10
CHAPTER OUTLINE
Stimulants are a class of drugs that stimulate activity in the central and sympathetic peripheral nervous systems, chiefly by enhancing neurotransmitter activity at catecholaminergic synapses.
Stimulants include both naturally occurring plant alkaloids, such as cocaine (Fig. 10-1), ephedra, and khat, and more than a dozen synthetic compounds, such as the amphetamines and methylphenidate. Most of these are variants of the basic phenethylamine chemical structure, which is shared by the endogenous catecholamine neurotransmitters norepinephrine and dopamine (Fig. 10-2). The wakefulness-promoting agents modafinil and its (R)-isomer, armodafinil, have a mechanism of action similar to that of stimulants, but a different chemical structure and reduced propensity for abuse (1). They are not considered in this chapter.
All stimulants share the same range of psychological and physiologic effects, while differing in potency and pharmacokinetic characteristics. Caffeine, the most widely used stimulant, is considered separately in Chapter 11. 3,4-Methylenedioxymethamphetamine (MDMA, “Ecstasy”), a structural analog of methamphetamine with both stimulant and hallucinogenic characteristics, is considered separately in Chapter 14.
FORMULATIONS AND METHODS OF USE AND ABUSE
Plant-Derived Stimulants
Several naturally occurring, plant-derived stimulants are widely available for traditional oral use in many areas of the world. These include cocaine (in South America), ephedra (in North America and East Asia), and khat (in East Africa and Arabia). Such use often is culturally sanctioned and may not be associated with abuse or dependence. Use of more potent formulations (e.g., the extracted active chemical) or more rapidly acting routes of administration has significant abuse potential and is illegal even where oral formulations are allowed.
Cocaine
Cocaine is an alkaloid with a tropane ester chemical structure (see Fig. 10-1) similar to that of scopolamine and other plant alkaloids. It occurs in leaves of the coca bush, Erythroxylum coca, which grows at altitudes of 1,500 to 6,000 feet in the Andean region of South America (chiefly Colombia, Peru, and Bolivia). The leaf contains cocaine (0.2% to 1%) and more than a dozen other tropane alkaloids (such as benzoylecgonine, methylecgonine, ecgonine, and cinnamoylcocaine), most of which are of unknown pharmacologic activity. Another Erythroxylum species, E. novogranatense, contains lesser amounts of cocaine and greater levels of other alkaloids (1a). Cocaine exists as two stereoisomers: naturally occurring (−)-cocaine and (+)-cocaine, which has less affinity for the dopamine transporter and is relatively inactive in vivo because of its very rapid metabolism by butyrylcholineesterase (2).
FIGURE 10-1 Chemical structures of cocaine, mazindol, and methylphenidate.
About 149,000 hectares of coca were cultivated in Bolivia, Colombia, and Peru in 2010. This resulted in an estimated production of 800 to 1,000 metric tons of cocaine (3), most destined for export. Domestic use of oral cocaine is legal in these countries, usually as coca tea or by chewing the leaves. Coca leaves typically are chewed in conjunction with lime or plant ash, which alkalinizes the saliva and thus enhances absorption of the cocaine. Cocaine is legally available in the United States only as a 4% or 10% injectable solution (or powder for reconstitution) or viscous liquid for use as a local or topical anesthetic. Legal cocaine preparations rarely are diverted for misuse.
Illicit cocaine is smuggled into the United States specifically for abuse purposes from its countries of origin. The average retail purity was 50% in the United States and 32% in Western and Central Europe (3).
Preparation of illicit cocaine begins with crushing the coca leaves and heating them in an organic solvent (often kerosene) to extract and partially purify the cocaine (4). After several more extraction and filtering steps, the coca paste (now 80% to 90% pure) is heated in an organic solvent (often ether or acetone) with a concentrated acid to convert it to a salt form. The salt is readily converted back to the base by heating it in an organic solvent at basic pH. This process is known as “freebasing,” and was practiced by cocaine users during the 1980s, before cocaine base (or “freebase”) was widely available on the retail street market. “Crack” as a street name for base cocaine reportedly derives from the crackling sound made during this process.
Cocaine is available for street use in two forms: base and salt (5,6). These have different physical properties, which favor different routes of administration. The base has a relatively low melting point (98°C) and vaporizes before substantial pyrolytic destruction has occurred. This allows cocaine base to be smoked, though the majority of the cocaine may be in the form of small particles (<5 μm) that reach the alveoli, rather than true cocaine vapor (7). Cocaine base is relatively insoluble in water (alcohol:water solubility ratio of 100:1), making it difficult to dissolve for injection purposes. In contrast, cocaine salt does not melt at less than 195°C, so heating it for smoking results in destruction of most of the cocaine. However, cocaine salt is highly water soluble (alcohol:water solubility ratio of 1:8), making it easy to dissolve for injection purposes and facilitating absorption across mucous membranes. Regardless of the chemical form or route of administration, the cocaine molecule exerts the same actions once it reaches the brain or other target organ (5).
Adulterants are added (i.e., the cocaine is “cut”) to enhance dealer profits. These include both inert fillers that look like cocaine (such as dextrose, lactose, mannitol, or starch) and active chemicals that either mimic the local anesthetic effect of cocaine (such as benzocaine, lidocaine, or procaine) or provide some psychoactive effect (such as ephedrine, amphetamine, caffeine, or phencyclidine [PCP]) (4,8). The veterinary antihelminthic agent levamisole is increasingly common as a cocaine adulterant, found in more than 60% of analyzed street cocaine samples in the United States (9). Cocaine adulterated with levamisole is associated with serious side effects, including agranulocytosis and cutaneous vasculitis. Street cocaine may also contain contaminants from the preparation process (such as benzene, acetone, or sodium bicarbonate) (8).
Ephedra
Ephedrine and pseudoephedrine are naturally occurring alkaloids with a phenethylamine chemical structure (see Fig. 10-2) that are found in several Ephedraceae species (especially Ephedra sinica, E. equisetina, and E. gerardiana) (6,10). Ephedra is a preparation of dried young branches of Ephedra species, typically containing 1% to 3% ephedrine. This may be converted into a capsule, tincture, liquid extract, or tea. Ephedra products are widely used in East Asia and North America; they appear in the pharmacopoeias of China, Japan, and Germany.
FIGURE 10-2 Chemical structures of endogenous catecholamine neurotransmitters (dopamine, norepinephrine) and phenethylamine stimulant drugs.
Ephedra products often are advertised as legal versions of or alternatives to the more strictly regulated manufactured stimulants. They may appeal to consumers as safer than synthetic stimulants because they are “natural” or “herbal.” Synthetic ephedrine and pseudoephedrine also are available as tablets or capsules (see below). Ephedra alkaloids have the same range of psychological and physiologic effects as do cocaine and amphetamines. There is limited evidence of their efficacy for weight loss in obese individuals (11). Ephedra use has been associated with severe cardiovascular and central nervous system (CNS) effects, including death (12), leading to its banning from the US market in 2006.
Khat
Khat is the common term for preparations of the Catha edulis plant, which is native to East Africa (Sudan to Madagascar) and the southern Arabian peninsula (Yemen) (10,13,14). Fresh khat leaves contain at least two stimulant alkaloids with phenethylamine chemical structures: cathinone (present at 1% to 3%) and cathine (norpseudoephedrine).
Pure cathinone is a Schedule I controlled substance; cathine is in Schedule IV. Cathinone displays neuropharmacologic potency similar to amphetamines (15). Recreational use of potent synthetic cathinone congeners such as mephedrone, methylone, and 3,4-methylenedioxypyrovalerone, often marketed as “bath salts,” has increased markedly around the world in the past decade (see “Synthetic Stimulants” below and “Bath Salts” sidebar).
Khat use has been a widely accepted social custom for centuries, apparently predating the use of coffee (caffeine). The leaves are used in the same way as coca leaves in South America, that is, chewed and kept in the cheek for several hours. Less often, the leaves are brewed into tea or crushed with honey to make a paste. Moderate use reduces fatigue and appetite. Compulsive use may result in manic behavior or psychotic symptoms such as paranoia or hallucinations. The extent of abuse or dependence is unclear. Khat loses much of its potency within 2 days of harvesting, as cathinone is converted to the much less potent cathine. Some khat use is found among immigrant communities in Europe, but there appears to be negligible use of khat in the United States. Methcathinone is widely abused in Russia and the Baltic area.
Synthetic Stimulants
More than a dozen synthetic stimulant medications are legally available in the United States, by either prescription (Table 10-1) or over the counter (OTC) (Table 10-2). Most represent variations on the basic phenethylamine structure (see Fig. 10-2). Common trade and street names, controlled substance scheduling, clinical uses (U.S. Food and Drug Administration [FDA] approved and otherwise), and typical doses are listed in Tables 10-1 and 10-2. All legal stimulants, other than cocaine, are sold for oral use in a tablet, capsule, or liquid form.
TABLE 10-1 STIMULANTS AVAILABLE BY PRESCRIPTION IN THE UNITED STATES
aNot labeled for this indication by the U.S. Food and Drug Administration.
ADHD, attention deficit/hyperactivity disorder; CSA, the U.S. Controlled Substances Act.
TABLE 10-2 STIMULANTS AVAILABLE AS OVER-THE-COUNTER PREPARATIONS IN THE UNITED STATES
Several stimulants are available in extended- or sustained-release formulations (16,17), chiefly prescribed for the treatment of attention deficit disorder (ADD). Methylphenidate is also available as a transdermal patch, which releases the drug at a rate of 1.1 to 3.3 mg/h, depending on the patch size (18). Amphetamine is available as a prodrug, lisdexamfetamine, consisting of d-amphetamine coupled to the amino acid L-lysine (19,20). The active drug is formed as the inactive lysine is hydrolyzed off in the intestines and liver. All these formulations have two theoretical advantages over conventional immediate-release formulations: improved patient compliance and effectiveness because of longer duration of action and reduced abuse liability because of slower onset of action and weaker peak subjective effects (21).
Some OTC stimulants also are available in aerosolized formulations for nasal inhalation (insufflation) for use as decongestants. Phenylephrine is available as a sterile solution for parenteral administration to treat hypotension.
Synthetic stimulants typically are abused by the oral or intravenous route. Amphetamines, especially highly pure crystallized methamphetamine (“ice”), may be used intranasally or smoked, as is cocaine. Some abused synthetic stimulants, such as methylphenidate, are diverted from legitimate sources and thus are of known content and purity. If oral administration is not intended, the original tablet or capsule may be crushed or opened to allow the drug to be taken intranasally or mixed with water for injection. In contrast, amphetamines, especially methamphetamine, usually are synthesized in clandestine laboratories directly for illicit use. This can be done with standard chemical reactions applied to legally available precursors. For example, methamphetamine (desoxyephedrine) can be made by reducing ephedrine or pseudoephedrine. For this reason, retail purchases in the United States of products containing ephedrine or pseudoephedrine are limited to 3.6 g/d and 9 g/mo and require photographic identification. Stimulants with a phenylisopropylamine structure (such as amphetamine, methamphetamine, ephedrine, pseudoephedrine, and phenylpropanolamine) have a chiral (stereoisomeric) center at the alpha-carbon atom (see Fig. 10-2), and so exist in two (or more) stereoisomer forms that differ in pharmacodynamic and pharmacokinetic properties (22,23). The d– or S-(+) isomer generally has three to five times the CNS activity and about one-third the half-life of the l– or R-(−) isomer. The l-isomers have more peripheral alpha-adrenergic activity. For example, d-methamphetamine is a potent CNS stimulant, whereas l-methamphetamine (l-desoxyephedrine) has been used as a decongestant (as in the Vicks nasal inhaler) (24). Methylphenidate exists in four stereoisomeric forms, of which the d-threo enantiomer is the active one (25).
The recreational use of synthetic stimulants not related to prescription medications has increased substantially in recent years. These compounds are typically synthetic analogs of cathinone (stimulant found in the khat plant) and are classified in Schedule I as having high abuse potential and no legitimate medical use. The products are purchased over the Internet or at retail outlets not typically associated with illicit drugs and marketed with innocuous names (e.g., “bath salts,” “plant food”) as “legal” alternatives to cocaine and methamphetamine (26). Synthetic cathinones exert potent amphetamine-type stimulant effects and can cause serious cardiovascular and neurologic side effects requiring emergency medical care (see “Bath Salts” sidebar for more details).
Clinical Uses
Cocaine is used clinically only as a local or topical anesthetic, chiefly for eye, ear, nose, or throat surgery or procedures (27). Other prescription stimulants generally are used for one of the several FDA-approved indications: ADD in both children (28,29) and adults (30–32), narcolepsy and excessive daytime sleepiness (33,34), and appetite suppression to promote weight loss in exogenous obesity (35,36) (see Table 10-1).
OTC stimulants generally are used for decongestion and bronchodilation in the treatment of asthma, upper respiratory infections, allergic rhinitis, sinusitis, or bronchitis and for appetite suppression to promote weight loss in exogenous obesity (both of which are FDA-approved indications) (see Table 10-2). Parenteral phenylephrine also is approved by the FDA as an adjunct to prolong the duration of spinal anesthesia, to terminate paroxysmal supraventricular tachycardia (probably indirectly by stimulation of arterial baroreceptors), and for immediate, short-term treatment of hypotension (especially when due to anesthesia, drugs, or hypersensitivity reactions).
In addition to their FDA-approved indications, oral stimulants have a long history of accepted clinical use for other indications (37,38). Amphetamines and methylphenidate are used as quick-acting (2- to 3-day), short-term antidepressants in persons who are elderly, medically ill, or HIV infected or in those with neurologic conditions such as stroke or traumatic brain injury, especially those who cannot tolerate the side effects of standard antidepressants (38,39). Such patients may exhibit apathy, fatigue, and psychomotor retardation, rather than a full-blown classic depressive syndrome. Often, it is unclear whether the beneficial effect of stimulants in such patients is due to the drug’s activating effects or to true antidepressant actions (40). Stimulants also have been used to augment the response to standard antidepressants (41). Amphetamines have been used to improve recovery after stroke, but their efficacy is questionable (42). Amphetamines, methylphenidate, and mazindol have been used to potentiate opiate analgesia and to counteract opiate-induced sedation and respiratory suppression, thus allowing larger doses of opiates to be used (37,38). Cocaine has been used for this purpose as part of Brompton cocktail (with alcohol and an opiate) in the treatment of cancer pain. Ephedrine and phenylephrine still are used parenterally to counteract hypotension associated with spinal anesthesia (especially in obstetrical and urologic surgery) (6). Most other clinical uses of stimulants for their pressor effect have been superseded by more selective agents.
There is no evidence that medical use of stimulants at therapeutic doses in appropriately diagnosed patients leads to stimulant abuse or increases the risk of serious adverse events, although there are few data from long-term, controlled trials (32,38,43). Prospective, longitudinal studies of children receiving stimulant treatment for attention deficit/ hyperactivity disorder (ADHD) find no increased risk of developing substance abuse (44).
Nonmedical Use, Abuse, and Dependence
Oral stimulants (both prescription and OTC) are widely used in work, school, military, and sports settings, often without medical supervision, for their alerting, antifatigue, sleep-suppressing, and performance-enhancing properties (45,46). Nonmedical use of prescription stimulants in the general US population has increased substantially since the early 1990s (47), whereas use in the U.S. military has declined more than 80%, largely as the result of a comprehensive antidrug policy that includes mandatory urine drug testing. In 2005, 1.9% of U.S. military personnel had used cocaine, and 1.4% had used other stimulants within the preceding 12 months (48)—substantially less than among the general population of similar age and gender. Use by other occupational groups, such as long-distance truck drivers and night-shift workers, remains problematic.
The stimulants’ antifatigue and sleep-suppression effects have been well demonstrated in laboratory and field studies. Enhancement of cognitive and psychomotor performance is more difficult to demonstrate in controlled studies and occurs more robustly in persons who already are fatigued or sleep deprived. Stimulants are used by athletes to improve performance, especially in endurance sports such as cycling, which require anaerobic exercise, although there is little published evidence of their efficacy (49–51). Stimulants of all types (illicit, prescription, and OTC) are banned by the World Anti-Doping Agency and many other sports organizations (52).
All stimulants have a potential for misuse, abuse, and dependence, varying only in their potency. Cocaine, amphetamine, and methamphetamine have high abuse potential, as reflected in their placement in Schedule II. Community-based interview surveys suggest that up to one in six persons who use cocaine and one in nine who use prescription stimulants for other than medical purposes will become dependent (53). Even higher rates are found in treatment-seeking populations: up to four-fifths of those who have used cocaine several times and up to half of those who have used amphetamines become dependent (54). Heavier users and those who use the intravenous and smoked forms are more likely to become dependent than are lighter users or those who use the intranasal and oral forms (54–56). Even lower-potency stimulants in Schedule IV, such as pemoline and phentermine, or OTC stimulants, such as ephedrine, pseudoephedrine, and phenylpropanol-amine, have been misused and abused (57).
Studies of psychoactive drug use and abuse/dependence by pairs of fraternal (dizygotic) and identical (monozygotic) twins suggest that there is a genetic influence both on initiation and use of stimulants and on stimulant abuse and dependence (58,59). Findings are best explained by a single genetic factor influencing illegal drugs (as distinct from legal drugs such as alcohol, tobacco, and caffeine), which may account for about three-fourths of the variance in cocaine dependence and one-fourth of the variance in amphetamine dependence. There do not appear to be significant gender differences in genetic influence. Efforts to identify a specific gene or genes responsible for stimulant dependence have not been successful. Many candidates have been identified in genome-wide association studies, but none has been robustly replicated (60).
The greater abuse liability of intravenous and smoked (as opposed to oral and intranasal) routes of administration is considered owing to their faster rate of drug delivery to the brain (6 to 8 seconds, in the case of smoking), resulting in a faster onset of psychological effects (61,62). This faster onset is associated with a more intense pleasurable response and greater abuse liability (the so-called “rate hypothesis” of psychoactive drug action) (21).
Stimulants are used in a variety of patterns (63,64). “Binge” use involves short periods of heavy use (e.g., on weekends or after payday), separated by longer periods of little or no use. Others may use frequently for an extended period until their finances are exhausted or their access to drug is interrupted. A small number of users may use low doses daily without dose escalation over time. Some of these users may be self-medicating an underlying neuropsychiatric disorder such as ADD or narcolepsy. Typical cocaine doses are 12 to 15 g orally (coca leaf chewing), 20 to 100 mg intranasally, 10 to 50 mg intravenously, and 50 to 200 mg smoked.
High rates of stimulant abuse have been documented in persons involved with the criminal justice system. A national survey of 6,982 local jail inmates in 2002 found that 20.7% reported using cocaine in the month before their offense; 10.6% had used cocaine at the time of their offense (65). The figures for use of other stimulants were 11.4% and 5.2%, respectively. These occurrence rates suggest an association between stimulant use and crime, violence, and aggression. There are several potential causal mechanisms for this association, including behaviors associated with the illegal manufacture, distribution, and marketing of drugs; behaviors associated with users obtaining drug (or the money to buy drug); or a direct pharmacologic effect of stimulants on behavior (66–68). The association also may reflect the co-occurrence of stimulant use and antisocial personality disorder (which is associated with high rates of criminal behavior).
Stimulant use is associated with agitation and aggressive behavior (69). Low doses increase aggressive behavior in human experimental laboratory models of aggression (e.g., willingness to administer electric shock) (67). Numerous case reports document violent behavior due to stimulant-induced irritability, paranoia, or frank psychosis (70).
Unintended use of cocaine may occur in persons who swallow the drug in their possession to avoid arrest or prosecution (“body stuffers”) or who swallow large quantities to transport it without detection by law enforcement authorities (“body packers,” “mules”) (71). The drug may be wrapped in plastic bags, balloons, condoms, paper, aluminum foil, or a combination. If the wrapper fails, the carrier may be exposed suddenly to large doses of cocaine in the gastrointestinal tract, resulting in severe acute cocaine intoxication.
Stimulants often are used in combination with other drugs, either concurrently or sequentially. Concurrent use of a stimulant and an opiate, sometimes in the same injecting syringe, is considered by many users to provide a qualitatively better subjective effect (“high”) than either drug alone (72,73). Concurrent intravenous use of cocaine plus heroin is termed speedballing. Combined use of oral amphetamine plus an oral opiate (such as codeine) also is common. Human experimental studies suggest that the acute psychological effects of cocaine are somewhat enhanced with concurrent administration of cocaine and an opiate (74). Other drugs sometimes used concurrently with smoked cocaine include PCP, marijuana, or tobacco (75). CNS depressant drugs, such as alcohol, benzodiazepines, opiates, and marijuana, often are used after cocaine use to temper unpleasant effects of cocaine intoxication (such as anxiety, paranoia, restlessness) and/or to relieve symptoms of cocaine withdrawal.
Naturally occurring plant alkaloids have been used for their CNS stimulant properties for thousands of years (6). Chinese medicine has used the herbal preparation Mahuang (ephedra) for at least 5,000 years. Chewing of coca leaves has been prevalent in the Andean regions of South America for at least 2,000 years (76). Coca leaves and pottery images of figures with bulging cheeks (presumably wads of coca leaf) have been found in 1,400-year-old Peruvian burial sites. The Spanish conquerors of South America found coca leaf chewing common in the Andean regions and noted its association with increased energy and decreased need for food and sleep. They did not discourage the practice, which continues to this day and is legal in Bolivia and Peru (77).
Coca received little attention in Europe until the second half of the 19th century. In 1860, a German graduate student, Albert Niemann, isolated cocaine as the active ingredient of coca leaf. This discovery helped generate the popularity of cocaine-containing products throughout Europe and North America. Cocaine-containing wines, such as Vin Mariani (containing 6 to 8 mg of cocaine per ounce), were widely advertised and endorsed by prominent political and cultural figures. A nonalcoholic beverage (containing 4.5 mg of cocaine per 6 ounces) was introduced in 1886 and quickly became one of the world’s most popular soft drinks: CocaCola. A fluid extract of coca for medical use (containing 0.5 mg of cocaine per mL) appeared in the U.S. Pharmacopeia in 1882. The first specific use of cocaine in medicine came in 1884, when the German ophthalmologist Koller discovered its efficacy as a local anesthetic during surgery. In the same year, Sigmund Freud published his monograph, Uber Coca, describing the first systematic study of cocaine’s psychological effects (albeit with a sample of one, himself) and suggesting its use as a treatment for morphine addiction.
With widespread use of cocaine came increasing reports of adverse effects. The first report of cocaine-associated cardiac arrest and stroke was published in 1886. By 1903, cocaine had been removed from Coca-Cola. In 1914, the Harrison Narcotic Act banned cocaine from OTC medications, beverages, and foods in the United States, restricting its use to prescription drugs. For the next 50 years, cocaine remained largely out of public view and medical attention, except for limited use as a local anesthetic.
Synthetic stimulants first appeared with the synthesis of amphetamine in 1887 (by Edeleau) and of methamphetamine in 1919. These attracted little attention until amphetamine became popular as an OTC bronchodilator (in the Benzedrine inhaler) in the early 1930s. By 1933, its CNS stimulant properties were recognized, leading to its use for weight loss, narcolepsy, depression, and childhood hyperactivity. Amphetamine’s advantages also were recognized by stimulant abusers. It largely replaced cocaine in illicit use because of its low cost, ready availability, and longer duration of action. This growing abuse pattern led to a switch in 1937 from OTC to prescription-only status. During World War II, amphetamine was widely used by the Allied and Axis countries to enhance the performance of troops and factory workers. After the war, widespread abuse in Japan and Sweden of large leftover stockpiles led to tight restrictions on amphetamine manufacture and dispensing. In response to increasing rates of intravenous abuse of amphetamine extracted from Benzedrine inhalers, the U.S. FDA banned the inhalers in 1959. With passage of the Controlled Substances Act in 1970, cocaine, amphetamine, and methamphetamine were placed in Schedule II because they have high potential for abuse and accepted medical use only with severe restrictions. As recognition of their abuse potential grew, the number of prescription stimulants available in the United States declined from 65 (marketed by 40 different companies) in 1970 to 8 (marketed by six companies) in 1995 (38).
There are substantial geographic and sociodemographic differences in the epidemiology of stimulant use (3,78). In 2011, there were an estimated 17 million cocaine users worldwide, representing 0.4% of the 15- to 64-year-old population. About one-quarter (27%) were in North America (4.6 million; 1.5% prevalence), where use declined about one-third over the prior 5 years. In contrast, use is increasing in West and Central Europe (4.0 million users, 1.2%), South America (3.3 million users, 1.3%), Central America and the Caribbean (0.36 million users, 0.7%), West, Central, and Southern Africa (2.2 million users, 0.7%), and Oceania (0.37 million users, 1.5%). Cocaine use is also increasing, but remains at low prevalence, in East and Southeast Europe
(0.56 million, 0.2%), North and Eastern Africa (0.03 million, 0.02%), and Asia (1.3 million, 0.05%). In 2011, there were an estimated 33.7 million (0.7% prevalence) global nonmedical users of synthetic amphetamine-type stimulants (primarily amphetamine and methamphetamine). Such misuse is most prevalent in Oceania (0.5 million, 2.1%), North America (4.0 million, 1.3%), Central America (0.33 million, 1.3%), Africa (5.1 million, 0.9%), the Caribbean (0.2 million, 0.8%), Asia (19.1 million, 0.7%), and West and Central Europe (2.2 million, 0.7). There is relatively less use in East and Southeast Europe (0.87 million, 0.4%) and South America (1.4 million, 0.5%). These patterns of stimulant use probably reflect availability and access, because the only source of cocaine is the Andean region of South America, whereas amphetamines are readily synthesized anywhere.
Oral use of cocaine has a long cultural tradition and is legal in Bolivia and Peru, where surveys estimate a lifetime prevalence of coca leaf use up to 90% and 30%, respectively (77). Purified cocaine, suitable for intravenous, smoked, or intranasal administration, is illegal in all Andean countries. The lifetime prevalence of such cocaine use is similar to that reported in North America.
A detailed view of stimulant epidemiology in the United States comes from two annual national surveys conducted by the Substance Abuse and Mental Health Services Administration. The National Survey on Drug Use and Health (NSDUH) surveys a representative sample of household residents 12 years and older that is sufficiently large (about 67,500 annually) to generate valid population estimates of drug use. NSDUH data may tend to underestimate overall drug use because the survey sample does not include some groups in which drug use is likely to be higher, such as persons in correctional settings, homeless persons, hospital patients, and residential college students. The NSDUH includes nonmedical use of prescription drugs but does not measure use of OTC medications or drugs considered dietary supplements, such as ephedra or caffeine (see Chapter 2). Information on adverse consequences of stimulant use comes from the Drug Abuse Warning Network (DAWN). The DAWN is a nationwide survey of 233 general, nonfederal, short-stay hospitals with 24-hour emergency departments, selected to be representative of all US hospitals. Data are collected on patient visits associated with the use of illegal drugs or nonmedical use of prescription and OTC medications. According to NSDUH data, cocaine is the second most widely used illegal drug in the United States, after marijuana. Cocaine use in the United States peaked during the mid-1980s. The 2011 NSDUH estimated that 36.9 million Americans (14.3% of the US population 12 years old or older) had used cocaine at some time during their lifetimes (8.2 million [22.2%] by smoking “crack” cocaine); 3.9 million (1.5%) had used cocaine (0.63 million [16.3%] by smoking) within the preceding year; and 1.4 million (0.5%) had used cocaine (0.23 million [16.7%] by smoking) within the preceding month, considered current use (79). There were an estimated 670,000 new cocaine users in 2011 (76,000 [11,3%] by smoking), with a mean age of 20.1 years. An estimated 821,000 Americans met the psychiatric diagnostic criteria (DSM-IV) (80) for cocaine abuse or dependence in 2011; an estimated 511,000 people received specialty treatment for their cocaine use.
Cocaine use occurs in all segments of American society but is substantially more prevalent in certain population groups. Data from the 2001–2003 National Comorbidity Survey Replication (a representative, community-based sample of 5,692 adults) suggest that the most likely cocaine user is an unemployed, divorced/separated, non-Hispanic white male high school dropout or graduate in his 20s, living in a nonrural area in the Northeast or West, for whom religion is not important (81). These associations between cocaine use and age, gender, education level, and employment and marital status are generally found throughout the world (82).
Cocaine use is highly associated with legal substance use and with psychiatric syndromes. Cigarette smokers or heavy alcohol drinkers are each at least 10 times more likely to use cocaine than are nonsmokers or moderate (non-binge) drinkers. Current cocaine users are twice as likely to have symptoms of depressive or anxiety disorders than are nonusers (83).
The 2011 NSDUH estimated that 20.4 million Americans (7.9% of the US population aged 12 years or older) were nonmedical users of stimulants other than cocaine at some time during their lifetimes; 2.7 million (1.0%) had used such stimulants within the preceding year; and 0.97 million (0.4%) were current (past month) users. There were 845,000 new stimulant users in 2006, with a mean age of 23 years. An estimated 390,000 (0.2%) Americans met psychiatric diagnostic criteria (DSM-IV) for noncocaine stimulant abuse or dependence in 2006.
The most commonly misused synthetic stimulant is methamphetamine. The 2011 NSDUH estimated that 439,000 Americans (0.2% of population) were current users (79). Methamphetamine use is more prevalent in the western two-thirds of the United States (84).
Stimulant use often is associated with adverse consequences. In the 2011 DAWN survey, cocaine was the drug associated most often with visits to hospital emergency departments, with an estimated half-million visits (40.3% of all illicit drug–related visits), a rate of 162 cocaine-associated visits per 100,000 population (85). Amphetamines were associated with 160 thousand visits (12.8%), a rate of 51.3 visits per 100,000 population. There were 15,514 stimulant-related deaths among 15- to 64-year-olds in the United States from 1999 through 2009 (based on death certificate data collected by the Centers for Disease Control and Prevention), a rate of 0.97/100,000 person-years in 2009 (86). The highest death rates were among American Indians or Alaska Natives and those living in the West. Only a minority of stimulant users appear to seek drug abuse treatment. In 2010, there were 148,000 admissions (8.1% of all admissions) of individuals reporting cocaine as their primary drug of abuse to publicly funded and/or licensed substance abuse treatment programs, a rate of 56 admissions per 100,000 population and a 38% decrease over the preceding decade (87). More than two-thirds (70.6%) of these admissions involved smoked cocaine. Another 112,000 admissions (6.2%) were for primary amphetamine/methamphetamine use, a rate of 43 per 100,000 and a 40% increase over the preceding decade.
Absorption and Distribution
Route of administration has a major effect on the pharma-cokinetic characteristics of stimulants (88). Smoked stimulants (such as cocaine base or methamphetamine) are rapidly absorbed through the lungs and probably reach the brain in 6 to 8 seconds. Thus, the onset and peak effect occur within minutes of administration. As the stimulant redistributes from the brain, there is a rapid decline in effect. Intravenous administration produces peak brain uptake in 4 to 7 minutes, based on positron emission tomography (PET) studies with radiolabeled cocaine (89). Greatest cocaine uptake occurs in the striatum (caudate, putamen, and nucleus accumbens) and least uptake in the orbital cortex and cerebellum. Clearance to half-peak brain levels requires 17 to 30 minutes and is fastest in the orbital cortex, thalamus, and cerebellum and slowest in the striatum. The rapid offset after rapid onset often is experienced as a “crash” by users of smoked or intravenous stimulants. Heavy cocaine users have about 20% less brain cocaine uptake than do healthy nonusers (90). The mechanism of this difference is not known but could be related to differences in plasma protein binding or permeability of the blood–brain barrier.
Intranasal and oral stimulants have a slower absorption and onset of effect (30 to 45 minutes), a longer peak effect, and a more gradual decline from peak. The peak intensity of effect is weaker than with smoked or intravenous administration because less active drug reaches its site of action in the brain. Coca leaf chewing produces less than half the peak cocaine plasma concentrations of an equivalent dose of intranasal cocaine (91). However, even a single oral dose of cocaine (such as 2 mg in a cup of coca tea) may yield detectable urine concentrations of cocaine metabolites (92,93). Pharmacokinetic parameters for oral stimulants are given in Table 10-3.
TABLE 10-3 PHARMACOKINETIC PARAMETERS OF ORAL STIMULANTS
a Urine pH dependent: lower pH yields a shorter half-life.
Tmax, time of maximum plasma concentration (in hours); T1/2, half-life (in hours); Vd, apparent volume of distribution (in liters per kg of body weight); pKa, acid dissociation constant (= pH at which drug is 50% ionized); Fb, fraction of bound drug.
From Baselt RC. Disposition of toxic drugs and chemicals in man, 7th ed. Foster City, CA: Chemical Toxicology Institute, 2004.
Cocaine is well absorbed through mucous membranes and also is absorbed through intact skin or by passive inhalation of smoked cocaine or aerosolized particles (94–96). Passive exposure can result in adverse medical effects in infants (96,97) and detectable urine concentrations of cocaine metabolites in medical and laboratory personnel (95,98). In many cases, these concentrations are too low to trigger a positive result on routine urine drug testing.
Stimulants distribute into most tissues of the body. Cocaine is rapidly taken up into the heart, kidney, adrenal glands, and liver (2). In addition to blood and urine (99), cocaine and its hydrolytic metabolites, amphetamines, phentermine, and ephedrine and its analogs appear in hair (100–102), sweat (103), oral fluid (saliva) (104–106), nails (107), and breast milk (108) and cross the placenta to appear in umbilical cord blood, amniotic fluid, and meconium (107). Analysis of these tissues and fluids is used for drug detection in workplace, legal, and treatment settings (109). Stimulants can also be detected postmortem in body fluids and tissues (110).
Metabolism
In humans (and other primates), 95% of cocaine is metabolized by hydrolysis of ester bonds to benzoylecgonine (the primary urinary metabolite) and ecgonine methyl ester by the action of carboxylesterases in the liver and butyryl-cholinesterase in the liver, plasma, brain, lung, and other tissues (91,111–113). The remaining 5% of cocaine is N-demethylated to norcocaine by the CYP3A4 isozyme of the liver cytochrome P450 microsomal enzyme system. This is the predominant metabolic pathway in rodents.
Norcocaine has some pharmacologic actions similar to those of cocaine and is hepatotoxic. This may account for the significant hepatotoxicity of cocaine in rodents, which is not found in primates. Cocaine’s hydrolytic metabolites appear to be much less active pharmacologically, though this has not been well studied (114,115).
Amphetamines are metabolized in the liver via three different pathways: deamination to inactive metabolites, oxidation to norephedrine and other active metabolites, and parahydroxylation to active metabolites (116). Amphetamine itself is the initial metabolite of methamphetamine.
When cocaine is smoked, a pyrolysis product is formed (methylecgonidine or anhydroecgonine methyl ester), the presence of which allows identification of the smoked route of administration (88,91,113).
Elimination
Stimulants and their metabolites are largely eliminated in the urine (22,91). Benzoylecgonine is the cocaine metabolite found in highest concentration in urine for several days after cocaine use. It is this substance, rather than the parent drug cocaine, that actually is measured in routine urine drug tests for cocaine. GI absorption and urinary elimination of amphetamines are highly pH dependent (37). Because amphetamines are weak bases (pKa around 9.9) (116), acidification of the GI tract or urine substantially decreases absorption and increases excretion, respectively (91). Conversely, alkalinization of the GI tract or urine can increase GI absorption and reduce excretion to negligible levels. This fact is exploited by drug users who take large doses of sodium bicarbonate to prolong the action of amphetamines and reduce the amount present in the urine for detection by drug tests (117).
The primary drug interaction of stimulants that is of clinical concern is with other stimulants or with other medications that also enhance catecholamine activity (38). Such interactions risk overstimulation of the sympathetic nervous system, with possible cardiac arrhythmia, hypertension, seizure, cardiovascular collapse, and death. The major potential for interaction is presented by monoamine oxidase inhibitors (MAOIs), which are used as antidepressants. MAOIs enhance catecholamine activity by inhibiting a major metabolic pathway for catecholamines. Potent prescription stimulants, such as amphetamine and methamphetamine, should not be used within 2 weeks of MAOI use. Stimulants should be used cautiously in conjunction with tricyclic antidepressants, many of which block presynaptic reuptake of catecholamines.
When cocaine is used in combination with alcohol, a new compound, cocaethylene, is formed by transesterification (118). Cocaethylene has pharmacologic actions similar to, but less potent than, those of cocaine, with a longer half-life. Formation of cocaethylene may contribute to more severe or longer-lasting toxic effects of cocaine when it is used along with alcohol. However, human laboratory studies of the cocaine/alcohol/cocaethylene interaction have given inconsistent results (119,120).
Central Nervous System
Intoxication
All stimulants produce a similar range of psychological, behavioral, and physiologic effects, with the intensity and duration depending on potency, dose, route of administration, and duration of use (see Section 6, Chapter 46, “Management of Stimulant, Hallucinogen, Marijuana, Phencyclidine, and Club Drug Intoxication and Withdrawal”). The initial effects—usually desired— include increased energy, alertness, and sociability; elation or euphoria; and decreased fatigue, need for sleep, and appetite (121). The intense pleasurable feeling has been described as a “total body orgasm” (122). These effects may occur after 5 to 20 mg of oral amphetamine, methamphetamine, or methylphenidate; 100 to 200 mg of oral cocaine; 40 to 100 mg of intranasal cocaine; or 15 to 25 mg of IV or smoked cocaine (122,123). Such single oral doses of stimulants improve cognitive and psychomotor performance in subjects whose performance has been impaired by fatigue, sleep deprivation, or alcohol, especially in tasks that require focused and sustained attention (vigilance) (123,124).
There is less consistent evidence that stimulants are of any benefit in subjects who are fully alert and attentive or engaged in tasks involving learning, memory, or problem solving. With increasing potency, dose, duration of use, or a more efficient route of administration, stimulant effects often progress to include dysphoric effects such as anxiety, irritability, panic attacks, interpersonal sensitivity, hyper-vigilance, suspiciousness, paranoia, grandiosity, impaired judgment, and psychotic symptoms such as delusions and hallucinations. Among nontreatment-seeking users, 10% to 40% may have sleep disturbance and weight loss (due to appetite suppression), and up to 25% may experience severe paranoia and/or hallucinations (125,126). The proportion of stimulant users experiencing paranoia or psychotic symptoms varies with intensity and duration of use and degree of dependence and has been as high as 100% in some studies, even among community-based and non–treatment-seeking individuals (127–129).
Patients with stimulant psychosis may closely resemble those with acute schizophrenia (130,131)—perhaps not surprising, given that both conditions share the presumed pathophysiology of excessive brain dopamine activity (132). Cocaine-induced psychosis may differ from acute schizophrenic psychosis in being marked by less thought disorder and bizarre delusions and fewer negative symptoms such as alogia and inattention (129). Stimulant-induced hallucinations may be auditory, visual, or somatosensory (127,133). Tactile hallucinations are especially typical of stimulant psychosis and include the sensation of something (e.g., insects) crawling under the skin (“formication,” “cocaine bugs”).
Parallel behavioral effects include restlessness, agitation, tremor, dyskinesia, and repetitive or stereotyped behaviors such as picking at the skin or foraging for drug (“punding,” “hung-up activity”) (69,134). Associated physiologic effects include tachycardia, pupil dilation, diaphoresis, and nausea, reflecting stimulation of the sympathetic nervous system. Criteria for the psychiatric diagnosis of stimulant intoxication are given in (80). Treatment of acute stimulant intoxication is reviewed in Section 6, Chapter 46, “Management of Stimulant, Hallucinogen, Marijuana, Phencyclidine, and Club Drug Intoxication and Withdrawal.” Cocaine and the more potent synthetic stimulants (such as amphetamines) produce these adverse effects at readily available doses by any route of administration. Less potent oral stimulants may require chronic, high-dose use or diversion to intravenous use to cause these effects. Even OTC stimulants have been associated with severe psychological effects, abuse, and dependence.
There is wide individual variability in the response to stimulants. The reasons are poorly understood but presumably are related to differences in genetics, psychological characteristics (including personality traits), previous drug experience, the setting in which the drug is taken, and the existence of psychiatric or medical comorbidities (122,135,136). Identical twins are highly concordant in their response to single doses of stimulants, suggesting an important genetic component. In animals, response to stimulants appears to be under polygenic control, with independent genetic influences on responses to cocaine or amphetamine (137). In rats, behavioral response to cocaine shows a negative correlation with baseline brain dopamine concentrations and a positive correlation with the increase in dopamine concentration elicited by a cocaine challenge (138).
Individual differences in tolerance and sensitization to stimulants (see later discussion: Neuroadaptation) may account for the poor correlation between stimulant plasma concentrations and toxic effects (122,139,140). Fatal cases of amphetamine or cocaine intoxication may present with 100-fold differences in plasma stimulant concentration (6).
Chronic Effects
Chronic cocaine or amphetamine abuse is associated with cognitive impairment that may persist for at least several months of abstinence (141,142). Most affected are visuomotor performance, attention, inhibitory control, and verbal memory. Several studies have found abnormalities of behavioral regulation and risk–reward decision making. This type of impairment is associated with lesions of the frontal cortex, a brain area that shows decreased regional blood flow and metabolic activity in abstinent cocaine abusers.
Chronic cocaine use is associated with decreased gray and white matter volumes in the frontal cortex of the brain (143), enlarged basal ganglia, and lower concentrations of N-acetylaspartate (a magnetic resonance spectroscopy marker for normal neuronal function) and higher concentrations of creatine and myoinositol (markers of glial cell activity and inflammation) (144).
Chronic amphetamine or methamphetamine use (either oral or intravenous) can cause a psychotic syndrome (with paranoia and hallucinations) that may persist for years after the last drug use, even in persons with no personal or family history of psychiatric disorder (145,146). Methamphetamine psychosis may be associated with focal perfusion deficits in the frontal, parietal, and temporal lobes of the cerebral cortex (147). Psychotic flashbacks have been reported in methamphetamine abusers up to 2 years after their last drug use and often are precipitated by threatening experiences (146). A persisting psychosis after cocaine use has not been reported, except in patients with an underlying psychiatric disorder (such as schizophrenia or bipolar disorder) (148).
Withdrawal
Cessation of stimulant use may result in a withdrawal syndrome that does not have prominent physiologic features and is not life threatening (149–151). Withdrawal symptoms generally are the opposite of those associated with stimulant intoxication and include depressed mood, anhedonia (inability to experience pleasure), fatigue, difficulty concentrating, increased total sleep and rapid eye movement sleep duration (but with poor sleep quality) (152), and increased appetite. Criteria for the psychiatric diagnosis of stimulant withdrawal are given in (80). Treatment of stimulant withdrawal is reviewed in Section 6, Chapter 46, “Management of Stimulant, Hallucinogen, Marijuana, Phencyclidine, and Club Drug Intoxication and Withdrawal.”
An early report of cocaine withdrawal among 30 outpatients described a complex triphasic syndrome lasting several months. This finding has not been replicated in subsequent inpatient or outpatient studies (149,151,153). Several studies of inpatients undergoing cocaine withdrawal found a monotonic decline in symptoms over 1 to 2 weeks.
Rodents undergoing cocaine or amphetamine withdrawal after a period of chronic drug exposure tend to show biphasic changes in the brain: initial increases in extracellular dopamine concentrations and expression of dopamine transporter and kappa and mu opiate receptors, followed by decreases in these variables (154,155). The few human studies on cocaine withdrawal do not find consistent changes in peripheral markers of dopamine activity, as in plasma concentrations of dopamine metabolites or of prolactin (a hormone under dopamine control) (156,157).
Human brain imaging studies suggest a modest increase in dopamine transporter binding during early cocaine withdrawal (using single-photon emission computed tomography [SPECT]) (158), followed by a decrease after 11 to 30 days of abstinence (using PET) (157). This pattern is consistent with most, but not all, postmortem studies of cocaine users (159).
Behavioral Pharmacology
Cocaine, amphetamines, cathinone, ephedrine, and most other stimulants that have been tested in animals consistently produce increased motor activity, repetitive stereotyped behavior, drug discrimination, and evidence of reinforcing effects (such as drug self-administration and conditioned place preference) (160–162). Animals allowed free access to stimulants often self-administer in a “binge-abstinence” pattern: periods of high levels of drug intake (producing stereotyped behavior, hyperactivity, decreased eating, and little sleep), alternating with periods of abstinence, during which behavior returns to normal (138). Animals given unlimited access to stimulants may self-administer to the point of death during a binge period. The rewarding effect of stimulants in animals is influenced by the same factors as are other drug and natural reinforcers, for example, the dose of drug available, the schedule of reinforcement, the animal’s past history of development and drug exposure, and the current environment and condition of the animal. Stimulant self-administration is reduced by increased work requirements, availability of an alternative potent reinforcer, or the concurrent presence of punishment (as by electric shock) and increased by food deprivation or stress (136,163).
Animals undergoing enforced abstinence after a period of stimulant self-administration initially increase their responding in an apparent attempt to obtain drug but eventually extinguish their drug-seeking behavior (138). However, they will promptly resume drug-seeking behavior if given a single “priming” dose of the drug or exposed to drug-associated stimuli or stress (such as electric foot shock) (164). This reinstatement of drug-seeking behavior has been considered an animal model of relapse to drug use after treatment.
Stimulants produce a distinctive set of subjective psychological effects (including euphoria, drug liking, increased energy, and increased alertness) in humans under controlled double-blind experimental conditions (161,165). d-Amphetamine, benzphetamine, cocaine, ephedrine, mazindol, methylphenidate, phenmetrazine, and phenyl-propanolamine are readily distinguished from placebo or sedative drugs but often are not distinguished from each other when equipotent doses are given.
Other Central Nervous System Effects
Acute stimulant administration is associated with transient increases in electroencephalographic (EEG) alpha, beta, and theta activity, which correlate with the acute psychological effects (166). Stimulant use by any route of administration is associated with seizures, even in persons without a preexisting seizure disorder (167–169). These can occur with the first use and most often are single, generalized tonic–clonic seizures, although multiple seizures and status epilepticus can occur. Most cocaine-associated seizures occur within 90 minutes of drug use, during the time of peak plasma concentration.
Cocaine and amphetamine use are associated with cerebral vasoconstriction, cerebrovascular atherosclerosis, cere-brovascular disease, and stroke (170–172). In a study of more than 800,000 discharges from Texas hospitals in 2003, cocaine and amphetamine users had more than twice the risk of stroke than do patients not using stimulants (173). Hemorrhagic stroke appears more common in intranasal or intravenous users, whereas ischemic stroke is more common in smokers. Neurologic symptoms usually appear within 3 hours of drug use, though a minority of patients may be asymptomatic for up to 24 hours. An underlying cerebrovascular abnormality (such as arterial aneurysm or arteriovenous malformation) probably increases the risk of cocaine-associated stroke, but the majority of such stroke patients do not have any cerebrovascular risk factors. The OTC stimulant phenylpropanolamine, marketed as a decongestant and appetite suppressant, was associated with a significant risk of hemorrhagic stroke in a recent case–control study, even in patients who used it at recommended doses (174). This finding led the FDA to remove phenylpropanol-amine from the US market in October 2000.
Stimulant use is associated with a variety of movement disorders, presumably as the result of increased dopamine activity in the basal ganglia and other brain areas that control movement (122,167,175). Such disorders include repetitive stereotyped behaviors (such as repeated dismantling of objects, cleaning, doodling, and searching for imaginary objects), acute dystonic reactions, choreoathetosis and akathisia (so-called crack dancers), buccolingual dyskinesias (“twisted mouth” or “boca torcida”), and exacerbation of Tourette syndrome and tardive dyskinesia. Cocaine users are at increased risk of extrapyramidal symptoms (but not akathisia) when taking antipsychotic medications (176).
Cardiovascular System
Stimulants act acutely on the cardiovascular system both directly (by increasing adrenergic activity at sympathetic nerve terminals) and via the CNS to increase heart rate, blood pressure, and systemic vascular resistance (170,177). Stimulant-induced increases in heart rate and blood pressure are significantly correlated with increases in plasma norepinephrine and epinephrine concentrations (178,179), suggesting mediation by increased activity of the sympathetic nervous system. The mechanism may be prolonged blockade of norepinephrine transporters in the heart, amplifying the action of endogenous norepinephrine.
Cocaine-induced tachycardia is blocked by beta-adrenergic receptor blockade (propranolol) but not by muscarinic receptor blockade (atropine) (180), further suggesting a sympathetic role. The resulting increase in myocardial oxygen demand, often accompanied by decreased coronary blood flow (from vasospasm and vasoconstriction), may cause acute myocardial infarction, even in young persons without atherosclerosis. This process may be promoted by cocaine-induced increases in circulating activated platelets, platelet aggregation, and thromboxane synthesis. Cocaine use is a factor in about one-fourth of nonfatal heart attacks in persons younger than 45 years (181). Frequent cocaine users are up to seven times more likely to have a nonfatal heart attack than are nonusers (181). Postmortem studies suggest that chronic cocaine use is associated with higher levels of coronary atherosclerosis (171).
Cocaine use is associated with cardiac arrhythmias (such as ventricular tachycardia or fibrillation) and sudden death (177,182). The mechanisms include blockade of myocyte sodium channels (resulting in impaired cardiac conduction and areas of localized conduction block) and increased concentration of plasma norepinephrine (which sensitizes the myocardium).
Chronic cocaine or amphetamine use is associated with cardiomyopathy and myocarditis (177,183,184). Case series of asymptomatic cocaine abusers have found up to half with echocardiographic abnormalities such as left ventricular hypertrophy and abnormal segmental wall motion. Cocaine-associated cases of dilated cardiomyopathy and myocardial fibrosis may be due to direct toxic effects of high concentrations of circulating norepinephrine. Cocaine-associated myocarditis (whose acute symptoms may mimic myocardial infarction) may be a direct toxic effect of cocaine or a hypersensitivity effect. Autopsy series of current cocaine users have found myocarditis in up to 20%.
Other Organ Systems
No large surveys or prospective studies have comprehensively evaluated the natural history of stimulant use or the frequency of adverse effects. Existing knowledge derives largely from case reports or case series of persons who come to medical attention (185). In the absence of experimental data, it may be difficult to determine the extent to which an observed adverse effect is the result of a direct action on the affected organ or tissue, an indirect action, an effect of a street drug contaminant, or secondary to other factors that are part of a drug-using lifestyle, such as needle sharing, malnutrition, use of other substances, and the like. The most relevant indirect action of cocaine in producing adverse effects in many organs and tissues is ischemia and infarction. These result from several mechanisms, including vasoconstriction, vasospasm, damage to endothelium, and increased clotting as the result of increased number of circulating activated platelets, enhanced platelet aggregation, and increased thromboxane synthesis (167). These mechanisms often reinforce one another: for example, vasospasm may damage endothelium, endothelial damage increases thromboxane synthesis, and thromboxane causes platelet aggregation and vasoconstriction.
Adverse effects of stimulant use on particular organ systems often depend on the route of administration. For example, smoked stimulants produce lung toxicity not found with other routes, injection use is associated with infectious diseases such as human immunodeficiency virus and hepatitis C, and intranasal use is associated with damage to the nasal septum.
Pulmonary
Smoked cocaine produces both acute and chronic pulmonary toxicity (186–189). Acute respiratory symptoms may develop in up to half of users within minutes to several hours after smoking. Symptoms include productive cough, shortness of breath, wheezing, chest pain, hemoptysis, and exacerbation of asthma. More severe, and rarer, acute effects include pulmonary edema, pulmonary hemorrhage, pneumothorax, pneumomediastinum, and thermal airway injury. Pulmonary edema also has been reported after intravenous cocaine use. Chronic cocaine smoking has been associated in case reports with pulmonary and peripheral eosinophilia, interstitial pneumonitis, and bronchiolitis obliterans. The pathophysiology of these adverse effects is not definitively understood but presumably involves a combination of direct damage by cocaine or inhaled microparticles to the alveolar–capillary membrane, vasoconstriction and damage to the pulmonary vascular bed, and/or interstitial disease.
The long-term effect of cocaine smoking on pulmonary function remains unclear (186). Standard pulmonary function tests (spirometry) have been normal in most studies. Some studies have found increased alveolar epithelial permeability and moderately decreased (up to 20%) pulmonary diffusion capacity among cocaine smokers without acute symptoms, although other studies have found normal function. The attribution of these abnormalities to cocaine use is confounded by the fact that the vast majority of cocaine-smoking subjects also were smokers of tobacco and/or marijuana.
Renal
Stimulants have little direct toxic effect on the kidneys. Acute renal failure can occur as a result of renal ischemia or infarction, malignant hypertension, or rhabdomyolysis (see later discussion: Musculoskeletal) (190–192). Release of myoglobin during rhabdomyolysis may cause renal tubular obstruction or direct myoglobin damage to renal tubules. Intrarenal arterial constriction with resulting renal medullary ischemia also may contribute to renal tubular damage.
Gastrointestinal
Cocaine reduces gastric motility and delays gastric emptying, in part by affecting medullary centers that regulate these functions (167). The major gastrointestinal effects of cocaine use are due to vasoconstriction and ischemia: gastroduodenal ulceration and perforation, intestinal infarction and perforation, and ischemic colitis (167,185,193). The distribution of cocaine-associated ulcers is primarily in the greater curvature and prepyloric region of the stomach, pyloric canal, and first portion of the duodenum, whereas peptic ulcers occur primarily in the duodenal bulb. Concealing cocaine by swallowing large packets (“body packing”) may result in severe acute toxicity if the wrapping deteriorates and allows cocaine into the gastrointestinal tract (175).
Liver
Cocaine is hepatotoxic in rodents, presumably because of oxidative metabolism to norcocaine by the cytochrome P450 microsomal enzyme system in the liver, with further transformation to reactive hepatotoxic compounds such as N-hydroxynorcocaine (175). This is a very minor metabolic pathway in humans (see previous discussion: Metabolism). There is no direct evidence that cocaine is hepatotoxic in humans. Liver abnormalities reported in case series of cocaine users can be accounted for by viral hepatitis from injection drug use, alcoholic liver disease, or other consequences of a drug-using lifestyle.
Pemoline has been associated with hepatocellular toxicity, sometimes resulting in fulminant liver failure and death (6,194). This led to pemoline’s withdrawal from the US market in 2005.
Endocrine
Acute cocaine use activates the hypothalamic–pituitary–adrenal (HPA) axis, stimulating secretion of epinephrine, corticotropin-releasing hormone, adrenocorticotrophic hormone (ACTH), and cortisol (175,195,196). Acute cocaine use decreases plasma prolactin concentrations in cocainenaïve individuals, presumably because of increased dopamine activity (dopamine inhibits prolactin release from the pituitary). Chronic cocaine users may have increased, normal, or decreased prolactin levels and usually do not show changes in response to acute cocaine. Acute cocaine use increases plasma luteinizing hormone, but chronic cocaine users have normal levels of testosterone, cortisol, luteinizing hormone, and thyroid hormones. A blunted thyroid-stimulating hormone response to thyroid-releasing hormone stimulation has been reported in one study but has not been replicated. Cocaine use is associated with increased risk of diabetic ketoacidosis, because of either poor treatment adherence or acute stimulation of the HPA (197).
Musculoskeletal
Stimulants may cause rhabdomyolysis by several different mechanisms: a direct toxic effect causing myofibrillar degeneration (probably rare except at very high doses), indirectly by vasoconstriction of intramuscular arteries resulting in ischemia, and secondary to stimulant-induced hyperthermia or seizures (167,198). Up to one-third of patients with rhabdomyolysis will develop acute renal failure, sometimes accompanied by disseminated intravascular coagulation and liver damage. This syndrome often is fatal.
Head and Neck
Common head and neck complications of cocaine use depend on the route of administration (167,175). Intranasal cocaine use (“snorting”) is associated with chronic rhinitis, perforated nasal septum and nasal collapse, oropharyngeal ulcers, and osteolytic sinusitis, presumably due to vasocon-striction and resulting ischemic necrosis (199). Changes in the sense of smell are rare, even in heavy users with intranasal damage (200). Oral cocaine use is associated with gingival ulceration and erosion of dental enamel (175). In South America, chronic coca leaf chewing has been associated with mild epithelial changes but no evidence of premalignant or malignant lesions (201). The observed changes may result from the alkaline ash usually chewed with the leaves to enhance cocaine extraction rather than from the cocaine itself. Smoked cocaine (“crack”) may cause corneal ulcers (202). Use of cocaine or methamphetamine by any route of administration reduces salivary secretions (xerostomia) and causes bruxism (203,204). Chronic use is associated with several dental problems, including caries, cracking of tooth enamel, and loss of teeth (“meth mouth”). Because oral health problems are pervasive among stimulant users, dental professionals serve an important role in identifying these users and getting them into treatment.
Immune System
Cocaine use has been associated with a variety of vasculitic syndromes primarily affecting skin and muscle (205). These may mimic rheumatologic conditions such as Henoch-Schönlein purpura, Stevens-Johnson syndrome, or Raynaud phenomenon. Cocaine-associated midline destructive lesions may resemble Wegener granulomatosis (206). In experimental studies, cocaine impairs innate immune mechanisms (e.g., the response of monocytes to bacterial lipopolysaccharide) (207).
Sexual Function
Stimulants are commonly thought of as an aphrodisiac, but chronic use usually reduces libido and impairs sexual function (208–210). Men may experience erectile dysfunction or delayed or inhibited ejaculation. Priapism is rare. Women may develop irregular menses.
Cocaine has been applied to the penis or clitoris to use its local anesthetic effect to delay orgasm (209).
Reproductive, Fetal, and Neonatal Health
Prescription stimulants, including cocaine and amphetamines, are classified by the FDA in pregnancy category C, meaning that risk cannot be ruled out because human studies are lacking. One exception is diethylpropion, which is category B (no evidence of risk in humans). Male chronic cocaine users have reduced sperm count and motility (210).
Prenatal (in utero) exposure to cocaine, amphetamines, or methylphenidate has been associated with vaginal bleeding, abruptio placenta, placenta previa, premature rupture of membranes, decreased head circumference, low birth weight, tremulousness, irritability, poor feeding, and autonomic instability (211–213). It is usually difficult in these studies to distinguish a direct effect of the stimulant from the effects of concomitant factors frequently present in drug users, such as other drug use (including alcohol, nicotine, and opiates), poor nutrition, and lack of prenatal care. Long-term studies suggest that prenatal cocaine exposure is associated with small but statistically significant impairments in sustained attention and behavioral self-regulation among preschool- and school-aged children (214) and in language and memory among adolescents (215). These effects appear modifiable by environmental factors such as prenatal care and the care-giving environment. Their causal mechanisms remain unknown. Cocaine, amphetamines, phentermine, ephedrine, and pseudoephedrine appear in breast milk (108). Cocaine and amphetamines may cause irritability, sleep disturbance, and tremors in the infant. Medical use by the mother of other prescription and OTC stimulants in appropriate doses usually does not have clinically significant adverse effects on nursing infants
Mechanisms of Action
Molecular mechanisms
All stimulant drugs act to enhance extracellular concentrations of monoamine neurotransmitters (dopamine, nor-epinephrine, and serotonin) in the central and peripheral nervous systems. Stimulants achieve their effects by disrupting the function of plasma membrane transporter proteins expressed on neurons that synthesize and release these neurotransmitters (216) (Fig. 10-3). Under normal circumstances, monoamine transporters (MATs) (or uptake pumps) mediate the uptake (or reuptake) of previously released neurotransmitter molecules from the extracellular space back into nerve cells (i.e., transmitter uptake), thus terminating neurotransmitter action. MATs belong to a gene family of neurotransmitter–sodium transporters, which depend upon intact sodium gradients to facilitate neurotransmitter uptake. Because transporters are not confined to nerve endings (i.e., synapses), being also found on cell bodies, dendrites and axons, they are critically involved with controlling extrasynaptic volume transmission of monoamines (217).
FIGURE 10-3 Mechanism of action of stimulants acting as monoamine transporter blockers (A) or substrates (B). Drugs that act as monoamine transporter (MAT) blockers increase the concentration of amine neurotransmitters (i.e., dopamine, norepinephrine, and serotonin) in the extracellular fluid (ECF) by blocking reuptake of previously released neurotransmitter molecules. Drugs that act as MAT substrates increase concentrations of amine transmitters in the ECF by a two-pronged mechanism: (a) they promote nonexocytotic release of cytoplasmic amine transmitters by entering neurons and reversing the normal direction of transporter flux (i.e., reverse transport); and (b) they disrupt storage of transmitters in vesicles by interacting with vesicular monoamine transporters (VMAT). The disruption of transmitter storage increases cytoplasmic concentration of transmitter molecules available for transporter-mediated release.
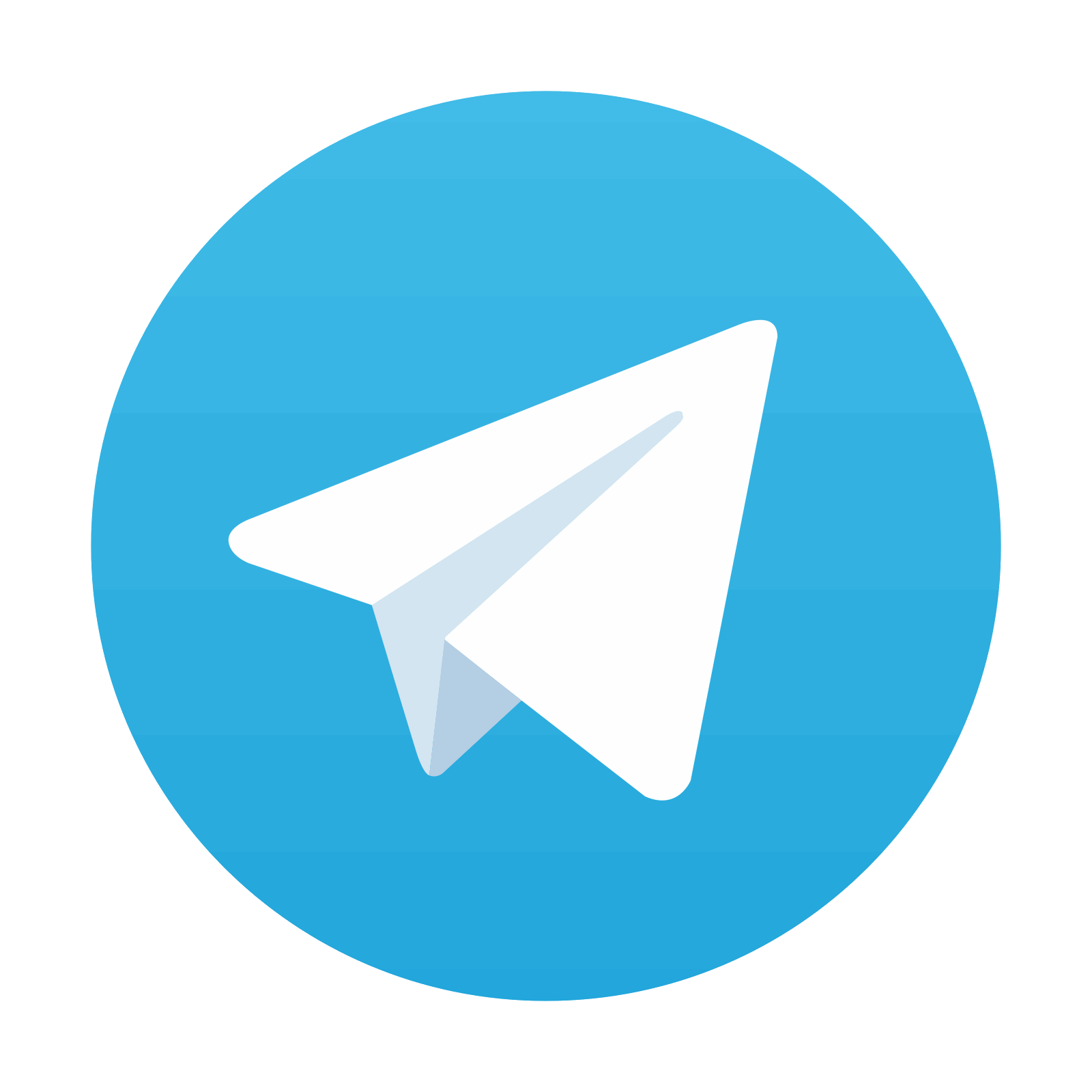
Stay updated, free articles. Join our Telegram channel

Full access? Get Clinical Tree
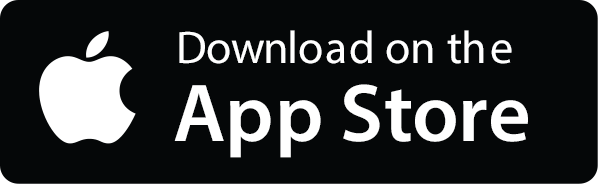
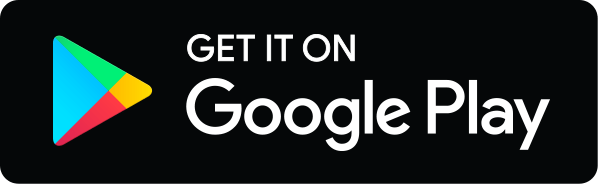