephrine are sometimes used clinically to assess the level of catecholamine production in a patient. Much of the urinary VMA and metanephrine is derived from neuronal rather than adrenal catecholamines.
ADRENAL CORTEX
Zona Fasciculata
The zona fasciculata produces the glucocorticoid hormone cortisol. This zone is an actively steroidogenic tissue composed of straight cords of large cells. These cells have a “foamy” cytoplasm because they are filled with lipid droplets that represent stored cholesterol esters. These cells make some cholesterol de novo but also import cholesterol from blood in the form of low-density lipoprotein (LDL) and high-density lipoprotein (HDL) particles. Free cholesterol is then esterified and stored in lipid droplets (Fig. 42-8). The stored cholesterol is continually turned back into free cholesterol by a cholesterol ester hydrolyase, a process that is increased in response to the stimulus of cortisol synthesis (e.g., adrenocorticotropic hormone [ACTH]’see later). In the zona fasciculata, cholesterol is converted sequentially to pregnenolone, progesterone, 17-hydroxyprogesterone, 11-deoxycortisol, and cortisol (Figs. 42-9 and 42-10). A parallel pathway in the zona fasciculata involves the conversion of progesterone to 11-deoxycorticosterone (DOC) and then to corticosterone (Fig. 42-10, C). This pathway is minor in humans, but in the absence of active CYP11B1 (11-hydroxylase activity), the production of DOC is significant. Because DOC acts as a weak mineralocorticoid (Table 42-3), elevated levels of DOC cause hypertension.
Table 42-3 Relative Glucocorticoid and Mineralocorticoid Potency of Natural Corticosteroids and Some Synthetic Analogues in Clinical Use*
Glucocorticoid | Mineralocorticoid | |
---|---|---|
Corticosterone | 0.5 | 1.5 |
Prednisone (1.2 double bond) | 4 | <0.1 |
6α-Methylprednisone (Medrol) | 5 | <0.1 |
9α-Fluoro-16α-hydroxyprednisolone (triamcinolone) | 5 | <0.1 |
9α-Fluoro-16α-methylprednisolone (dexamethasone) | 30 | <0.1 |
Aldosterone | 0.25 | 500 |
Deoxycorticosterone | 0.01 | 30 |
9α-Fluorocortisol | 10 | 500 |
* All values are relative to the glucocorticoid and mineralocorticoid potencies of cortisol, which have each been set at 1.0 arbitrarily. Cortisol actually has only 1/500 the potency of the natural mineralocorticoid aldosterone.
Pheochromocytoma is a tumor of chromaffin tissue that produces excessive quantities of catecholamines. These are commonly adrenal medullary tumors, but they can occur in other chromaffin cells of the autonomic nervous system. Although pheochromocytomas are not common tumors, they are the most common cause of hyperfunctioning of the adrenal medullary. The catecholamine most frequently elevated in pheochromocytoma is norepinephrine. For unknown reasons, the symptoms of excessive catecholamine secretion are often sporadic rather than continuous. Symptoms include hypertension, headaches (from hypertension), sweating, anxiety, palpitations, and chest pain. In addition, patients with this disorder may show orthostatic hypotension (despite the tendency for hypertension). This occurs because hypersecretion of catecholamines can decrease the postsynaptic response to norepinephrine as a result of down-regulation of the receptors (see Chapter 3). Consequently, the baroreceptor response to blood shifts that occurs on standing is blunted.
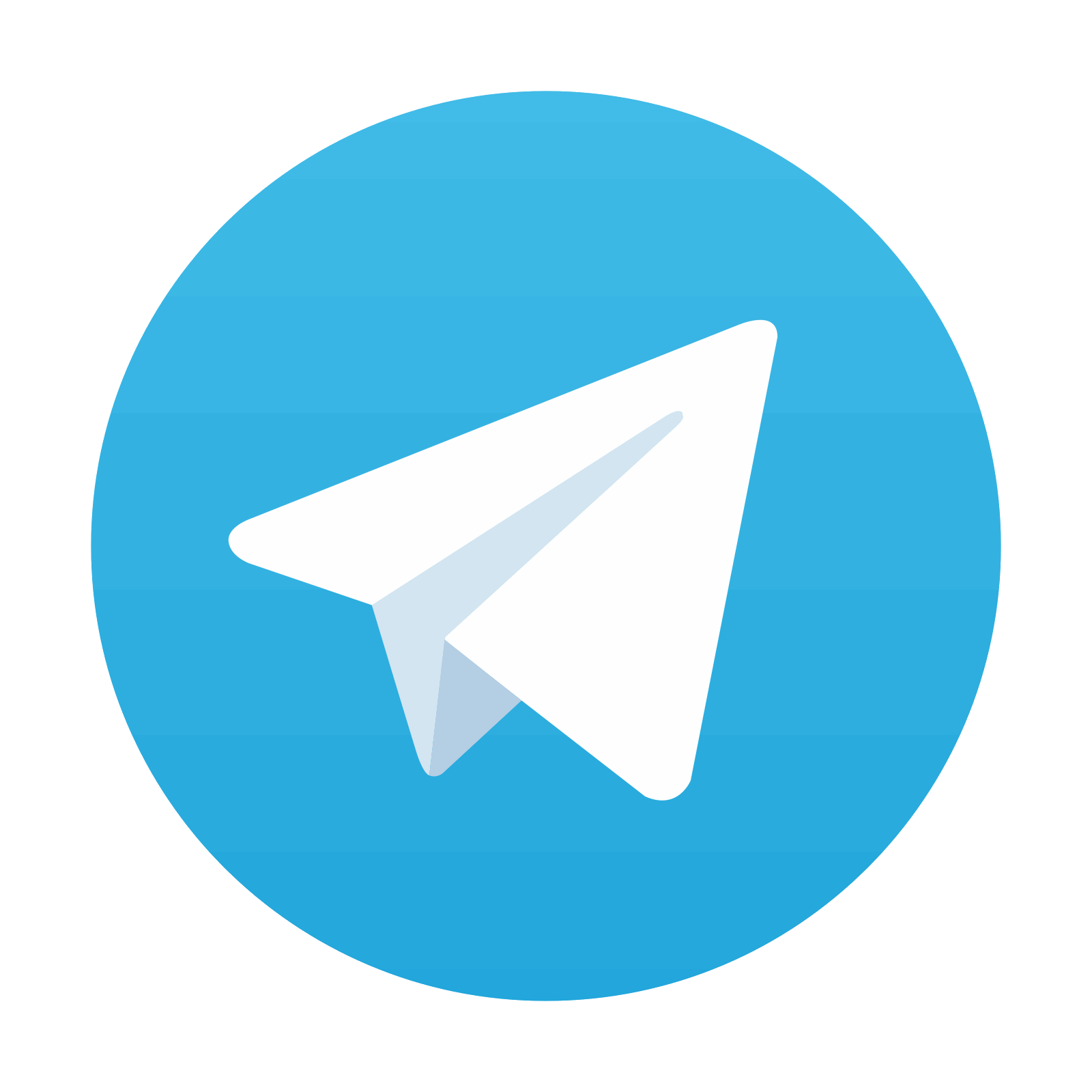
Stay updated, free articles. Join our Telegram channel

Full access? Get Clinical Tree
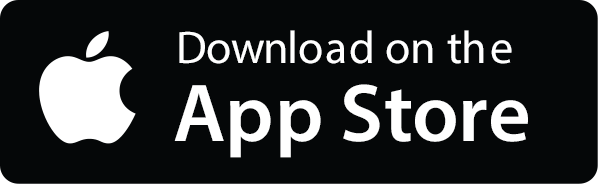
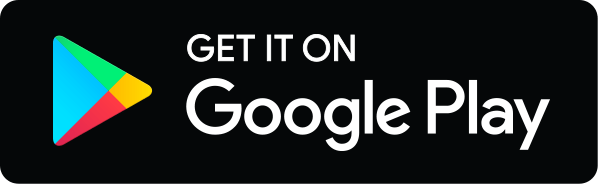