Figure 106-1. Enlarging uterus during gestation. At 12 weeks, the uterus rises out of the pelvis into the abdomen. At 20 weeks, the fundus is at the height of the umbilicus, and at 36 weeks, the uterus reaches to the upper abdomen.
PREMATURE ONSET OF LABOR
3 Preterm birth is defined as birth between 200/7 weeks and 366/7 weeks of gestation. The diagnosis is made clinically on the basis of the presence of regular uterine contractions accompanied by a change in cervical dilatation, effacement, or both, or initial presentation with regular contractions and cervical dilatation of at least 2 cm. Although only 12% of all live births in the United States fit this criterion, prematurity is responsible for approximately 70% of neonatal deaths, 36% of infant deaths, 25% to 50% of cases of long-term neurologic impairment in children, and a high cost to the health care system.13–17 Preterm labor with intact membranes is not the only cause of preterm birth; numerous preterm births are preceded by either rupture of membranes or medical/surgical problems necessitating delivery.18,19 The etiology of preterm labor is often multifactorial, but the onset of labor from fetal and uterine stress during surgical manipulation or intra-abdominal inflammatory processes is of most concern to the surgeon. Bacterial infection of the amniotic tissues or peritoneum leads to a local increase in eicosanoids and the onset of labor.20 Endotoxin acts on inflammatory cells to increase production of IL-1 and IL-6, which also amplify local prostaglandin production. All of these factors can act in concert to initiate and propagate preterm labor. Uterine activation and increased expression of gap junctions as well as oxytocin receptors can be induced by uterine stretch.21 Uterine trauma due to surgical manipulation can affect these same mechanisms, leading to premature uterine contractions. The interrelations at various levels between the inflammatory response, uterine and maternal trauma, and the initiation of labor can represent the body’s attempt to expel the fetus from a hostile uterine environment.
Tocolysis
4 To date, there is no evidence that tocolytic therapy has associated direct benefit on improving neonatal outcome, except for short-term prolongation of pregnancy (up to 48 hours) to allow for the administration of antenatal steroids, transport of the patient to a tertiary care facility, and fetal neuroprotection.22–24 In general, tocolysis is indicated between viability (24 weeks of gestation) and 34 weeks of gestation in women with preterm contractions with cervical change. However, it is accepted to administer tocolytics before viability after events known to cause preterm labor, such as intra-abdominal surgery and in utero fetal surgery although evidence of efficacy for such intervention is lacking.25,26 Prostaglandin synthesis inhibitors, magnesium sulfate, beta2-adrenergic agonists, and calcium channel blockers represent the four most common pharmacologic agents used as tocolytics. Because of the importance of prostaglandins in the initiation of uterine contraction, prostaglandin synthetase inhibitors are used to stop premature labor. Indomethacin is the nonsteroidal drug used most frequently for tocolysis because of its quick onset of action and ease of dosing. Studies report a 95% tocolytic success rate at 48 hours and a potential delay in delivery of 1 week in 80% of patients.27 Magnesium sulfate has long been used in the treatment of preeclampsia and has gained acceptance as a tocolytic and more recently as a neuroprotective agent to reduce the risk and severity of cerebral palsy when birth is anticipated before 32 weeks of gestation. Most data support the theory that magnesium exerts its effects by calcium antagonism. High intracellular magnesium concentrations inhibit Ca2+ entry into myometrial cells, interfering with actin–myosin coupling. High magnesium concentrations also increase the sensitivity of K+ channels, favoring hyperpolarization and uterine relaxation. Maternal serum levels necessary to inhibit myometrial contractility range between 4 and 9 mg/dL, two to six times normal levels.28 Beta2-adrenergic agonists used in the treatment of preterm labor include ritodrine and terbutaline. Stimulation of uterine beta2 receptors leads to activation of adenylate cyclase and an increase in intracellular cyclic adenosine monophosphate (cAMP) concentration. Activation of -dependent protein kinase A inhibits myosin light-chain phosphorylation and actin–myosin coupling. Protein kinase A activity is also associated with increased Ca2+ efflux, decreased Ca2+ influx, and increased K+ conductance. All these actions lead to myometrial relaxation. Because of reports of serious maternal side effects and possible adverse behavioral effects in offspring after in utero exposure to terbutaline, the U.S. Food and Drug Administration (FDA) issued a warning regarding the aforementioned agent and suggested that its use be limited to short-term inpatient acute tocolysis particularly in the setting of tachysystole.29,30 Calcium channel blockers inhibit entry of calcium through voltage-dependent Ca2+ channels. The use of oral nifedipine can abolish uterine activity and prevent delivery with minimal maternal side effects and no reported fetal or neonatal adverse effects.31 However, maternal and fetal side effects can be serious with other tocolytic agents. Premature constriction of the fetal ductus arteriosus and necrotizing enterocolitis with the use of indomethacin has been reported and can result in neonatal pulmonary hypertension and even death.32,33 Maternal cardiovascular side effects, including pulmonary edema, can occur in 5% of beta2 agonist users and 10% of those treated with magnesium. Although this complication is rare, it can result in maternal death. Serum concentrations of magnesium needed for tocolysis (4 to 9 mg/dL) are not far from levels causing ablation of deep tendon reflexes (9 to 13 mg/dL) or respiratory depression (14 mg/dL). The decision to use tocolytics must be made only after the diagnosis of premature onset of labor is confirmed, and the benefits of prolonging gestation outweigh the risks of tocolysis. Initiation of tocolytic therapy for potential but unconfirmed preterm labor is discouraged.34 Maintenance therapy with any of the aforementioned tocolytic agents has not been demonstrated to prolong pregnancy or improve neonatal outcomes after an initial treated episode of threatened preterm birth and, therefore, should not be used for this purpose.35–37
FETAL MONITORING
Perioperative fetal monitoring as well as the monitoring of premature uterine contractions is based on technology developed for intrapartum fetal monitoring in the late 1950s and early 1960s. Observational studies performed at that time and in the mid-1970s convincingly established a relationship between the degree of fetal acidemia and the presence and depth of decelerations and decreased or absent fetal heart rate variability.38,39 Established heart rate patterns and variable cardiac responses to fetal and maternal stimuli reflect an intact, well oxygenated central nervous system (CNS). Identification of deviation from those patterns indicative of metabolic acidosis was hoped to decrease the incidence of hypoxic–ischemic encephalopathy and death. Meta-analysis of randomized controlled trials comparing electronic fetal monitoring with intermittent auscultation has failed to show that electronic fetal monitoring decreased neurologic morbidity and mortality.40 Recently, researches using the US 2004-linked birth and infant death data found that the use of electronic fetal monitoring was associated with a substantial decrease in early neonatal morbidity particularly in preterm fetuses.41 However, authorities in the field remain skeptical of the findings and have cautioned that an epidemiologic association between fetal monitoring and reduced neonatal death does not establish causation.42 This controversy is not expected to be solved any time in the near future, but the fact is that of the approximately 4 million live births a year in the United States, 85% are assessed with fetal heart rate monitoring, making it the most common procedure in pregnancy.43 Fetal heart rate monitoring may be performed externally or internally. Most external monitor devices use the Doppler principle with computerized logic to count and interpret the Doppler signals originating from the fetal heart and maternal uterus. Internal fetal heart rate monitoring is accomplished with a spiral device attached to the fetal scalp or other presenting part. A transcervical intrauterine pressure catheter is sometimes used to monitor more accurately the strength and frequency of uterine contractions (Fig. 106-2). Both tracings are printed on a continuous strip of paper, with the fetal heart rate plotted at the top of the graph and uterine activity at the bottom.
Fetal Heart Rate
Normal fetal heart rate during pregnancy ranges between 110 and 160 beats/min. A prolonged baseline above 160 beats/min is considered a tachycardia, whereas that below 110 beats/min is a bradycardia. These terms refer to long-term patterns, and minute-to-minute variability in the heart rate away from baseline is known as acceleration and deceleration. Normal fetal heart rate tracings express continuous adjustment in the vagal tone through variability in the heart rate. Short-term or beat-to-beat variability is superimposed on broader, long-term variability of the baseline by three to five beats/min. The fetal heart rate response governed by a nonacidotic, well-functioning vagal conduction system manifests both long-term and short-term variability, with fluctuations of peaks and troughs ranging from 6 to 25 beats/min (Fig. 106-3). Decreased heart rate variability, either long or short term, can signal diminished CNS activity. Although this can be a physiologic response of the fetal sleep–wake cycle or maternal medication and sedatives, persistently decreased reactivity can signal CNS acidosis. Fetal heart rate accelerations are normal findings in the second half of pregnancy and occur as a result of increased sympathetic stimulation and decreased parasympathetic stimulation with fetal movements. Fetal heart rate decelerations are usually encountered during uterine contractions of the intrapartum period. Evaluation of decelerations during labor and as part of intraoperative fetal monitoring can give clues to the status of the placental blood supply. A prolonged deceleration is a visually apparent decrease in the fetal heart rate baseline that is 15 minutes or more, lasting 2 minutes or more but less than 10 minutes in duration. Early decelerations are characterized by a symmetrical and gradual decrease and return of the fetal heart rate that mirror uterine contractions and reflect increased vagal tone from a transient increase in intracranial pressure. This pattern is considered a benign physiologic manifestation of a functioning autonomic nervous system and has no adverse outcome. Variable decelerations are distinctively recognized by their abrupt decrease in fetal heart rate of 15 beats/min or greater from the onset of the deceleration to the beginning of the fetal heart rate nadir of 30 seconds, lasting 15 seconds or greater, and less than 2 minutes in duration. When variable decelerations are associated with uterine contractions, their onset, depth, and duration vary with successive uterine contractions. Isolated variable decelerations have little clinical significance, but if persistent with inadequate recovery between contractions, fetal hypoxemia and acidosis can occur. Late decelerations present usually as a symmetrical and gradual decrease and return of the fetal heart rate associated with a uterine contraction. The deceleration is delayed in timing, with its nadir occurring after the peak of the contraction. Late decelerations have an ominous connotation as they usually represent significant uteroplacental compromise; causes can range from maternal hypotension due to inferior vena cava compression to hypoxia or anemia.44 Intraoperative fetal compromise as indicated by an ominous heart rate tracing must be treated by correcting maternal hypotension or increasing oxygen delivery with increased FIO2, positional changes, aggressive fluid resuscitation, or blood transfusion. The inability to improve the intrauterine milieu can necessitate an emergent cesarean section to prevent fetal demise.45 The decision to use continuous fetal heart rate monitoring during nonobstetric surgery in pregnancy is controversial and should be based on gestational age, type, site, duration, and extent of the procedure. For previable pregnancies (<23 weeks), documentation of fetal heart activity by Doppler before and after the procedure suffices.46 For viable gestations, there is less consensus for fetal heart rate monitoring, but at a minimum, simultaneous electronic fetal heart rate and contraction monitoring should be performed before and after the procedure to assess fetal well-being and the absence of contractions. If the decision is to perform intraoperative electronic fetal heart monitoring, then all the necessary provisions should be made to provide optimal safety for the mother and the fetus. These include availability of obstetric care providers, anesthesiologists, neonatologists, and nurses working as a team and ready to intervene during the surgical procedure for fetal indications. Informed operative consent should thus be obtained in anticipation for a possible emergency delivery.47
Figure 106-2. Intraoperative and postoperative fetal monitoring using noninvasive surface Doppler and pressure sensor to monitor uterine contractions. (Reproduced from Miller DA, Paul R. Antepartum–intrapartum monitoring. In: Scott JR, DiSaia PJ, Hammond CB, et al., eds. Danforth’s Obstetrics and Gynecology. 8th ed. Philadelphia, PA: Lippincott Williams & Wilkins; 1999:243–255.)
IMAGING MODALITIES IN THE PREGNANT PATIENT
5 The most commonly used imaging modalities during pregnancy include radiography, ultrasonography, magnetic resonance imaging (MRI), and computed tomography (CT). The first and the latter are forms of ionizing radiation. The risks of ionizing radiation are an important source of anxiety for health care providers and patients that at times can force the physician to avoid the definitive diagnostic study of choice for fear that any radiation exposure would result in an anomalous fetus. This misconception could place both the mother and the fetus in greater danger from misdiagnosis. Although experimental data regarding the effects of ionizing radiation on the developing human fetus are impossible to obtain, animal exposure and follow-up of those inadvertently exposed to diagnostic and therapeutic radiation have provided some information. Retrospective studies of atomic bomb survivors in Hiroshima, Nagasaki, and victims of the atomic power plant disaster in Chernobyl have also allowed for evaluation of the effects of massive radiation exposure in utero.48,49
Figure 106-3. Fetal heart variability. A: Short-term and long-term variability both absent: abnormal. B: Short-term variability present, long-term variability absent: abnormal. C: Long-term variability present, short-term variability absent: abnormal. D: Both short- and long-term variability present: normal. (Reproduced from Miller DA, Paul R. Antepartum–intrapartum monitoring. In: Scott JR, DiSaia PJ, Hammond CB, et al., eds. Danforth’s Obstetrics and Gynecology. 8th ed. Philadelphia, PA: Lippincott Williams & Wilkins; 1999:243–255.)
Ionizing Radiation
Fetal effects of ionizing radiation depend on the dose absorbed by the fetal tissue and the stage of fetal development during exposure. Exposure is defined as the amount of ionic charge created by the radiation on passing through a defined mass of air. Units traditionally used to measure the effects of ionizing radiation include the radiation absorbed dose (rad) and the roentgen equivalents man (rem). Modern units include the gray (Gy) and sievert (Sv). According to the International System of Units, Gy is the unit that describes the potential radiation exposure from medical diagnostic equipment, but rad is the predominant measure used. One gray (Gy) is strictly defined as the deposition of 1.0 joule of energy per kilogram of tissue, and 1 rad is 1% of 1 Gy. As a gross estimate, exposure to 1 roentgen gives an absorbed dose of 1 rad or 10 mGy.
Sv is generally used to measure the public exposure to radiation as it accounts for factors such as type of radiation amount of time exposed, level of protection, and distance from the radiation source. One sievert (Sv) = 1 Gy = 100 rads. It is a misconception to assume that the radiation dose that a pregnant woman is exposed to or absorbs is the same as that absorbed by the fetus as the latter is partially protected from radiation injury by a pregnant woman’s surrounding soft tissues and uterus, both of which generally stop alpha and beta particles from penetration if they are not ingested, injected, or inhaled.50,51 Radiographic evaluation of the abdomen and pelvis is most likely to expose the fetus to direct ionizing radiation, whereas examination of the extremities and chest leads to exposure from scatter radiation only (Table 106-1).51 The potential deleterious consequences of radiation-induced ionization of vital cellular structures can be divided into four categories: (1) lethal effects, (2) teratogenic effect, (3) growth retardation, and (4) oncogenic potential. The occurrence of each effect depends upon the gestational age at the time of radiation exposure, the dose of radiation absorbed by the fetus, and fetal cellular repair mechanisms. The first three categories of adverse pregnancy outcomes have a deterministic effect whereby a threshold or No-Adverse-Effect Level exists. After exceeding the No-Adverse-Effect Level, a deterministic effect generally shows a gradient relationship with the absorbed dose – the larger the absorbed dose, the more severe the effect. On the contrary, cancer appears to have a stochastic effect in which, the probability, but not the severity of the effect, increases with the radiation dose. Stochastic effects are monoclonal, resulting in changes to the cell genome and altered differentiation and function of the affected cells.
Lethal Effects of Ionizing Radiation
The developing human is most sensitive to the lethal effects of ionizing radiation during the first 14 days after conception (3 to 4 weeks of gestational age). During this period, the “all or none” principle applies to the radiation-exposed embryo; it either survives undamaged or succumbs. At that gestational age, a dose of 100 to 200 mGy (10 to 20 rad) can be lethal for an embryo. Shortly thereafter, the threshold for fetal death increases to 250 to 500 mGy (25 to 50 rad). The estimated radiation dose to kill all embryos or fetuses less than 18 weeks of gestational age is 5,000 mGy (500 rad). At term, the fetal risk equals the pregnant woman’s risk at 20,000 mGy (2,000 rad).52,53
Table 106-1 Radiation Dosing to the Conceptus and Uterus from Selected Radiographic Examinations
Teratogenic Effects of Ionizing Radiation
Teratogenic effects of radiation can be seen during early organogenesis in animal studies. Exposure of rats to 100 rads at various points of development reveals a very narrow window for teratogenesis. This window corresponds to weeks 2 to 4 in human development, consistent with early organ formation. Despite these experimental data, it is difficult to link any specific human malformation with radiation exposure other than those of the CNS. Microcephaly, pigmentary changes in the retina, hydrocephalus, and optic nerve atrophy have been reported at a significantly higher rate after exposure to radiation in pregnancy.54 All patients were exposed to a minimum estimated dose of 100 rads, and no visceral, limb, or other malformations were found unless the child also exhibited CNS abnormalities. One explanation for this discrepancy is that the developmental period of the CNS is much longer, continuing throughout gestation and into the neonatal period, whereas other organs have a very narrow period during which morphologic alterations can be produced. Exposure to high doses of radiation during this narrow window is rare, and even if an isolated congenital abnormality were to occur, it would be difficult to separate this event from the background malformation rate. The extended period for CNS sensitivity to teratogenesis increases the prevalence of CNS malformation. Central nervous system malformation also occurs at threshold doses; however, low levels of exposure to 10 to 20 rads of radiation do not increase the incidence of microcephaly in experimental animals over baseline.55
Growth Retardation from Ionizing Radiation
Growth retardation results from radiation-induced cellular depletion. Although catch-up growth can occur if exposure happens early in gestation, permanent cell depletion and growth retardation occur with exposure during later fetal stages.56 Wood and colleagues58 studied growth retardation of children exposed in utero to the Japanese atomic blasts. Those within 1,500 m from the center of the explosion were exposed to over 25 rads and, when followed through age of 17 years, were 2 to 3 cm shorter and 3 kg lighter and had a head circumference 1-cm smaller than normal. Those beyond 1,500 m from the blast received less than 25 rads and had a normal head circumference, height, and weight.52,57,58 Further, animal and human data support the contention that exposures of less than 5 rads should not cause either anatomic malformation or growth retardation.55
Oncogenic Potential of Ionizing Radiation
The correlation between childhood cancer and in utero exposure to radiation has been demonstrated. Although conclusions regarding the cause-and-effect relationship of this correlation cannot be definitively established, further studies using twins59 and extensive data analysis60 have strengthened the argument that in utero radiation can increase the rate of childhood cancer even at doses of 1 rad. The current increase in childhood cancer is estimated at one to two cases per 3,000 children exposed to 1 rad in utero.57 Although these risks are very small, the patient must be counseled regarding this potential danger. By reviewing the current data, it is obvious that exposure to diagnostic levels of radiation carries little chance for spontaneous abortion, teratogenesis, or growth retardation. The increased risk of future cancer, however, does exist and must be weighed against the utility of the information provided by the study.
Ultrasound
Ultrasonography uses high-frequency, acoustic radiation to create images. A surface transducer containing piezoelectric crystals converts electrical energy into sound waves. Sound waves pass through tissue layers and are reflected back to the transducer when they encounter an interface between tissues of different densities. Digital images generated at 50 to more than 100 frames per second undergo postprocessing that produces the appearance of real-time imaging. Ultrasonography is considered the first-line diagnostic imaging modality of the pregnant surgical patient because it is nonionizing, widely available, portable, and of adequate diagnostic performance. This imaging technique has been used in obstetrics for more than 50 years without reported fetal damage or harmful effects.61,62 Ultrasonography is, however, a form of energy with effects on tissues through which the waveform penetrates. The two major mechanisms involved in potentially causing bioeffects are direct, resulting from the alternation of positive and negative pressures (mechanical or cavitation effects) and indirect, caused by heating of tissues secondary to transformation of the acoustic energy (thermal effects). Because of these theoretical concerns, several organizations have recommended that this diagnostic procedure should be performed only when there is a valid medical indication and the lowest possible ultrasonic exposure setting under the as low as reasonably achievable principle.63–65
Computed Tomography
In the past 10 years, the use of radiologic examinations in pregnant women has increased by 107%, with the greatest increase occurring in the use of CT.66 Computed tomography is most valuable in the second and third trimesters, when ultrasonography is of limited value, in a patient with a large body habitus, and when MRI is inconclusive or not available.67 Because of its sensitivity for the detection of abdominal and pelvic injury as well as retroperitoneal injury in which the clinical urgency requires prompt diagnosis, CT is the test of choice for injured pregnant patients.68–70 When CT is performed during pregnancy, using dose-reduction protocols (e.g., abdominal and internal barium shielding) results in significant reduction in the probability of biologic effects to the fetus without sacrificing the necessary diagnostic image quality.71
Magnetic Resonance Imaging
Like ultrasonography, MRI uses no ionizing radiation, instead relying on the magnetic properties of tissues to create images. Four magnetic fields interact during an MRI examination to create the image. The intrinsic magnetic field of an atomic nucleus, usually that of a hydrogen proton, is combined with a strong, uniformly applied external magnetic field and a weaker magnetic field gradient. In addition, a magnetic field is intermittently generated by pulsed radio frequency waves.52 When the radio frequency ceases, the nuclei return to their previous orientation and emit the radio frequency they absorbed. These signals are detected, stored, and processed by a computer to create an image. Theoretical concerns include the effects of fluctuating electromagnetic fields and high sound intensity levels with potential damage to the fetal ear. Other known dangers of MRI exposure include trauma from projection of small metal fragments into the eyes, interference with the operation of implantable electronic devices, position of metallic implants, and burns from heating of conductive materials in implants. Despite these concerns, present data have not conclusively documented any deleterious effects of MRI exposure on the developing conceptus or the pregnant woman.72
PHYSIOLOGIC CHANGES OF PREGNANCY THAT MIMIC DISEASE
Pregnancy physiology reflects the changes necessary for fetal gestation. It is characterized by the enlarging, gravid uterus and a changing hormonal milieu. These conditions predispose to certain diseases and change maternal physiology to mask the diagnosis of others. The cardiovascular system is affected by pregnancy. The cardiac output increases by 30% to 50%, as does the total blood volume. The corresponding red blood cell volume increases only by 20% to 30%, leading to a decrease in the hematocrit. Although the maternal oxygen-carrying capacity is increased and the rheologic properties of blood are optimized for maximum nutrient delivery to the fetus, a physiologic anemia develops that can be confused with a pathologic state.73 Diagnosis of anemia due to chronic blood loss, as occurs with cancers of the gastrointestinal tract, may be delayed as the gradually decreasing hematocrit is attributed to pregnancy. Other laboratory evaluations can also mask various disease states (Table 106-2). Serum alkaline phosphatase levels gradually increase because of production of an alkaline phosphatase isozyme by the placenta. This does not reflect biliary or bone disease. Albumin levels, commonly used to measure hepatic synthetic function, decrease, and a relative leukocytosis mimics a systemic infection.
Table 106-2 Change During Pregnancy in Laboratory Values
The gastrointestinal tract undergoes a state of relaxation and decreased smooth muscle activity during pregnancy. The lower esophageal sphincter tone gradually decreases, as does gastric emptying. Prolonged small bowel transit time and decreased colonic emptying not only work to maximize nutrient and water absorption but also contribute to the constipation reported by 38% of pregnant women.74 Although multifactorial, the actions of progesterone have been linked to inhibition of gut smooth muscle activity both in vitro and in vivo. Because this dose-dependent inhibition can be blocked by increasing the extracellular concentration of Ca2+, at least in vitro, it is proposed than progesterone works by limiting the effective Ca2+ available for actin–myosin coupling.75,76 This same mechanism contributes to uterine relaxation early in pregnancy, decreased intestinal motility, and the concurrent decrease in gallbladder tone and rate of emptying. With these changes, combined with supersaturation of bile by cholesterol, pregnancy becomes a lithogenic state.77 These effects of pregnancy are transient and gallbladder motility and volumes return to normal as early as 2 weeks after pregnancy, but the gallstones, if formed, may persist. Nausea and vomiting of pregnancy is a common occurrence affecting between 50% and 90% of all women.78,79 These symptoms rarely extend beyond the first trimester. Other causes must be investigated if the nausea and vomiting occur later in pregnancy. Abdominal pain, although often due to a surgical pathologic process, can have many causes. Round ligament pain is described as an aching, dragging pain more often felt on the right side. It is a common occurrence until 31 weeks of gestation. Pain in the hypochondrium can result from uterine pressure on the lower ribs, and lower abdominal pain may be psychogenic, related to anxiety about the pregnancy or insecurity over the social situation.80 It is up to the surgeon to determine the cause of abdominal pain in the pregnant patient and decide the course of diagnosis and intervention.
APPENDICITIS IN THE PREGNANT PATIENT
6 Acute appendicitis is the most common nonobstetric cause of acute surgical abdomen during pregnancy.81 Disease is confirmed in 65% to 75% of suspected cases for an incidence of approximately 1:1,500 births.82 Although previous series suggested that the condition had similar incidences for nonpregnant adults, equal distribution throughout pregnancy,83 and a more common occurrence later in gestation,84,85 a recent large case-control study indicated a lower incidence than among nonpregnant individuals, particularly in the third trimester.86 The incidence decreases in the third trimester to 15% to 30% compared to rates ranging from 20% to 60% in the first and second trimesters.87,88 A well-known fact is that the anatomic and physiologic changes of the gravid state make the diagnosis difficult. Attributing abdominal pain to other etiologies (e.g., pyelonephritis, false labor, and gastroenteritis), failure to pursue further investigation, and the physicians’ fear of surgically induced premature labor all contribute to delayed diagnosis and timely appendectomy in pregnancy. Appendiceal perforation rates are higher when compared with those from nonpregnant populations (55% vs. 4% to 19%).89 This is probably explained by confounding variables leading to the delay of diagnosis and therapy. Several series have documented a correlation between a delay in the diagnosis and gestational age. In the first trimester, most diagnoses were made promptly and significant delay in operation with a rate of perforation of 49% reported for patients presenting in the second trimester and a rate as high as 70% for those presenting in the third trimester.83,90 Perforated appendicitis presents a greater infectious risk in the pregnant patient than in the population as a whole. The large uterus interferes with proper omental migration throughout the abdominal cavity and prevents the walling off of the inflammatory process. Braxton Hicks contractions disrupt adhesion formation, and the general increase in vascularity of the abdomen with greater lymphatic drainage allows rapid dissemination of infection. The high circulatory levels of adrenocorticoids in pregnancy have also been hypothesized to diminish the tissue inflammatory response and hinder containment of infection.91–93 All of the classic signs and symptoms of appendicitis are altered by pregnancy (Table 106-3). Anorexia, nausea, and vomiting occur in 58% to 77% of pregnant patients with appendicitis.83,90 Although anorexia, nausea, and vomiting in the first trimester are common, their occurrence during the second and third trimesters, especially if associated with abdominal pain, require a thorough investigation. In a classic 1932 study, Baer and colleagues94 evaluated the migration of the appendix through the duration of pregnancy with barium enemas. Beginning in the first trimester, the appendix and the cecum are gradually displaced by the uterus and start a caudal migration out of the pelvis into the upper abdomen. By the third trimester, the appendiceal tip can abut the gallbladder (Fig. 106-4). The vague, referred epigastric pain due to early appendiceal obstruction is not changed by this anatomic consideration, but the location of the somatic pain is. In a large study of pregnant women with proven appendicitis, abdominal pain was present in all patients. Location of pain in the right lower quadrant, however, was common only early in gestation, and a portion of patients reported pain in the right upper quadrant. This change in symptomatology is easily understood if the anatomic changes of the appendix are taken into consideration.83 Both guarding and rigidity are valuable findings on physical examination but are common only in the first trimester. Elevation of the abdominal wall from the more laterally placed, upward-directed appendix and the laxity of the abdominal wall musculature caused by this distention decrease the reliability of these findings. Only 42.9% of patients in the third trimester are reported to have abdominal spasm and guarding, compared with 80% during the first trimester.95 Psoas and obturator signs are similarly obscured as the appendix is moved from its normal location and have not been shown to have clinical significance. The results of laboratory examinations commonly used to assist in the diagnosis of appendicitis are also obscured by pregnancy. The physiologic leukocytosis of pregnancy, ranging from 5,000 to 12,000 white blood cells (WBCs)/mL, overlaps that of appendicitis. In one collected series, the WBC count of pregnant patients with proven appendicitis was not significantly elevated over these values, and only 25% of patients had a WBC count over 15,000. Most patients (50%) had WBC counts ranging between 10,000 and 15,000, and 25% had less than 10,000 WBCs/mL.83 Urinalysis is a useful adjunct in the workup of appendicitis but again presents a dilemma in pregnancy. Urinary tract infection is common in pregnancy as the enlarging uterus compresses the right ureter. Dilatation and stagnant flow in the right collecting system can contribute to bacterial overgrowth and infection. Pyuria without bacteria can also indicate involvement of the right ureter in the appendiceal inflammatory process. The presence of pyuria and bacteriuria does not rule out appendiceal inflammation because the two conditions may coexist in 40% of pregnant patients.90 The risk of adverse pregnancy outcomes after appendectomy during pregnancy has been reported by two large population-based studies. From the California Inpatient File of 3,133 pregnant women undergoing surgery for suspected appendicitis, the fetal loss rate was 23%, and it was doubled – 6% versus 11% – with simple versus complicated disease.96 In the other study, the fetal loss rate within 1 week of the procedure was 22%, particularly when the surgery was performed after 23 weeks.82 Fortunately, these same researchers noted no additional increase in the preterm birth rate among the pregnancies that continued beyond the first postoperative week. Perforated appendicitis in pregnancy rapidly leads to diffuse peritonitis, premature labor, and fetal loss.85,97
Table 106-3 Variation in Signs and Symptoms of Appendicitis During Pregnancy
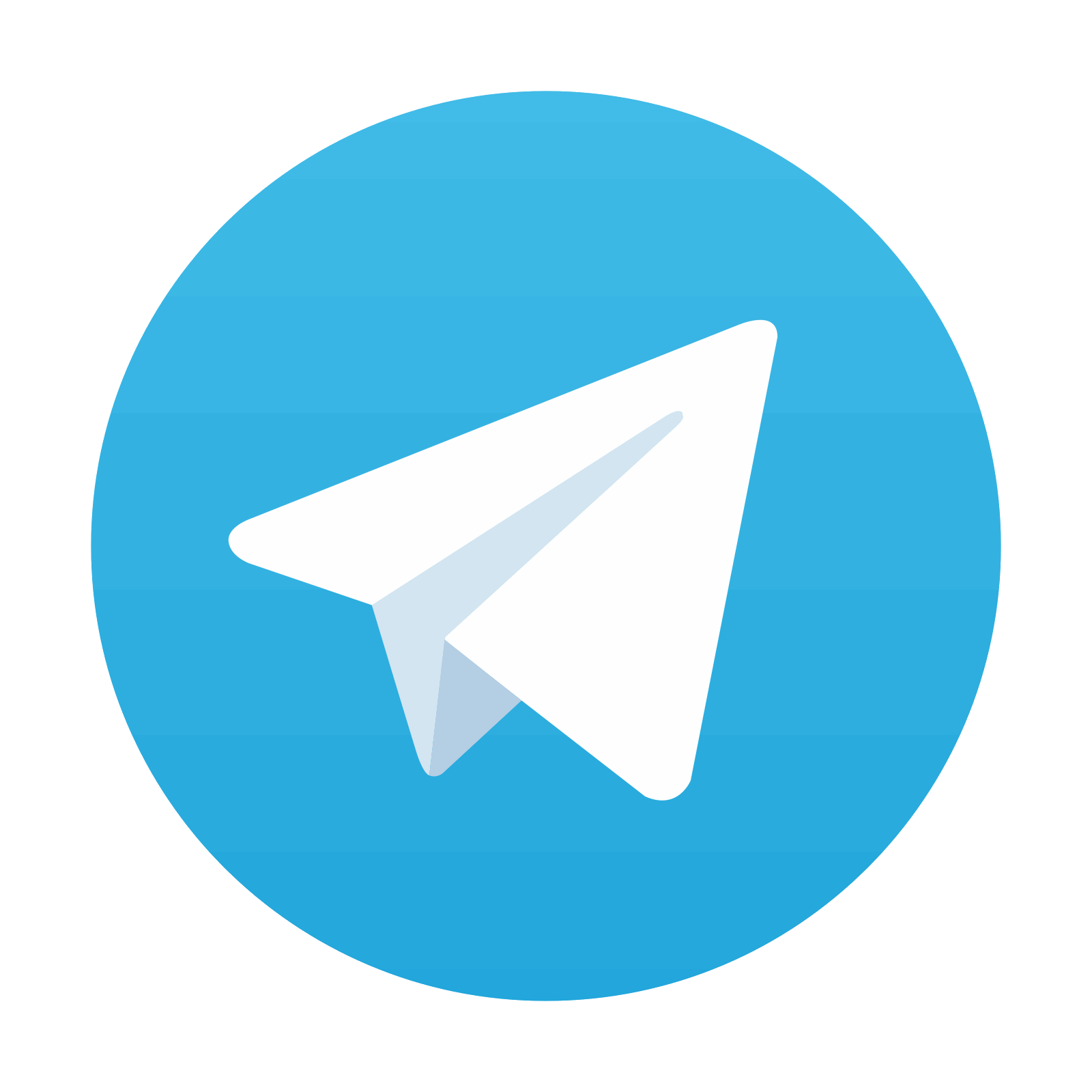
Stay updated, free articles. Join our Telegram channel

Full access? Get Clinical Tree
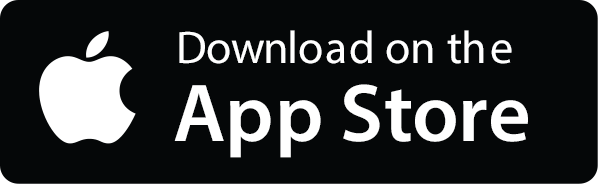
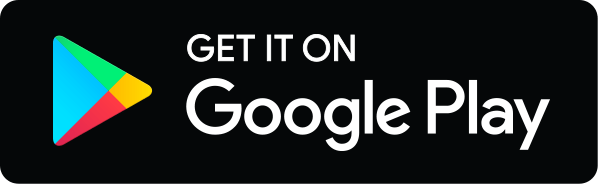