9
CHAPTER OUTLINE
■ DEFINITION OF DRUGS IN THE CLASS
■ SUBSTANCES INCLUDED IN THE CLASS
■ EPIDEMIOLOGY OF OPIOID ABUSE AND ADDICTION
■ PHARMACOKINETICS OF SPECIFIC DRUGS
■ TOXICITY STATES AND THEIR MEDICAL MANAGEMENT
■ MEDICAL COMPLICATIONS OF OPIOIDS
DEFINITION OF DRUGS IN THE CLASS
Three distinct types of opioid receptors are found in the nervous system: mu, kappa, and delta. Classic clinically used opioid analgesics act primarily as agonists or partial agonists at mu receptors. Heroin or illicit prescription opioids also act primarily as mu opioid receptor (MOP-r) agonists. Compounds in this class include the natural opiates (drugs derived from opium) and their man-made congeners which are agonists or antagonists, as well as the endogenous opioid neuropeptide agonists, products of three separate genes (1). The genes for each of these three receptors and each of these three classes of opioid peptides have been cloned from humans (2,3). These opioid receptors are members of the Gi-protein-coupled, 7-trans-membrane domain superfamily. The three main families of endogenous opioid peptides—beta-endorphin, enkephalins, and dynorphins—have a degree of selectivity for the three receptor types. For example, beta-endorphin and the enkephalins have relatively high affinity at mu and delta receptors and much lower at kappa. The dynorphins, by contrast, have relative selectivity for kappa receptors over the mu and delta. These receptors mediate a complex, partially overlapping array of physiologic and neurobiologic functions (4). For the purposes of this chapter, we will concentrate on the mu receptor as most of the clinically used opioids are active at this receptor. However, the entire endogenous opioid system plays an important role in responses to addictive opiates, including morphine, codeine, and heroin, as well as to synthetic opioids (3). Beta-endorphin is a product of proopiomelanocortin, which is produced primarily in the anterior pituitary of humans. It is also produced in the central nervous system (CNS) and in the periphery. The mu receptors mediate both the analgesic and rewarding effects of opioid compounds (be they heroin or prescription opioids) as well as their effects on many systems in the body, such as in the hypothalamic–pituitary–adrenal (HPA) axis, immune, gastrointestinal (GI), and pulmonary function.
The term “opioids” refers to all compounds, natural and synthetic, functionally related to opium derived from poppies and endogenous opioid neuropeptides. Opium is a naturally occurring mixture directly derived from the juice of the opium poppy (Papaver somniferum). Morphine (the prototypical MOP-r agonist) is the main active alkaloid in opium, whereas thebaine can be used as a starting point for production of semisynthetic MOP-r ligands. This chapter reviews the pharmacology of several exogenous opioids that are significant in the area of opioid addiction and its treatment, that is, heroin, morphine, oxycodone, codeine, meperidine, pentazocine, hydromorphone, and hydrocodone, as well as methadone, levo-alpha-acetylmethadol (LAAM), and buprenorphine.
SUBSTANCES INCLUDED IN THE CLASS
Heroin
Heroin is synthetically derived from the natural opioid alkaloid morphine. Largely owing to its very rapid onset of action and very short half-life, heroin is a popular drug of abuse. Heroin is classified in Schedule I (i.e., not available for any therapeutic use in the United States), although it is available in a few countries as a medication for treatment of heroin addiction (5,6). Heroin is a prodrug that is not itself active. It is most effective when used intravenously, but increasingly is used intranasally and, sometimes, smoked in the free base form (7). Intranasal and smoked routes may have increased in popularity because of the wider availability of high-purity heroin in recent years, and also as a means to reduce the risk of human immunodeficiency virus (HIV-1) transmission from intravenous use. Heroin is rapidly deacetylated to 6-monoace-tyl morphine and morphine, both of which are active at the mu opioid receptor.
Morphine and Synthetic Compounds
Morphine is a natural product of the poppy plant, Papaver somniferum. Chemically, morphine is an alkaloid that belongs to the class of phenanthrenes. This class also includes codeine and thebaine. Modifications of the latter result in synthetic compounds discussed below (4). Morphine is prescribed primarily as a high-potency analgesic. Biotransformations or synthetic modifications of the chemical structure of the morphine molecule at the 3, 6, and 17 positions produce other compounds, including morphine-6-glucuronide (M6G), a major pharmacologically active metabolite of morphine in humans. Related compounds include hydrocodone (Vicodin), oxycodone (OxyContin), hydromorphone (Dilaudid), and heroin. Synthetic compounds also include antagonists such as naloxone (Narcan), naltrexone (Trexan or ReVia or Vivitrol), and nalmefene (Revix), as well as partial agonists such as buprenorphine alone (Subutex) or, when combined with naloxone, (Suboxone) (4).
Oxycodone
Oxycodone has been used clinically since the early 1900s. It is combined with aspirin or acetaminophen for the treatment of moderate pain and is available orally without coan-algesic for severe pain (8). It is a popular drug of abuse, especially in controlled-release (CR) formulations that can be easily crushed and self-administered (intranasally or IV) for a potentially toxic, rapid “high” (9). Oxycodone is a semisynthetic compound derived from thebaine, with agonist activity primarily at mu receptors. Although structurally similar to codeine, it is pharmacodynamically comparable to morphine and has a 1:2 equivalence with morphine (10).
Codeine
French pharmacist Pierre-Jean Robiquet first discovered several natural products including codeine (11). Codeine is methyl morphine, with a methyl substitution on the phenolic hydroxyl group of morphine. It is more lipophilic than morphine and thus crosses the blood-brain barrier faster. It also has less first-pass metabolism in the liver, therefore, greater oral bioavailability than morphine, although it is less potent than morphine. A small part of codeine is metabolized to morphine via cytochrome 2D6 (4).
Meperidine
Meperidine is a phenylpiperidine and has a number of congeners. It is mostly effective in the CNS and bowel; however, it is no longer used for treatment of chronic pain owing to concerns regarding toxicity of its major metabolite and should not be used for greater than 48 hours or at doses greater than 600 mg/d. It has serotonergic activity when combined with monoamine oxidase inhibitors, which can produce serotonin toxicity (clonus, hyperreflexia, hyperthermia, and agitation) (12).
Pentazocine
Both parenteral and oral formulations of pentazocine were approved for marketing in the late 1960s. It is one of the initial “agonist-antagonist” medications. Pentazocine is a weak antagonist or partial agonist (it has a “ceiling effect,” plateau in maximal effect, contrasted with a full agonist) at the mu receptor and is also a kappa receptor partial agonist. In 1983, in order to block the euphorigenic effects of inappropriately injected pentazocine, pentazocine was manufactured in combination with naloxone (Talwin NX). Thus, if injected, this formulation would actually precipitate withdrawal in those with opioid dependence. Some data from the DAWN (Drug Abuse Warning Network) database indicated that abuse declined after this reformulation (13).
Hydromorphone
Hydromorphone is a more potent opioid analgesic than morphine. It is used for the treatment of moderate to severe pain and is excreted, along with its metabolites, by the kidney. It can be given intravenously, by infusion, orally, and per rectum, with low oral bioavailability. On a milligram basis, it is five times more potent than morphine when given orally, and 8.5 times as potent when given intravenously (14). A minor pathway for the metabolism of morphine to hydro-morphone has been identified (15).
Hydrocodone
Hydrocodone is a prescription drug frequently prescribed for a relatively minor (such as dental) pain. It is often used in combination with acetaminophen; thus, there can be hepatotoxicity associated with its abuse (8).
Methadone
Methadone is a synthetic long-acting full mu opioid agonist, active by parenteral and oral routes. It was first synthesized as a potential analgesic in Germany in the late 1930s and first studied for human use in the 1950s in the United States. It has been used primarily as a maintenance treatment for heroin addiction since the first research done in 1964 (16), and it was approved by the U.S. Food and Drug Administration (FDA) in 1972. Methadone is also used and is very effective in the treatment of chronic pain; however, it should not be used in opioid-naïve patients, due to the risk of respiratory depression. The l(R)-methadone enantiomer has up to 50 times more analgesic activity and also the potential to produce more respiratory depression than the d(S)-enantiomer. Both enantiomers have modest N-methyl-D-aspartate (NMDA) receptor antagonism. Methadone has a diphenylheptylamine chemical structure and consists of a racemic mixture of d(S)- and l(R)-methadone. Again, the l(R)-enantiomer is responsible for the majority of opioid effects as it is up to 50 times more potent than the d(S)-enantiomer (17).
Levo-alpha-acetylmethadol
LAAM is a synthetic, longer-acting (48-hour) congener of methadone that is also orally effective. LAAM was first studied in the 1970s for the treatment of heroin addiction (18) and approved in 1993 by the FDA (19) after a large multicenter safety trial. Postmarketing, after reports of prolonged QTc intervals on electrocardiogram, which can lead to torsade de pointes that may have been caused by LAAM, a black-box warning was added to the product label (20,21). LAAM remains approved for humans in the United States. However, no company is manufacturing LAAM at this time. As the new drug application for LAAM has not been withdrawn, LAAM could once again be made available in the United States (22).
Buprenorphine
Buprenorphine alone, and in combination with naloxone, was approved in 2002 by the FDA as an office-based sublingual treatment for heroin and opioid addiction (23–25) at the same time; buprenorphine was reclassified by the Drug Enforcement Administration from a Schedule V to a Schedule III drug (13). Buprenorphine alone, or when combined with naloxone, is a primarily MOP-r–directed partial agonist and is also a kappa partial agonist. The structure of buprenorphine is that of an oripavine with a C7 side chain, which contains a tert-butyl group. Norbuprenorphine is a major metabolite of buprenorphine in humans, with activity at the MOP-r as well (25).
The formulations of specific drugs are shown in Table 9-1.
TABLE 9-1 FORMULATIONS AND THEIR METHODS OF USE/ABUSE
Clinical Uses
Clinically used opioids (i.e., primarily MOP-r agonists) are used for pain control, and as maintenance medications for opioid addiction (i.e., methadone and buprenorphine). For minor pain, such as post-dental procedures, opioids such as hydrocodone are used. For more chronic or severe pain, opioids such as morphine may be used. There is the risk of iatrogenic addiction, respiratory depression, and also diversion of these medications. As a result, they must be dispensed cautiously. This caution, however, must be carefully balanced against the risk of undermedicating pain for each individual patient. When there is addiction to opioids, the approved long-acting opioid pharmacotherapies are available by prescription for detoxification and for maintenance, the latter having a well-proven long-term beneficial effect on patient health. Depot naltrexone (Vivitrol) was recently (October 2010) approved as a monthly IM injection for prevention of relapse following detoxification from opioid addiction.
Nonmedical Uses
Opioids are abused initially for their euphoria-inducing effects. There are patients who are prescribed opioids for pain treatment who go on to abuse and/or become dependent upon the medication, due to physiologic and/or psychological factors. Heroin, as mentioned, is not available for medical indications in the United States. Methadone and buprenorphine are sometimes diverted by those for whom it is prescribed, generally not for euphoria-inducing effects, but to prevent withdrawal.
Historical Features
Sumerian clay tablets (3000 BC) refer to the poppy; Sumerians named opium “gil” (“happiness”). The ancient Egyptians also cultivated poppies. Opium use was initially restricted and later became widely used. “Thebaine” is derived from the name for the Egyptian city “Thebes.” “Opium” may be a Greek-derived word (“opion” = poppy juice). Opium figures prominently in Greek mythology and was also mentioned in Hippocrates’ writings (460–377 BC). The ancient Roman author Pliny warned of the dangers of addiction to opium. In 1804, a young German pharmacist, Friedrich Sertürner, isolated morphine (which he named after Morpheus, the Greek god of dreams) (26). As a major development in opioid use and abuse, the hypodermic needle was perfected in 1853, which allowed for rapid analgesia, but also greater abuse morbidity. Diacetylmorphine was first synthesized as a semisynthetic analog in the 1870s by the Bayer company and marketed under the name “heroin.”
EPIDEMIOLOGY OF OPIOID ABUSE AND ADDICTION
The pharmacology of opioids is of particular relevance to the treatment of addictive disorders, given reports of increases in the abuse of illicit opioids, as well as illicit use of prescribed opioid medications (27). More than 100,000 people aged 12 to 25 initiated use annually between 1995 and 2002 (3). According to the Office of National Drug Control Policy publication of January 2008, more than 2.1 million teenagers in 2006 abused prescription drugs, and one-third of new abusers of prescription drugs in 2006 were 12- to 17-year-olds. Among 12- to 13-year-olds, prescription drugs are the drug of choice (28). According to the Substance Abuse and Mental Health Services Administration (SAMHSA) Treatment Episode Data Set, annual admissions to substance abuse treatment for primary heroin abuse increased from 228,000 in 1995 to 254,000 in 2005, with the percentage of primary heroin admissions remaining steady at about 14% to 15% of all substance abuse treatment admissions (7).
The majority of persons in treatment for heroin addiction are in methadone maintenance treatment. Also, currently, there are approximately 24,000 US physicians eligible to prescribe buprenorphine as office-based treatment to patients for treatment of opioid dependence (personal communication, Reckitt-Colman Co.). Eligibility requirements for physicians to use buprenorphine in treatment of addiction are as follows (as established by the SAMHSA DATA 2000; Drug Addiction Treatment Act 2000): completion of an 8-hour continuing medical education course; notification of the government of the intent to use buprenorphine for treatment of opioid-dependent patients; and having both the capacity to provide or refer patients for ancillary services and, as of December 2006, being in agreement to treat no more than 100 patients (increased from the original 30 maximum) at any one time in an individual or group practice (29,30).
Two cross-sectional studies conducted in New York City from 2000 to 2004 found that among new admissions to drug treatment, of those patients who had stopped injecting heroin as of 6 months prior to the study, the most common reasons for cessation included concerns about health and preference for the intranasal route (31).
The recent and major problem of opioid abuse and addiction is the illicit (nonprescription) use of prescription opioids, obtained illicitly or from family members or friends. As of 2007, 8th, 10th, and 12th grade students nationwide continued to show a decline in the proportions reporting illicit drug use. Less than 1% of students in these grades reported any use of heroin in 2007. However, the prevalence of other narcotic drug use reported for twelfth graders was 9.2% (32). In a National Institutes of Health: National Institute of Drug Abuse Research Report, published in 2001 and revised in 2005, it was reported that benzodiazepines and opioid pain relievers were the two most frequently reported prescription medications in drug abuse-related cases (33). In 2005, 30.1% of persons addicted to heroin sought treatment with methadone or buprenorphine maintenance, whereas only 19.9% of those addicted to illicitly used prescription opioids sought such pharmacotherapy (7).
Neurobiology, Mechanisms of Action, and Relationship to Abuse Liability
Abused opioids have primarily agonist effects at MOP-r receptors (encoded by the mu opioid receptor gene [OPRM1]) (17,34). MOP-r are members of the G-protein-coupled 7-transmembrane domain superfamily; they are coupled to Gi and Go proteins, and thus MOP-r agonists typically acutely result in a downstream decrease in adenylate cyclase activity (35).
Distribution in CNS and mediation of different functions
MOP-r are widely distributed in the CNS, and the constellation of their in vivo effects is mediated in different CNS areas (36–38). Thus, therapeutically desirable analgesic effects can be mediated in different sites including dorsal spinal cord and thalamus, whereas undesirable effects, such as respiratory depression, are thought to be mediated in the brainstem (39). Other regions involved in the classic processes of physiologic dependence/withdrawal to MOP-r agonists are thought to include the locus ceruleus and related centers (40,41). Classic rewarding effects of MOP-r agonists, of relevance to abuse and addiction, are likely mediated to a substantial degree in ventral and dorsal striatal areas and can depend (although not exclusively) (42) on downstream activation of the dopaminergic mesocorti-colimbic and nigrostriatal systems (43,44).
MOP-r Signaling Properties and Addiction/ Abuse Potential
A major underlying concept in the abuse potential of MOP-r agonists is their pharmacodynamic efficacy (i.e., their relative ability to stimulate downstream second messenger systems). In general, compounds with progressively greater efficacy (e.g., morphine or fentanyl-like compounds) have greater analgesic effects but also have greater abuse potential than partial agonists such as buprenorphine (35,45). Furthermore, other downstream effects of MOP-r agonist exposure are now postulated to be of relevance to the relative balance of therapeutic and undesirable effects of MOP-r agonists, including propensity to cause tolerance, or abuse potential. Major mechanisms of current interest are the relative propensity of compounds to cause MOP-r desensitization, and internalization, potentially related to their ability to stimulate the β-arrestin signaling pathways (46,47). For example, the main active heroin metabolite, morphine, results in lesser desensitization and internalization of receptors, compared to the endogenous neuropeptide ligands, or methadone (48). Thus, methadone maintenance can be used effectively for extended periods without the development of further tolerance (or progressively greater methadone dose requirements) (49). By contrast, abused heroin (through its main metabolite, morphine), or abused prescription opioids, may result in progressive cycles of dependence and tolerance, secondary to a lesser recruitment of endogenous MOP-r desensitization/internalization mechanisms (50).
PHARMACOKINETICS OF SPECIFIC DRUGS
It is beyond the scope of this chapter to provide a comprehensive table of dosing equivalents. There are a number of excellent reviews on this topic (4,8,51).
Heroin (Diacetylmorphine) Pharmacokinetics
Heroin is a very efficient prodrug that is more water soluble and more potent than morphine (52). It is synthesized from morphine by acetylation at both the 3 and 6 positions and metabolized in humans to active opioid compounds first by deacetylation to the active 6-monoacetylmorphine (6-MAM), and then by further deacetylation to morphine (52). Well-designed studies of heroin pharmacokinetics in humans have been performed (53–56). Heroin has an average half-life in blood of 3 minutes after intravenous administration; the half-life of 6-acetylmorphine in humans appears to be 30 minutes (53).
The use of intranasal, intramuscular, and subcutaneous heroin all produce peak blood levels of heroin or 6-acetylmorphine within 5 minutes; however, intranasal use has about half the relative potency of parenteral routes (54). Further research needs to be carried out on the role of 6-acetylmorphine (administered directly) in relation to the pharmacokinetics of parenteral heroin, particularly its onset of action and potency as compared with morphine. Most of the enzymes involved in the metabolism of opioids are part of the P450 microsomal enzyme system, though heroin and morphine are also biotransformed outside this system.
Morphine Pharmacokinetics
Morphine is largely selective for MOP-r and is considered by most physicians in the United States the drug of choice for the treatment of cancer pain. Morphine is biotransformed mainly by hepatic glucuronidation to the major but inactive metabolite morphine-3-glucuronide (M3G) and the biologically active M6G compound (57).
The pharmacokinetics of morphine and its metabolites vary, depending on the route of administration. Its favorable safety profile is due in large part to its pharmacokinetic profile. The oral bioavailability varies, from 35% to 75%, with a plasma half-life ranging from 2 to 3.5 hours. The half-life is less than the time course of analgesia, which is 4 to 6 hours, thus reducing accumulation. Morphine is metabolized mostly in the liver, with prolonged clearance because of enterohepatic cycling with oral dosing (58). In the setting of chronic liver disease, morphine oxidation is more affected than is glucuronidation. Use of lower doses or longer dosing intervals is recommended to minimize the risk of accumulation of morphine when chronic liver disease is present, particularly with repeated dosing. At 24 hours, more than 90% of morphine has been excreted in urine. M6G elimination seems to be closely tied to renal function, so accumulation of metabolites can occur. With renal compromise, less than 10% of morphine and its metabolites are excreted in feces; therefore, morphine should be used with great caution in patients with renal disease (unlike methadone, which can be given relatively safely in these patients: see Methadone Pharmacokinetics). The higher sensitivity of older adults to the analgesic properties of morphine may be related in part to altered pharmacokinetics (59,60).
Oxycodone Pharmacokinetics
The onset of action of oxycodone begins after 1 hour of PO (oral) administration, and in the CR form lasts for approximately 12 hours, with a plasma half-life of 3 to 4 hours for the immediate release (IR). Stable plasma levels are achieved within 24 hours. Oral bioavailability ranges from 60% to 87%, with 45% protein bound. Oxycodone is mostly metabolized in the liver, with the remainder as well as the metabolites metabolized in the kidneys. The two main metabolites are oxymorphone, which is also a potent analgesic, and the weaker analgesic noroxycodone, which is its major metabolite (10,61). In terms of protein binding and lipophilicity, oxycodone is similar to morphine, with slightly longer half-life and greater bioavailability. Unlike morphine, oxycodone is metabolized mostly by the cytochrome enzyme CYP2D6, while morphine in humans is primarily glucuronidated (62).
Codeine Pharmacokinetics
Codeine has a high oral-parenteral effect, owing to low first-pass metabolism in the liver. Metabolites are mostly inactive and excreted in the urine, with about 10% demethylated via CYP2D6 to morphine, which is mostly responsible for the analgesic effect of codeine, as codeine itself has very low affinity for opioid receptors. Genetic variations in this enzyme system may result in lower production of M6G. The allelic variants have different frequency in different ethnic groups, and can affect the depth of analgesia. Repeated doses of codeine may result in the accumulation of the active metabolite M6G in patients with renal disease.
Meperidine Pharmacokinetics
Onset of analgesia begins with the oral route after 15 minutes, with peak in 1 to 2 hours, which is close to peak level in plasma, with duration of about 1.5 to 3 hours (4). It is absorbed by all routes, but intramuscular administration results in a less reliable peak plasma level after 45 minutes, with wide range of plasma concentrations. After oral administration, about 50% of meperidine enters circulation without first-pass metabolism, with peak at 1 to 2 hours. Meperidine is mostly metabolized in the liver, with half-life of about 3 hours. Cirrhosis leads to increased bioavailability and half-life of both meperidine and normeperidine. Sixty percent of meperidine is protein bound and little is excreted unmetabolized (12).
Pentazocine Pharmacokinetics
Pentazocine is a mixed agonist–antagonist (with intermediate efficacy effects at both MOP-r and KOP-r) that can be given intramuscularly or orally, but is not currently available in the oral formulation. It can cause psychotomimetic effects (likely due to its KOP-r actions) and therefore has a very limited role in the treatment of chronic pain. Its duration of action is 3 to 6 hours. Its peak effect is at 0.5 to 1 hour when given intramuscularly and 1 to 2 hours when given orally (8). Sixty percent of the drug is bound to protein. Pentazocine is metabolized by the liver via oxidative and glucuronide conjugation with an extensive first-pass effect. When administered orally, the bioavailability of pentazocine is about 10%, except in patients with cirrhosis, which increases bioavailability to 60% to 70%. The drug half-life is 2 to 3 hours. Small amounts of unchanged pentazocine are excreted with urine (8).
Hydromorphone Pharmacokinetics
Hydromorphone is shorter acting than morphine. It is derived from morphine, although it may also be produced in the body in small amounts by N-demethylation of hydrocodone. It has an oral bioavailability of 30% to 40%, with an analgesic onset after 10 to 20 minutes, which peaks at about 30 to 60 minutes and persists for about 3 to 5 hours. The oral-parenteral ratio is about 5:1, with an equivalency of 1.5 mg of hydrocodone to 10 mg morphine (63).
Hydrocodone Pharmacokinetics
Hydrocodone has a half-life of 2 to 4 hours, with a peak effect at 0.5 to 1 hour. Its duration of action is 3 to 4 hours (8). Codeine may show up as trace quantities of hydrocodone in urine testing as up to 11% of codeine is metabolized to hydrocodone (64), which could be misinterpreted as hydrocodone abuse.
Methadone Pharmacokinetics
Methadone, as used in the United States, is a racemic compound; the l(R)-enantiomer is the active enantiomer and the other d(S)-enantiomer is the inactive enantiomer. Both enantiomers are weak NMDA receptor antagonists; therefore, racemic methadone, retards and attenuates the development of opioid tolerance (65). Methadone meets the two important criteria for a medication for the treatment of addiction: high systemic bioavailability (>90%) with oral administration and long apparent half-life with long-term administration in humans (66). The medical safety of long-term methadone maintenance treatment has been well studied (67).
Oral methadone has a rapid absorption but a delayed onset of action, with peak plasma levels achieved by 2 to 4 hours and sustained over a 24-hour dosing period (36,66,68,69). Moreover, the mean plasma apparent terminal half-life of racemic d,l-methadone in human subjects is around 24 hours (66). The l-enantiomer has a half-life of 36 hours (65,70,71). Biotransformation of methadone is accelerated in the third trimester; therefore, methadone dose may need to be increased in the final stages of pregnancy (72).
When taken on a chronic basis, methadone is stored and accumulated mostly in the liver (68,73). Methadone plasma levels are relatively constant because of slow release of unmetabolized methadone into the blood, which extends the apparent terminal half-life. Methadone is more than 90% plasma protein bound both to albumin and globulins (72,74). These properties help explain why methadone maintenance treatment is effective as a once-daily, orally administered pharmacotherapy for heroin addiction (16), unlike heroin and morphine, both of which have a relatively rapid onset and offset of effect and short duration of action.
Owing to the long half-life of methadone, when beginning long-term methadone maintenance treatment (usually starting with a 20- to 40-mg daily dose), escalation of dose exceeding the rate of development of tolerance can result in accumulation, with sedation and even respiratory depression. Thus, dosages must be increased slowly, usually by 10 mg every 4 to 7 days. In some patients, doses in the appropriate range (e.g., 80 to 150 mg/d) do not result in either clinical improvement or in apparent therapeutic plasma levels of 250 to 400 ng/mL, and this may be due to “rapid metabolism” related to individual genetic differences of the cytochrome P450– enzyme or p-glycoprotein–related transporter systems (69,75,76), the latter also potentially related to blood-brain barrier passage of methadone. Methadone levels in the cerebrospinal fluid peak 3 to 8 hours after methadone dosing (77,78).
Methadone is biotransformed in the liver by the cytochrome P450-related enzymes (primarily by the CYP3A4 and, to a lesser extent, the CYP2B6, CYP2D6, and CYP1A2 systems) to two N-demethylated biologically inactive metabolites, which undergo additional oxidative metabolism (17,65). Methadone and its metabolites are excreted in nearly equal amounts in urine and in feces (78–82). In patients with renal disease, methadone can be cleared almost entirely by the GI tract, reducing potential toxicity by preventing accumulation (80–82
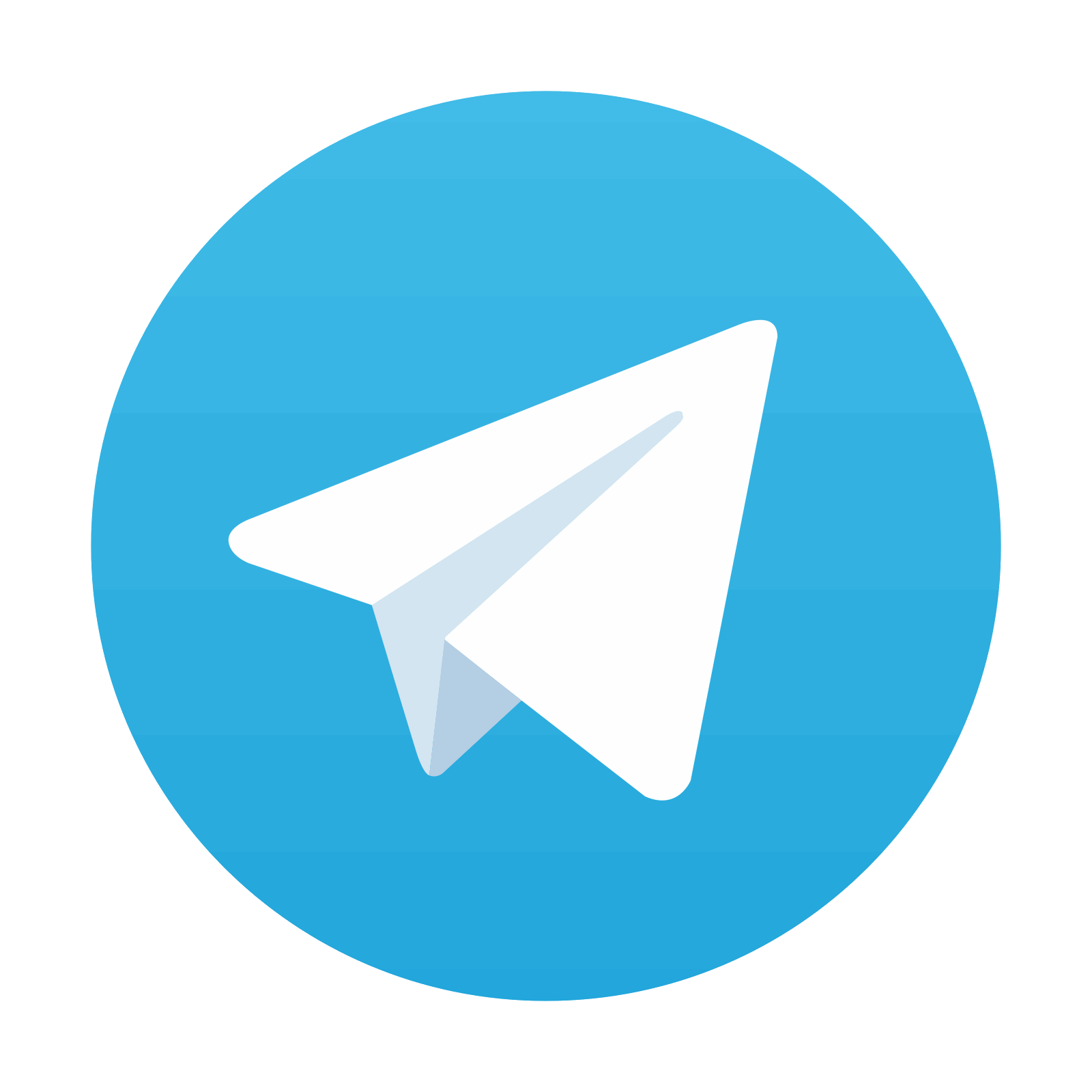
Stay updated, free articles. Join our Telegram channel

Full access? Get Clinical Tree
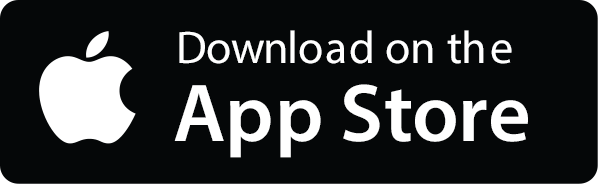
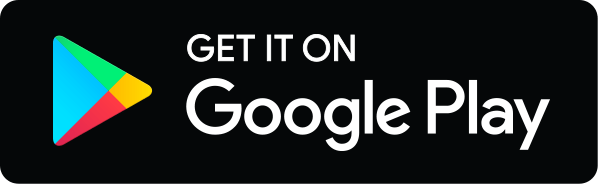